© Springer International Publishing Switzerland 2016
Debra G.B. Leonard (ed.)Molecular Pathology in Clinical Practice10.1007/978-3-319-19674-9_2626. Von Hippel-Lindau Disease
(1)
Department of Pathology and Laboratory Medicine, The Children’s Hospital of Philadelphia, ARC 714G, 34th and Civic Center Boulevard, Philadelphia, PA 19104, USA
Abstract
Von Hippel-Lindau disease (VHLD) is an autosomal dominant cancer predisposition syndrome characterized by tumors of the brain and spine, retina, kidney, adrenal glands, and several other organs. Point mutations and deletions in the VHL tumor suppressor gene have been identified in virtually 100 % of patients who meet strict clinical criteria. Molecular diagnostic testing consists of DNA sequence analysis of the coding region (~72 % of mutations) as well as deletion analysis (~28 % of mutations). Most cases are inherited; however about 20 % of cases are the result of de novo mutations; individuals mosaic for a VHL gene mutation have been reported. Molecular testing may be used to confirm a clinical diagnosis in an affected patient or rule out the disease in individuals with a single typical tumor. In families with a known mutation, molecular testing may be used for predictive testing of at-risk members or for prenatal testing.
Keywords
Von Hippel-Lindau disease VHL geneTumor suppressorDe novo mutationsMolecular testingDiagnostic testingPredictive testingPrenatal testingIntroduction
Von Hippel-Lindau disease (VHLD; OMIM#19330) is an autosomal dominant cancer predisposition syndrome that gives rise to hemangioblastomas of the brain and spine, retinal angiomas, clear cell renal cell carcinoma, pheochromocytoma, endolymphatic sac tumors, tumors of the epididymis or broad ligament, and pancreatic tumors or cysts [1]. The incidence of VHLD is approximately 1 in 36,000 live births [2]. Onset is typically between the second and fourth decades of life, with penetrance for the disease nearly complete by the age of 65 years. In most cases, a family history of the disorder is apparent. In about 20 % of cases, however, the proband appears to have acquired a new mutation [3].
Molecular Basis of Disease
The VHL tumor suppressor gene was isolated by positional cloning in 1993 [4]. The gene, which consists of three exons spanning about 10 kilobases (kb) of genomic DNA on the short arm of chromosome 3 (3p25.3), is highly conserved among worms, flies, frogs, fish, chickens, humans, and other mammals (reviewed in Refs. 5 and 6). Two transcripts, 6.0 and 6.5 kb in size, are almost ubiquitously expressed and encode proteins of 213 and 160 amino acid residues, respectively. The latter isoform is the major product in most tissues and results from initiation of translation from an internal methionine codon at position 54. Both protein isoforms appear to be functional.
The VHL protein has been implicated in a variety of functions including transcriptional regulation, posttranscriptional gene expression, apoptosis, extracellular matrix formation, and ubiquitylation (reviewed in Refs. 5 and 6). The role of VHL in the regulation of hypoxia-inducible genes through the targeted ubiquitylation and degradation of hypoxia-inducible factor 1 alpha subunit (HIF1A) has been elucidated, leading to a model of how disruption of the VHL gene results in the production of highly vascularized tumors.
Normal VHL binds to the protein elongin C, which forms a complex with elongin B, cullin-2 (CUL2), and Rbx1. This complex resembles the SKP1-CUL1-F-box protein (SCF) ubiquitin ligase or E3 complex in yeast that catalyzes the polyubiquitylation of specific proteins and targets them for degradation by proteosomes. Under normoxic conditions, HIF1A is hydroxylated at a specific asparagine residue by a member of the egg-laying deficiency protein nine-like (EGLN) protein family of prolyl hydroxylase enzymes. VHL binds to hydroxylated HIF1A and targets it for degradation. Under hypoxic conditions, HIF1A is not hydroxylated, VHL does not bind, and HIF1A subunits accumulate. HIF1A forms heterodimers with HIF1B and activates transcription of a variety of hypoxia-inducible messenger RNAs (i.e., VEGF, EPO, TGFA, PDGFB). Likewise, when VHL is absent or mutated, HIF1A subunits accumulate, resulting in cell proliferation and the neovascularization of tumors characteristic of VHLD [5].
Predisposition to VHLD is inherited in an autosomal dominant manner. However, tumor formation requires inactivation of the second allele (i.e., through loss of heterozygosity, methylation, or point mutation), and so the disease is recessive at the level of the cell. Mutations known to result in predisposition to VHLD include partial or complete deletions of the gene and point mutations (missense, nonsense, frameshift, and splice site). Point mutations are predicted either to truncate the protein, alter protein folding, or interfere with the binding of VHL to elongin C, HIF1A, or other target proteins [5, 7]. Although a handful of mutations and one mutation “hotspot” in exon 3 are common, point mutations are distributed over all three exons of the gene from codon 54 (internal initiator methionine) to the stop codon.
Four VHLD phenotypes have been described based on the likelihood of pheochromocytoma or renal cell carcinoma [8]. Type 1 is characterized by a low risk for pheochromocytoma. Truncating mutations or missense mutations that are predicted to grossly disrupt the folding of the VHL protein [7] are associated with VHLD type 1. VHLD type 2 is characterized by a high risk for pheochromocytoma. Most patients with VHLD type 2 have missense mutations of VHL. VHLD type 2 is further subdivided into those with a low risk (type 2A) and those with a high risk (type 2B) of renal cell carcinoma, as well as individuals at risk for pheochromocytoma only (type 2C). Some missense mutations correlate with a specific type 2 VHLD phenotype [6, 8].
A novel genotype-phenotype correlation has been reported, but is not yet considered to be a separate VHL type [9]. Individuals with a complete deletion of the VHL gene are more likely to present with multiple hemangioblastomas of the brain or spine or both as the first or only symptom of disease. Pheochromocytoma and renal cell carcinoma are less likely or may present at a later stage in life. At present, it is not clear why a complete deletion of the VHL gene would result in a phenotype distinctly different from that caused by a partial deletion or truncating mutation (i.e., VHL type 1). However, deletion of a neighboring gene(s) at the same locus may contribute to this phenotype [6].
Clinical Utility
Clinical molecular testing for VHLD has proven to be virtually 100 % effective at detecting germline mutations in patients with pathology-proven disease [10]. For this reason, molecular testing may be used to confirm a clinical diagnosis in an affected patient, screen for a mutation in an unaffected individual with a family history of VHLD, or rule out the disease in individuals with one VHLD-like tumor but no family history. When the mutation in the family is known, molecular testing may be used for diagnostic or presymptomatic testing of at-risk family members or for prenatal testing.
A clinical diagnosis of VHLD may be made in an individual with at least two typical VHLD tumors with or without a family history of VHLD or in an individual with at least one typical tumor and a significant family history [1]. In such cases, a VHL gene mutation is almost invariably found. Identification of a VHL mutation confirms the clinical diagnosis, establishes the need for periodic clinical screening, and facilitates presymptomatic testing of at-risk relatives.
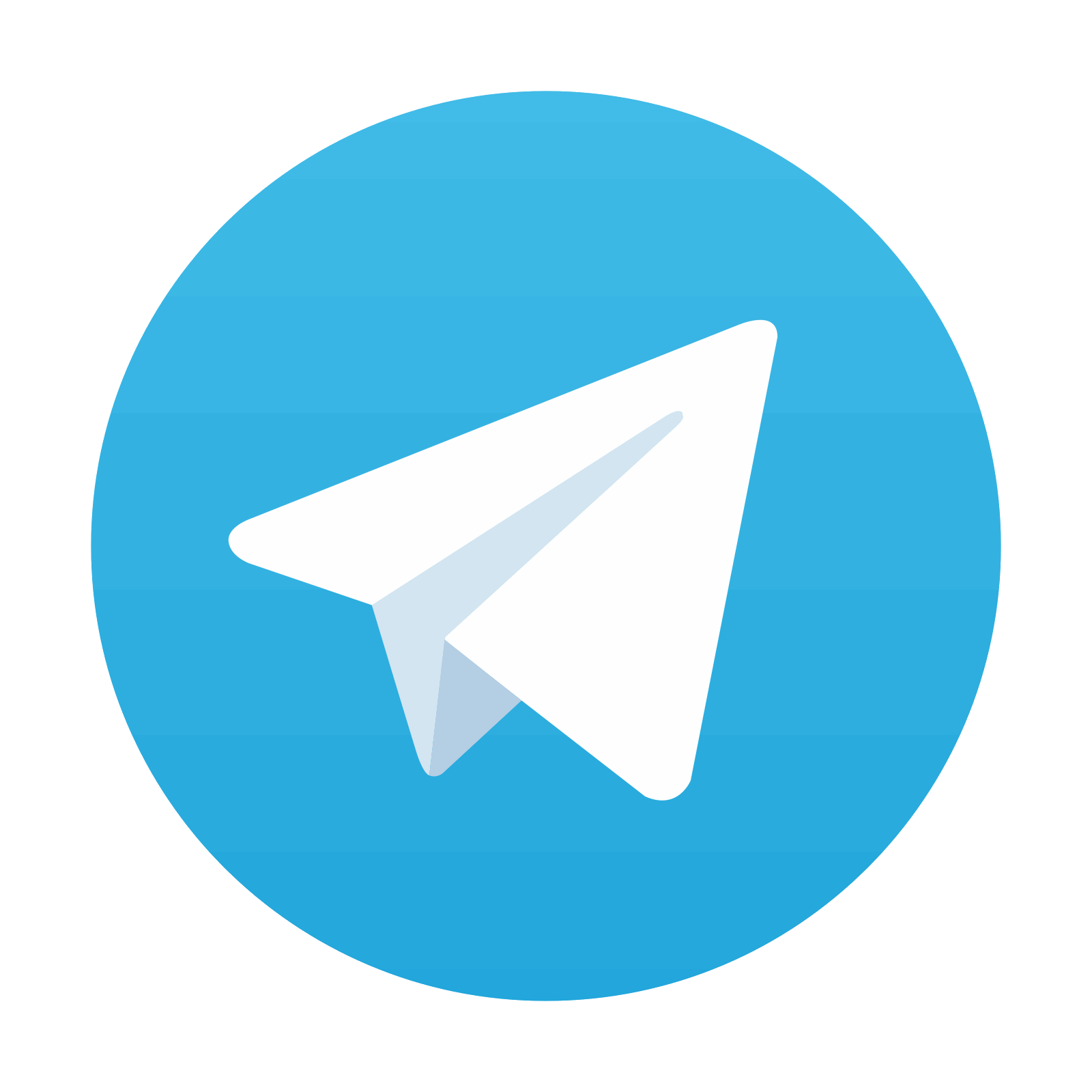
Stay updated, free articles. Join our Telegram channel

Full access? Get Clinical Tree
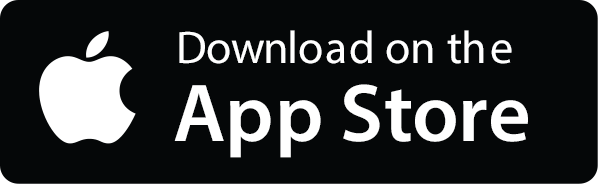
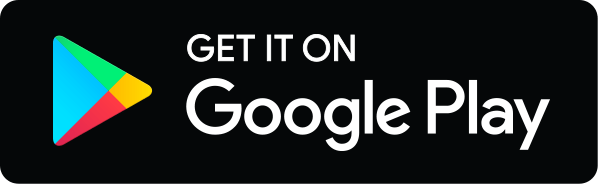