© Springer International Publishing Switzerland 2016
Debra G.B. Leonard (ed.)Molecular Pathology in Clinical Practice10.1007/978-3-319-19674-9_2222. Inherited Breast Cancer
(1)
Hebrew University Medical School, Jerusalem, Israel
(2)
Medical Genetics Institute, Shaare Zedek Medical Center, Jerusalem, Israel
(3)
Department of Pathology, Shaare Zedek Medical Center, Medical Genetics Institute, Jerusalem, Israel
Abstract
Inherited mutations in high-risk breast cancer-predisposing genes explain approximately 10 % of all breast cancer cases. Most of these mutations are in the BRCA1 and BRCA2 genes, as part of hereditary breast and ovarian cancer (HBOC). Others are in genes associated with syndromes which include a wider spectrum of malignancies and/or distinct clinical features. Molecular analysis, guided by clinical criteria and application of risk assessment models, currently reveal the underlying cause in roughly half of hereditary breast cancer families. Women who have inherited mutations in the HBOC genes have a high lifetime risk for both breast cancer and ovarian cancer. In BRCA1 and BRCA2 carriers, effective surveillance and prevention measures (such as risk-reduction salpingo-oophorectomy) reduce morbidity and mortality, and mutation status can enable targeted therapy (such as PARP inhibitors).
Clinical genetic analysis for suspected inherited predisposition to breast cancer, previously mostly limited to Sanger sequencing of BRCA1 and BRCA2, has been transformed by next-generation sequencing (NGS) technology, which enables rapid and simultaneous analysis of multiple genes. The ability of NGS assays to accurately and cost-effectively detect all classes of mutations, including large rearrangements, offers an important advantage over previous testing strategies. However, clinical interpretation of variants remains a significant challenge even in the well-studied BRCA1 and BRCA2 genes, let alone other breast cancer-associated genes. Testing numerous individuals is revealing an ever greater number of rare variations, whose effect on gene and protein function remains unclear. Reporting such variants of unknown significance is not driven by their clinical utility, and there is an urgent need for improved strategies to assess their functional and clinical effects. Testing genes beyond BRCA1 and BRCA2 is further complicated by the current lack of evidence-based guidelines for surveillance and prevention measures, even for carriers of mutations in genes considered to be clear moderate-risk predisposition genes.
Nevertheless, identifying cancer-predisposing mutations is increasingly feasible, will lead to optimized, personalized care for mutation carriers, and is likely to provide insights of broader relevance to cancer.
Keywords
Breast cancer BRCA1 BRCA2 HBOC (hereditary breast and ovarian cancer)Risk assessmentNGS (next-generation sequencing)PARP inhibitorsRR-BSO (risk-reducing salpingo-oophorectomy)Multiple gene testing panelsIntroduction
Breast cancer is the most common invasive cancer in women and in Western countries is the leading cause of cancer deaths in women after lung cancer. Breast cancer risk is affected by both environmental/behavioral and genetic factors, reflecting complex disease etiology. Established risk factors include age, family history of breast cancer, reproductive behavior, hormonal exposures, lifestyle habits, and personal breast-specific history (e.g., mammographic density, previous breast cancer, breast biopsy features of benign lesions) [1]. Family history of breast cancer is the most significant risk factor after female gender and older age. In the Swedish Family-Cancer Database, the attributable risk of a positive family history was estimated at about 11 % [2]. The risk to relatives of a person with breast cancer increases with closer degree of relationship and with younger age at cancer diagnosis [3]. Presence of an affected first-degree relative confers a relative risk of 1.76–2.96, depending on the age at diagnosis and the tumor’s receptor status [4]. Male breast cancer is rare in general and has a higher genetic component than female breast cancer [5].
The Molecular Basis of Inherited Breast Cancer
Inherited mutations in high-risk breast cancer-predisposing genes explain 8–10 % of all breast cancer cases [6]. Most of these mutations are in the BRCA1 and BRCA2 genes, which were identified in 1994 and 1995, respectively, as the bases of hereditary breast-ovarian cancer syndrome (HBOC). More rarely, breast cancer predisposition is caused by mutations in genes associated with other syndromes which include a wider spectrum of malignancies and/or distinct clinical features. Examples include TP53 (Li-Fraumeni syndrome), PTEN (Cowden syndrome), and STK11 (Peutz-Jeghers syndrome). Molecular analysis guided by clinical criteria [7, 8] and application of risk assessment models (see below) currently reveal the underlying cause in roughly half of hereditary breast cancer families [9].
The remaining cases have been the subject of intense investigation in the past 15 years, but this has not led to the discovery of additional major breast cancer genes. Although some familial aggregates may be explained by mutations conferring moderate (two- to three-fold) risks, e.g., CHEK2 or ATM mutations, or by combinations of low-risk alleles [10], a plausible explanation is that non-BRCA1–BRCA2 hereditary breast cancer is caused by high-risk mutations in a large number of genes, with each gene mutated in only a small proportion of families [11]. This hypothesis is being tested, with the advent of genome sequencing in clinical practice. Indeed, RAD51C [12] and RAD51D [13] mutations have been identified as rare causes of HBOC (approximately 1 % of non-BRCA1–BRCA2 families), and a significant number of new genes can be expected to be identified in the near future.
BRCA1 and BRCA2 Structure and Function
BRCA1 (NG_005905.2) and BRCA2 (NG_012772.1) were identified as genes mutated in HBOC, by genetic analysis in families with multiple cases of these malignancies [14, 15]. The BRCA1 gene on chromosome 17 encodes a 7.8 kb transcript composed of 24 coding exons that is translated to a 1,863 amino acid (220 kD) protein [16]. BRCA1 is a chromatin-interacting protein with an amino-terminal RING domain that has E3 ubiquitin ligase activity, a nuclear localization signal, and the BRCA1 C-terminal (BRCT) phosphopeptide-binding domain, which is conserved in multiple proteins involved in the DNA damage response (DDR). The BRCA2 gene on chromosome 13 encodes a 10.4 kb transcript composed of 27 exons that is translated to a 3,418 amino acid (380 kD) protein. BRCA2 contains a DNA-binding domain (DBD) that binds both single-stranded DNA (ssDNA) and double-stranded DNA (dsDNA), eight BRC repeats that bind RAD51, a key protein in homologous recombination (HR), and a C-terminal NLS [17].
Both BRCA1 and BRCA2 play important roles in the DDR, the cellular defense mechanism against genotoxic stress. The DDR is a multistage process that includes sensing ssDNA and dsDNA breaks (DSBs), mediating between sensors and repair effectors, and repairing DNA damage. Impairment of the DDR can lead to genomic/chromosomal instability, a hallmark of many malignancies, and indeed, cells lacking either BRCA1 or BRCA2 have multiple chromosomal aberrations, suggesting that both proteins function as “caretakers” of genomic integrity (reviewed in Ref. 17). Additional BRCA1 roles include estrogen receptor (ER) signaling [18], TP53 stabilization, and transcription modulation including heterochromatin-mediated silencing [19]. BRCA2 has more limited functions and, by recruiting RAD51, is primarily a co-effector of DNA repair by HR, in which the undamaged sister chromatid is used as an accurate template for DSB repair. The BRCA1-PALB2 complex is required for BRCA2 recruitment [17], and RAD51 binding to the BRCA2-BRC repeats facilitates recruitment of RAD51 to DSBs [20].
BRCA1 and BRCA2 Mutations
Mutational analysis is complicated by the large size of the BRCA1 and BRCA2 genes and the wide variety and distribution of mutations. Interpretation of test results is complicated by occurrence of variants of unknown significance (VUS, see below). Both public and commercial databases curate BRCA1 and BRCA2 mutations. The National Human Genome Research Institute hosts the Breast Cancer Information Core (BIC) (see URL list), which at the time of this writing includes 15,311 entries for BRCA1, with 1,787 distinct mutations, polymorphisms, and variants, 981 of which had been reported only once. For BRCA2, BIC contains 14,914 entries, including 2,000 distinct mutations, polymorphisms, and variants, 1,065 of which have been reported only once. Additional online databases include the Human Gene Mutation Database (HGMD, see URL list), the Leiden Open Variation Database (LOVD), and ClinVar (see URL list).
Founder Mutations
The frequency and spectrum of BRCA1 and BRCA2 mutations are highly dependent on ethnicity [21, 22]. While the majority of mutations are rare, “private” mutations observed in single cases or families, some mutations recur in particular ethnic groups and are called founder mutations. These founder mutations are thought to have occurred once in the history of an ethnic group and predominate because of population isolation and expansion. BRCA1 and BRCA2 testing is obviously simplified when founder mutations account for a high proportion of mutations in a specific ethnic group.
Founder BRCA1 and BRCA2 mutations have been described worldwide including in Norway [23], Sweden [24], Poland [25], and numerous other countries, as well as in many ethnic groups [21], including people of African ancestry [26]. Perhaps the most prominent examples are the founder mutations in Ashkenazi Jews (Jews of European origin) and in Iceland. Among Ashkenazi Jews, two BRCA1 mutations (185delAG and 5382insC) and one BRCA2 mutation (6174delT) are found in approximately 2.5 % of healthy individuals and account for approximately 10 % of all breast cancer cases, approximately one quarter of breast cancers diagnosed before age 40, and approximately 40 % of ovarian cancer at any age [27, 28]. Other BRCA1 and BRCA2 mutations are rare [29].
In Iceland, the frequency of the common BRCA2 mutation (999del5) is 0.4 % and accounts for 8.5 % and 7.9 % of breast cancer and ovarian cancer patients, respectively [30]. In addition, a rare BRCA1 founder mutation (G5193A) is present in 1 % of cases of breast cancer and ovarian cancer [21]. In males, these Icelandic founder mutations are responsible for about half of male breast cancer cases and a large percentage of prostate cancer in breast cancer families. Founder mutations also include deletions and duplications. The 6 kbp BRCA1 exon 13 duplication is an ancestral British mutation found in 10 of 1,831 (0.5 %) of affected families [31]. Three BRCA1 genomic deletions in exons 22, 13, and 13–16 account for as much as 36 % of BRCA1 mutations in families of Dutch ancestry [32].
Clinical Utility of BRCA1 and BRCA2 Testing
Cancer Risks in BRCA1 and BRCA2 Mutation Carriers
Women who have inherited mutations in the BRCA1 and BRCA2 genes have a high lifetime risk of breast cancer (56–84 %) [27, 33–35], an increased risk of contralateral breast cancer [27, 36–39], and a substantial lifetime risk of ovarian cancer (11–62 %, depending on the gene involved and the population studied) [27, 36, 37, 40, 41]. The average age at diagnosis of both breast and ovarian cancer is generally younger for BRCA1 carriers than for BRCA2 carriers, but each can manifest as breast cancer in the 20s. In addition to breast and ovarian cancer, an excess of male breast cancer occurs in BRCA2 families and to a lesser extent in BRCA1 families [29]. Lifetime risk of breast cancer is about 6 % for male BRCA2 carriers and is probably lower for male BRCA1 carriers. BRCA2 mutations also are associated with an excess risk for prostate cancer in males and for pancreatic cancer and melanoma in both sexes.
In fact, BRCA2 has been considered an important pancreatic cancer predisposition gene for many years [42], and more recent reports have estimated that it accounts for 6–12 % of pancreatic cancer families [43, 44]. BRCA1 and BRCA2 mutation carriers have a 2.5-fold and 3.5- to 10-fold increased risk of pancreatic cancer, respectively [45, 46], although absolute risks are low (<2 %) [47].
Risk Management in BRCA1 and BRCA2 Mutation Carriers
Effective cancer risk management strategies are available for BRCA1 and BRCA2 carriers as well as for families with a high clinical suspicion of genetic predisposition. HBOC management includes both surveillance and preventive measures, e.g., early breast cancer surveillance (from age 25 to 30 years) by annual mammography and breast MRI, chemoprevention for breast (e.g., tamoxifen) and ovarian (e.g., oral contraceptives) cancers, and risk-reducing (RR) surgery by mastectomy (RRM) and salpingo-oophorectomy (RRSO). Two large prospective studies [48, 49] showed that in BRCA1 and BRCA2 carriers, RRSO reduces overall mortality by 60–77 %. Breast cancer-specific mortality was reduced by 56 % and ovarian cancer-specific mortality by 79 % [48].
Testing Family Members
In the setting of a known familial mutation, identifying a relative as a noncarrier obviates the need for aggressive surveillance and prevention measures and provides reassurance to the individuals tested as well as to their offspring. Identifying that a person is a BRCA1 or BRCA2 carrier provides an opportunity for effective interventions.
Therapeutic Implications
Testing may have clinical implications for individuals newly or previously diagnosed with breast or ovarian cancer. Some evidence indicates that tumors in BRCA1 and BRCA2 mutation carriers are particularly sensitive to platinum-based chemotherapy (reviewed in Ref. 50), and a new class of compounds, poly-ADP-ribose-polymerase (PARP) inhibitors, were designed specifically to target BRCA-associated tumors. PARP inhibitors target the ssDNA repair pathway. Unrepaired ssDNA breaks convert to DSBs, which cannot be repaired by BRCA-deficient cells, resulting in selective synthetic lethality of these tumor cells [51]. A PARP inhibitor has already been approved by the FDA for advanced ovarian cancer in BRCA mutation carriers [52, 53], heralding the therapeutic utility of BRCA1 and BRCA2 testing in treatment selection. Responses have also been observed in BRCA carriers with pancreatic cancer, and clinical trials are underway with various PARP inhibitors in a variety of BRCA and BRCA-pathway-associated tumors [52, 54, 55].
BRCA1 and BRCA2 Testing
Breast Cancer Pathology in BRCA1 and BRCA2 Mutation Carriers
Gene expression analysis studies indicate five major breast cancer types: luminal A, luminal B, “normal breast-like,” HER2 amplified, and basal [56, 57]. Luminal A tumors tend to be of lower grade, showing tubule formation, lower proliferative activity, cellular pleomorphism, and estrogen (ER) and progesterone receptors (PR) expression, and lack of HER2 amplification. Luminal B tumors tend to be high grade (show high proliferative activity and pleomorphism, with little or no tubule formation), express ER and PR, and show variable HER2 expression and amplification. Basal-type carcinomas tend to be high grade, not to express ER, PR, or HER2 (i.e., are triple negative), and express the high molecular weight cytokeratins: 5/6 and 14 and/or EGFR1. This basal group includes medullary and atypical medullary breast carcinomas. The HER2 oncogene amplification subtype tends to be high grade and not to express ER or PR.
BRCA1-associated breast cancers tend to be basal-type, high grade, and show a syncytial growth pattern with metaplastic features more than sporadic and other familial breast cancers. Basal-type carcinomas are 27 times more likely to be associated with a BRCA1 mutation compared to other breast cancer subtypes [58]. In contrast, BRCA2-associated breast cancers have less distinct morphological characteristics. BRCA2-associated breast cancers tend to have luminal B-type features, i.e., tubule formation, “pushing” borders, high mitotic counts and proliferative activity (by Ki-67 immunostaining), and ER and PR expression. A differentiating point between BRCA2-associated tumors and characteristic sporadic tumors may lie in the discrepancy between their high proliferative activity relative to the “quiescent” features of hormone receptor expression and tubule formation [58] and in the tendency to have “pushing” borders [59]. However, pathological and expression profile differences are neither sufficiently sensitive nor specific to preclude BRCA1 or BRCA2 testing in cases lacking these features.
Immunohistochemistry for BRCA1 and BRCA2
Lack of BRCA1 or BRCA2 protein expression occurs in BRCA1- and BRCA2-associated tumors of almost all carriers (except those with missense mutations). This is a result of loss of the normal (nonmutant) allele. In affected persons, tumor BRCA1 and BRCA2 immunohistochemistry (IHC) is a plausible screening test to identify potential carriers, similar to IHC for mismatch repair protein expression to screen for hereditary nonpolyposis colon cancer (HNPCC). IHC for BRCA1 was originally confounded by conflicting evidence regarding its subcellular localization and lack of robust antibodies, and evidence suggested that it did not reliably identify BRCA1 carriers [60]. More recent studies demonstrated a 52 % positive predictive value (PPV) in ovarian cancer cases (86 % sensitivity and 78 % specificity for germline mutations) [61] and 80 % sensitivity and 100 % specificity in breast cancers [62]. These studies all used a nuclear staining monoclonal antibody (Ab-1/MS110) against the N-terminus. A reliable BRCA1 C-terminal antibody is not commercially available, precluding distinction between wild-type and truncated BRCA1 proteins. For BRCA2, IHC combining both N-terminal and C-terminal antibodies has been shown to be highly sensitive (95 %) and specific (98 %) in identifying potential carriers of BRCA2 truncating mutations [63]. However, BRCA1 and BRCA2 IHC is not widely used, probably due to lack of robust BRCA1 IHC, the lack of identification of missense mutations in either gene, and the existence of other mechanisms, e.g., promoter methylation, leading to loss of BRCA1 protein (Meisel et al. 2014).
Refining the morphological and IHC characteristics of carcinomas associated with specific mutations, and quantitative assessment of these associations, may still play a role in prioritizing genetic analysis. Even if new sequencing methods render prioritization superfluous, these correlates remain important for assessment of VUS, both in BRCA1 and BRCA2, and in other susceptibility genes.
BRCA1 and BRCA2 Genetic Testing Methods
Clinical and Pathological Correlates of BRCA1 and BRCA2 Mutations
The complexity of full molecular analysis of BRCA1 and BRCA2 has led to attempts to identify clinical, pathological, and gene expression correlates of mutations in these genes, to aid in selecting cases to test and prioritize the genes to be analyzed. Despite the excess of male breast and other cancers in BRCA2 vs BRCA1 families, breast and ovarian cancer are the predominant malignancies in both cases, so generally the types of cancers in a family cannot reliably distinguish BRCA1 from BRCA2. More importantly, approximately half of the affected female carriers do not have a significant family history that would have led to testing before their cancer diagnosis [27, 64].
Probability Models
Probability models were developed to estimate the likelihood that an individual or family has a BRCA1 or BRCA2 mutation. Testing costs were a major impetus for developing these models, and testing is commonly considered as indicated if the probability of mutation detection is at least 10 % (common in the USA and for privately funded testing) or 20 % (for public health services, e.g., the UK NHS NICE guidelines; see URL list). Although decreasing testing costs and increasing therapeutic implications lessen the relevance of such thresholds, estimating the likelihood of a HBOC mutation remains important because of the complexity of interpreting test results. In particular, the significance of a VUS is in part determined by the PPV calculated a priori by a prediction model.
Two broadly used models are BRCAPRO and Myriad II. BRCAPRO ([65], also see Cancer Pro in URL list) is a computer-based Bayesian probability model that uses breast and/or ovarian cancer family history to determine the probability that a BRCA1 or BRCA2 mutation accounts for cancer in the index case. The main parameters integrated in the risk assessment are the population prevalence of mutations, age-specific risks for carriers, and Ashkenazi Jewish heritage. The model is frequently updated, and the recent addition of breast tumor markers (hormone receptor and HER2 status) has improved prediction accuracy [66]). Myriad II (see URL list) is an online set of frequently updated prevalence tables categorized by ethnic ancestry (Ashkenazi Jewish or other), age of breast cancer diagnosis (<50 years or >50 years), and personal or family history of ovarian cancer. Myriad II is based on test results from the Myriad Genetic Laboratories commercial testing service [29]. Both models predict mutation presence more accurately than family cancer history alone and are part of the publicly available, convenient CancerGene software package (Cancer Pro, see URL list), which simultaneously calculates results for both models. In a direct comparison, BRCAPRO had higher sensitivity and similar specificity to Myriad II [67].
Other probability models include the Breast and Ovarian Analysis of Disease Incidence and Carrier Estimation Algorithm (BOADICEA) [68, 69], which performs as well as BRCAPRO [70], Tyrer-Cuzick [34, 71], and the Manchester Scoring System [72]. Notably, most prediction models were developed using data from non-Hispanic Caucasian women and may be less accurate in other populations. Both BOADICEA and BRCAPRO have poorer performance in African Americans and Hispanics [73], and BRCAPRO and Myriad II underpredicted Asian carriers [74].
Standard Genetic Testing Methods
Targeted Mutation Analysis
In relevant populations, targeted founder mutation testing remains the first step in BRCA1 and BRCA2 analysis. Because targeted analysis is simple, inexpensive, and detects only clearly pathological mutations, it can be offered to all women affected with breast/ovarian cancer in populations with founder mutations, even without prior risk assessment as detailed above [64]. Targeted mutation analysis techniques include PCR-RFLP which requires postamplification restriction enzyme digestion and TaqMan real-time allelic discrimination which is more rapid and scalable to high-throughput genotyping. If no founder mutation is detected, full analysis is considered based on the residual likelihood that a BRCA1 or BRCA2 germline mutation is present in the particular individual.
Mutation Scanning and Sanger Sequencing
Because Sanger sequencing large genes is both time- and cost-intensive, historically, various scanning techniques were used to detect BRCA1 and BRCA2 mutations, e.g., single-strand conformation polymorphism (SSCP) and high-performance liquid chromatography (HPLC). However, sensitivity of scanning is low, with nearly one-third of BRCA1 and BRCA2 mutations detectable by sequencing are missed by scanning methods [75]. Therefore, despite higher costs, Sanger sequencing of BRCA1 and BRCA2 coding exons and flanking intronic sequences has been the gold standard for non-rearrangement mutation analysis. An important concern with any sequencing technique is identification of VUS which are found in 5–10 % of all fully sequenced individuals ([76] and discussed below).
BRCA1 and BRCA2 Genomic Rearrangement Testing
Large deletions or insertions are estimated to account for 12–18 % of BRCA1 mutations but are less frequent in BRCA2 and in Ashkenazi Jews [77–79]. Such rearrangements are not detectable by Sanger sequencing. In the USA, until many claims of the Myriad patent for BRCA1 and BRCA2 testing was invalidated by the US Supreme Court, standard testing included both Sanger sequencing and screening for four large deletions and duplications in BRCA1, specifically large deletions in exons 13, 14–20, and 22, and duplication of exon 13. In a study of 20,000 patients, 66 of 2,634 (2.5 %) deleterious mutations were rearrangements [80]. The exon 13 duplication represented 80 % of rearrangement mutations and 2 % of total mutations, making this the most prevalent non-Ashkenazi mutation [80]. Outside the USA, testing for large rearrangements has been comprehensive, revealing that approximately 10 % of BRCA1 and BRCA2 mutations are genomic rearrangements ([79], [81]). Genomic rearrangement testing has usually been performed using PCR-based quantitative methods such as multiplex ligation-dependent probe amplification (MLPA) [82]. Even with the advent of next-generation sequencing (NGS) technologies, detection of large genomic rearrangements remains challenging, requiring very high coverage, so complementing NGS with specific rearrangement testing has been advocated [83, 84].
Next-Generation Sequencing of BRCA1 and BRCA2
BRCA1 and BRCA2 testing has become integral to the clinical management of women with a personal or family history of breast or ovarian cancer [77] and has significantly increased the number of tests performed. In parallel, NGS testing methods allow higher throughput and lower cost per sequenced base. NGS can generate gigabases of nucleotides of sequence data in a single instrument run [85, 86], enabling sequencing of human exomes and genomes (NGS as a method is described in Chap. 59). NGS testing for BRCA1 and BRCA2 analysis [83, 87–91] has become routine in many clinical laboratories in the last five years.
NGS for point/small mutation analysis was originally reported using the GAIIx-Illumina MPS platform on templates produced by long-range PCR of the BRCA1, BRCA2, and TP53 genes [87] or by in-solution capture using custom-designed complementary RNA oligonucleotide baits for 21 breast/ovarian cancer predisposition genes including BRCA1 and BRCA2 [82]. A multiplex bar-coded amplicon pyrosequencing method for BRCA1 and BRCA2 analysis was also reported (Roche/GS-FLX) [88, 89], with 98 % sensitivity. Only 3 of 133 (2 %) variants were not detected, which were all deletions or duplications in homopolymers of >7 nucleotides, a known limitation of pyrosequencing [88, 89]. All other studies ([82, 87], Hernan et al. 2012, [91]) reported 100 % sensitivity for point mutations and small insertions/deletions (indels). Specificity ranged from 84 % [87] to 100 % [82, 88, 89]. Bar coding strategies that enable pooling samples in one run are available and further reduce sequencing costs without compromising accuracy.
Next-Generation Sequencing for BRCA1 and BRCA2 Genomic Rearrangements
Detecting genomic rearrangements is possible with some but not all NGS strategies. De Leeneer et al. reported a lack of detection of large rearrangements, which is typical of NGS using short reads from short-fragment libraries [88, 89]. In contrast, Walsh identified all six large rearrangements tested (160 bp to 101 kbp in size) by comparing the number of reads at each base pair in each sample to the number of reads at the same base pair for all other samples [82]. This read depth analysis was possible because of significantly higher read depth (>1,200 vs 38 in Ref. 88, 89) and use of a mate-paired library template. In mate-paired libraries, template DNA fragments of known size (typically 2–5 kbp) are circularized and re-sheared, so that both ends of the original fragment are adjacent to each other (“mates”) in a new fragment which is sequenced. The original distance between the sequenced “mates” is known and can be compared to the expected distance in the reference sequence. This approach is now commonly used in genome-wide detection of structural rearrangements [92, 93].
The ability of NGS assays to accurately and cost-effectively detect all classes of mutations, including large rearrangements, offers an important advantage compared to previous testing strategies, which required multiple techniques to achieve comprehensive BRCA1 and BRCA2 analysis.
NGS Testing for Breast Cancer Predisposition Genes Beyond BRCA1 and BRCA2: Advantages and Challenges
In recent years, testing has expanded to cancer predisposition genes other than BRCA1 and BRCA2. These genes can be defined as genes in which rare mutations confer a greater than two-fold relative breast cancer risk [94]. Advances in technology have allowed development of multiplex gene panels in which many genes can be assessed simultaneously by NGS. NGS has made large-scale, high-throughput testing available and affordable. The advantage of this approach is an efficient evaluation that may be only slightly more expensive than standard-of-care genetic testing (or in the case of serial testing even less expensive) [95]. For familial cancer conditions, exome sequencing is gradually becoming more successful and is identifying new breast cancer risk genes [96, 97]. Identification of non-syndromic genes will remain challenging until it is possible to interpret data from testing of thousands of individuals. Analytical prioritization strategies will thus have high utility over the next few years [98].
Identifying a cancer-predisposing mutation will lead to optimized, personalized care for mutation carriers and will probably provide insights of broader relevance to cancer.
Currently, over 20 breast cancer predisposition genes are associated with at least a moderately increased risk for breast cancer, including BRCA1, BRCA2, TP53, PTEN, STK11, CHEK2, ATM, BRIP1, PALB2, CDH1, RAD51C, RAD51D, and more genes are likely to be identified in the future [99]. Although approximately 5 % of high-risk patients with negative BRCA results can be expected to carry a mutation in CHEK2 or TP53 [77], these and other genes were often tested only selectively [100] because of the low expected yield per gene, compounded by high sequencing costs. NGS testing has revolutionized the possibility of multiple gene testing. NGS testing of over 20 genes has been reported at less than half the cost of commercial testing of only BRCA1 and BRCA2 [82], and NGS-based panels of multiple cancer-related genes are available in clinical laboratories. Multiple gene testing is also being performed for other familial cancers, such as in Lynch (HNPCC) syndrome and pancreatic cancer [101]. In 278 young onset (<40 years) breast cancer patients in which previous BRCA1 and BRCA2 testing was negative, a 20-gene NGS panel revealed a deleterious or likely deleterious mutation in 11 % [102]. A similar yield of 11.4 %, after excluding BRCA1 and BRCA2 mutations, was observed in a study of 198 persons including a mix of affected and unaffected high-risk individuals [103].
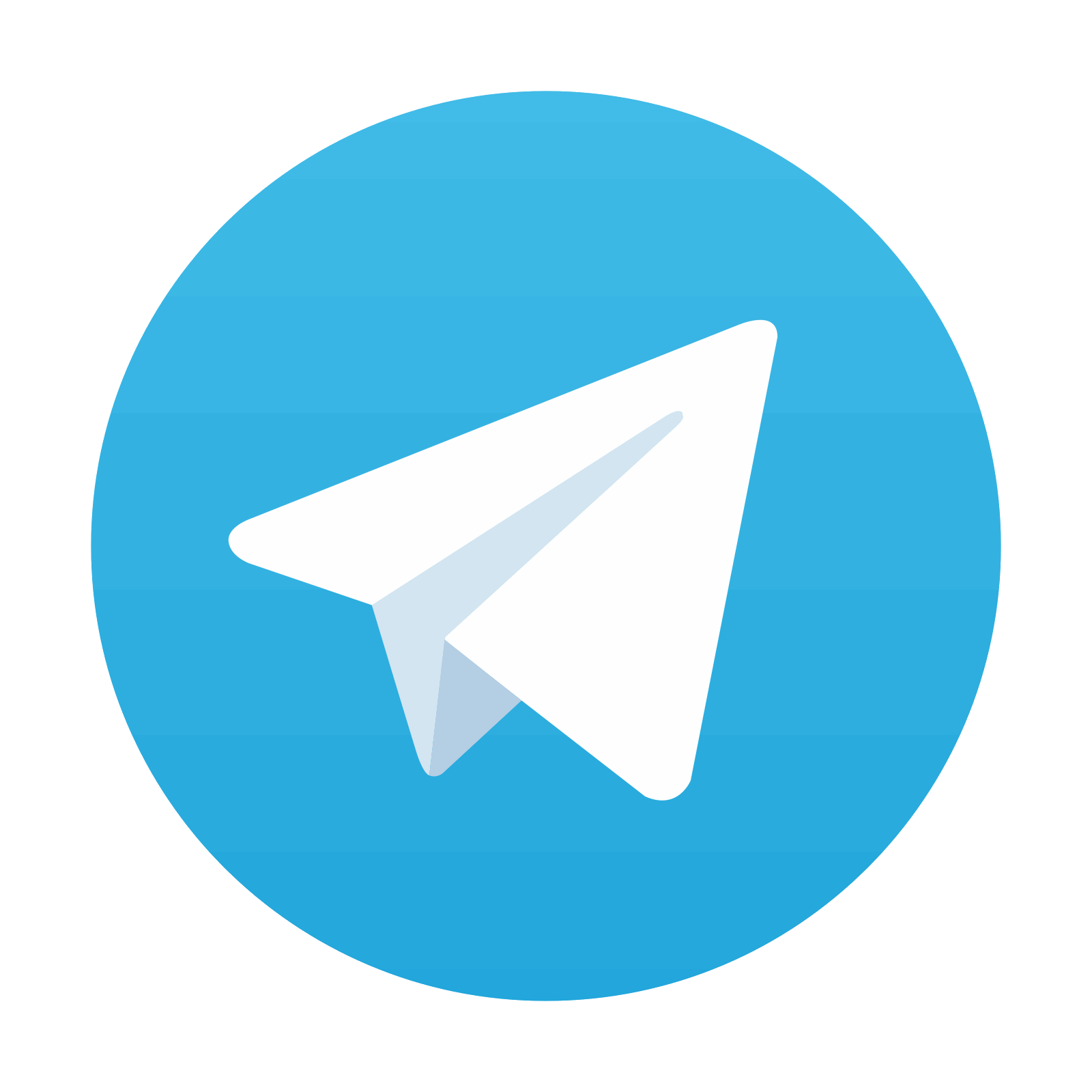
Stay updated, free articles. Join our Telegram channel

Full access? Get Clinical Tree
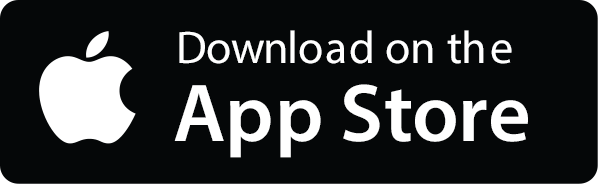
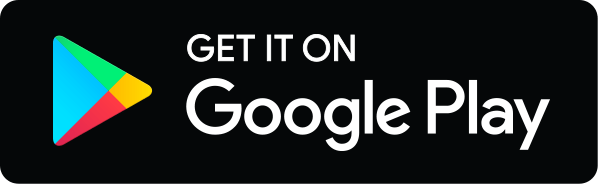