Herpesviruses
Herpes simplex 1 and 2
Varicella-zoster
Epstein-Barr
Cytomegalovirus
Human herpesvirus 6
Enteroviruses
Poliovirus
Coxsackievirus
Echovirus
Numbered enteroviruses
Parechoviruses
Human parechovirus 1–14
Arboviruses
See Table 48.2
Childhood illness associated
Measles
Mumps
Rubella
Rabies
Vesicular stomatitis virus
JC polyoma virus
Human immunodeficiency virus type 1
Table 48.2
Arthropod-borne viruses (arboviruses) and endemic areas
Family | Genus | Virus | Predominant regions |
---|---|---|---|
Togaviridae | Alphavirus | Eastern equine | Eastern, southern USA; Canada; Central, South America |
Western equine | Western, central USA; Central, South America | ||
Venezuelan equine | Central, South America; eastern, western USA | ||
Flaviviridae | Flavivirus | St Louis | USA; South America |
Powassan | Canada; USA; Russia | ||
Tick-borne | Central Europe; Russia | ||
Japanese | Asia; Russia; India; Sri Lanka | ||
Murray Valley | USA | ||
West Nile | North America; Europe; Middle East; Africa; Asia; Australia; Oceania | ||
Bunyaviridae | Bunyavirus | California groupa | California; upper Midwest; West Virginia; Virginia; Kentucky; Tennessee; |
North Carolina; Alabama | |||
Reoviridae | Coltivirus | Colorado tick fever | Western mountain USA |
Encephalitis occurs at a lower frequency than meningitis, with arboviruses being the leading cause both worldwide and in the USA [8–10]. Flaviviruses and alphaviruses are the most frequent arboviruses causing encephalitis and are responsible for the majority of mosquito and tick-borne encephalitis that cause epidemic and endemic disease in Asia, Europe, and the Americas [8–10]. Case fatality rates vary greatly, ranging from 5–70 %. Human immunodeficiency virus (HIV) and rabies virus (genus Lyssavirus) are other important causes of encephalitis worldwide [1, 2]. Herpes simplex virus (HSV) CNS infections affect all ages, occur at all times of the year, and have the highest encephalitis mortality rate in the USA [1–4]. In a small number of cases, childhood viral diseases including rubella, measles, varicella-zoster virus (VZV), mumps, and human herpes virus 6 (HHV-6) can progress to neurologic disease during primary infection or with viral reactivation (VZV and HHV-6). Cytomegalovirus (CMV) [11–13] and JC polyomavirus (JCV) [14] are responsible for encephalitides in immunocompromised patients.
Overview of Diagnostic Testing for Viral CNS Disease
Traditionally, the diagnosis of viral CNS infection has been based on laboratory findings in conjunction with patient history, clinical manifestations, and geographic and epidemiologic factors [1–4, 8, 9]. The diagnosis of viral encephalitis requires supplemental tests, including lumbar puncture, radiographic imaging such as computed tomography (CT) scans and magnetic resonance imaging (MRI) studies, and in some cases brain biopsy.
In general, patients with viral meningitis typically have a cerebrospinal fluid (CSF) pleocytosis with 10–500 leukocytes/mm3, a slightly elevated protein (<100 mg/dL), and a glucose level greater than 40 % of a simultaneously drawn serum sample [1, 2]. However, there can be a tremendous range of values that often overlap with those indicative of bacterial meningitis. Patients with viral meningitis or encephalitis may or may not have a CSF pleocytosis [15]. Therefore, normal CSF cell counts should not be used as a sole exclusion criterion for both diagnosis and determining which samples should be tested by molecular methods for viral pathogens. CSF glucose levels can be normal or low, as seen with bacterial meningitis. Encephalitis can lead to hemorrhagic necrosis with elevated protein levels and the presence of red blood cells.
Negative cultures for bacterial, fungal, and mycobacterial pathogens can aid in the diagnosis of viral CNS infections but can take several weeks for definitive results. However, bacterial cultures may be falsely negative if patients have been treated with antibiotics prior to sample collection. Traditionally, the identification of the specific virus relied on viral culture, serologic detection of virus-specific IgM and IgG antibodies (either systemic or intrathecal), or both [3, 4, 16–18]. However, the ability to isolate the virus is highly dependent on the viral species, time of sample collection, sample handling and processing, and prior treatment of the patient with antiviral agents. In only approximately 10–16 % of viral meningitis cases is the causative agent identified using viral culture [3, 4, 7, 16–19]. Serologic diagnosis can be made from serum in certain cases as early as 5 days after infection with the development of specific IgM antibodies, but often takes weeks to demonstrate a diagnostic rise in IgG antibody titers between acute and convalescent serum samples [3, 4, 14, 16–18, 20, 22]. Some patients with West Nile viral infections have detectable IgM antibodies in the serum for up to 500 days after infection, making the distinction between recent and past infection difficult [22]. In addition, immune status of the patient can affect the development of virus-specific antibodies. Since CNS infection may compromise the integrity of the blood–brain barrier, interpretation of CSF serology can be problematic.
Currently, molecular amplification methods that detect viral pathogens in CSF play a critical role in the rapid and accurate diagnosis of viral CNS infections [16, 18, 19]. This approach has largely abrogated the need for brain biopsy for the diagnosis of encephalitis and, in cases where biopsy is needed, can be used to detect viral pathogens in tissue specimens. In most cases, CSF is easily obtained, especially compared to brain biopsy. CSF should be stored frozen, preferably at ≤70 °C, to maintain the stability of viral nucleic acids. Isolation of the nucleic acids is achieved using a variety of extraction methods (described in Chap. 2) that also remove amplification inhibitors and neutralize any DNases or RNases present in the sample. The volume of CSF required can vary significantly and is dependent on the viral target, relative levels of virus present in the CSF, and the sensitivity of the testing method.
Results from molecular tests can be available within 24 h and possibly as soon as 2 h for applications utilizing real-time PCR technologies that incorporate amplification and detection in one step. This is in contrast to viral culture and serology, which can require up to 28 days for a final result. In the case of enteroviral infections, rapid detection of this pathogen has been shown to direct the selection of appropriate therapy, decrease the number of patients unnecessarily placed on empiric antibiotic therapy, shorten length of hospitalization, and save medical costs [23–26]. Overall, molecular amplification assays are highly sensitive and, depending on the virus and amplification target, can detect as low as one viral particle per reaction. High assay specificity is obtained by gene-specific targeting and often is significantly more sensitive and specific than serologic testing, which can demonstrate cross-reactivity among related viruses. Multiplex assays offer the versatility of screening for several pathogens in one test. Quantitative assays are useful for differentiating active from latent infection for herpesviruses and for monitoring response to antiviral therapy.
The last decade has seen a significant increase in the availability of commercially developed systems, assays, and reagents for molecular detection of viral infections. Table 48.3 lists the currently available US Food and Drug Administration (FDA)-cleared systems/assays and a representative selection of commercially available analyte-specific reagents (ASRs) that can be used in laboratory-developed tests (LDTs). This is not intended to be an exhaustive list, but represents several that are available in the US market. In addition, many types of reagents and kits are available from other manufacturers, including many that are Conformité Européenné (CE)-marked and not available in the USA.
Table 48.3
Commercial molecular assays and reagents available in the USA for detection of relevant viral agents
Target | Manufacturer | Test name | Method |
---|---|---|---|
US FDA-cleared a for CSF | |||
Enterovirus (EN) | bioMérieux, Inc. | NucliSENS EasyQ Enterovirus | Real-time NASBA |
Cepheid | Xpert EV | Real-time PCR | |
Herpes simplex virus | Focus Diagnostics | Simplexa HSV 1 and 2 | Scorpion probes, real-time PCR |
Analyte-specific reagents b | |||
Cytomegalovirus (CMV) | Epoch Biosciences/Nanogen | CMV primers and probes | MGB Alert, real-time PCR |
EraGen Biosciences | CMV primers ASR | Multicode bases, real-time PCR | |
Focus Diagnostics | Simplexa CMV ASR | Scorpion probes, real-time PCR | |
bioMérieux/Argene | Herpes Generic Consensus and Identification Assays | Hybridization probes, PCR | |
Enterovirus (EV) | Epoch Biosciences/Nanogen | Enterovirus | MGB Alert, real-time PCR |
EraGen Biosciences | Enterovirus primers ASR | Multicode bases, real-time PCR | |
Focus Diagnostics | Simplexa Enterovirus ASR | Scorpion probes, real-time PCR | |
Herpes simplex virus (HSV) | Cepheid | HSV 1 and 2 | TaqMan hydrolysis probes, real-time PCR |
Epoch Biosciences/Nanogen | HSV 1 and 2 | MGB Alert, real-time PCR | |
EraGen Biosciences | HSV primers ASR | Multicode bases, real-time PCR | |
Roche Diagnostics | HSV 1 and 2 ASR | FRET probes, real-time PCR | |
bioMérieux/Argene | Herpes Generic Consensus and Identification Assays | Hybridization probes, PCR | |
Human herpes virus 6 (HHV-6) | bioMérieux/Argene | Herpes Generic Consensus and Identification Assays | Hybridization probes, PCR |
Varicella-zoster virus (VZV) | EraGen Biosciences | VZV primers ASR | Multicode bases, real-time PCR |
Focus Diagnostics | Simplexa VZV 1 and 2 ASR | Scorpion probes, real-time PCR | |
bioMérieux/Argene | Herpes Generic Consensus and Identification Assays | Hybridization probes, PCR |
This chapter reviews the advances in the molecular testing for the most common causes of viral meningitis and encephalitis, including the enteroviruses, herpesviruses, and arboviruses. In addition, viruses affecting persons with immune suppression, including human immunodeficiency virus type 1 (HIV-1), JCV, and CMV are briefly discussed.
Enteroviruses and Parechoviruses
Epidemiology and Disease
Human enteroviruses are small, nonenveloped, single-stranded RNA viruses that are distributed into seven species (human enteroviruses A, B, C, and D and human rhinoviruses A, B, and C) of the Picornaviridae family (www.picornaviridae.com; accessed June 2014). The non-polio enteroviruses, including the coxsackieviruses, echoviruses, and numbered enteroviruses, are responsible for approximately 50 million infections per year in the USA and possibly more than a billion worldwide [4, 27, 28]. Enteroviruses cause an array of illnesses in both adults and children, including respiratory, ocular, cardiac, gastrointestinal, and neurologic diseases, as well as skin and oral eruptions [27, 28]. In the neonate, enteroviruses can cause a sepsis-like picture or meningoencephalitis, which can be severe. Outside the neonatal period, children under 5 years of age appear to be the most susceptible to infection, partly due to a lack of acquired immunity and poor hygienic habits. Encephalitis is uncommon and meningitis is rarely associated with complicated disease and poor clinical outcome; enteroviral meningitis may be more severe in adults than in children. Although enteroviral infections can occur year-round, the majority of the infections in temperate climates occur during the summer through autumn months.
Human parechoviruses are related but molecularly distinct members of the Picornaviridae that were originally classified as additional serotypes of enteroviruses (echovirus 22 and 23) because of similarities in clinical and laboratory characteristics [6]. However, parechovirus has now been established as its own genus based on extensive molecular studies. Increased surveillance and molecular phylogenetic studies have identified 16 distinct types [6]. The epidemiology and clinical presentation of parechovirus infection overlap with enteroviruses. While neonates and young children are primarily affected by both viruses, parechovirus infections in persons over age 10 have rarely been reported [6].
Laboratory Diagnosis
Many enteroviruses can be cultured in human and primate cell lines [27]. No single cell line is optimal for all enteroviral types, and therefore several different cell lines susceptible to enteroviral infection typically are used for clinical testing. Viral CSF culture has a sensitivity of approximately 65–75 %, in part because of the lability of the virus and possible low levels in the CSF. Furthermore, not all enteroviral serotypes are able to be recovered in tissue culture, including several coxsackievirus A strains that require mouse inoculation for detection. Isolation of enteroviruses in culture can take 3–8 days and therefore is generally not rapid enough to affect either treatment options or length of hospitalization, resulting in unnecessary antibiotic therapy until bacterial CSF cultures are negative at 48–72 h. Several serologic assays can be used to diagnose enteroviral infections, but are not clinically useful because they can be cross-reactive, nonspecific, and difficult to interpret due to the extended incubation and prodromal periods found with many enteroviral illnesses. In addition, culture of parechoviruses is also difficult, so the detection of both enteroviruses and parechoviruses is primarily performed using molecular methods.
Molecular Tests
To increase the sensitivity of enterovirus detection and to reduce the diagnostic turnaround time, molecular tests have been developed [19, 24, 29–36]. The molecular testing methods utilize either reverse transcription-polymerase chain reaction (RT-PCR) amplification combined with enzyme-linked immunosorbent assay (ELISA) [24, 29, 31–34, 36], real-time RT-PCR [35], or nucleic acid sequence-based amplification (NASBA) combined with molecular beacon technology [30]. The assays utilize primer sequences selected from the conserved 5′ untranslated region (5′ UTR) of the enteroviral genome. Sequence variations are present in this region, and the scope of detection of the various enteroviral serotypes is dependent on primer sequence selection [24, 29–36]. Since different serotypes circulate in distinct areas of the world, assays should be validated using local clinical isolates and reference strains known to be endemic in the region. Overall, enterovirus molecular tests are highly sensitive, some detecting the majority of enteroviral isolates at as low as 0.1 tissue culture infectious dose 50 (TCID50) [24, 29–36]. The molecular enterovirus tests are generally very specific, but have been shown to cross-react with rhinovirus [29, 35]. The significance of this cross-reactivity is discussed later in this chapter.
The availability of commercial reagents for molecular detection of enteroviruses has increased substantially in recent years. Two systems for the detection of enteroviruses from CSF samples are US FDA-cleared: Xpert EV (Cepheid, Sunnyvale, CA; 2007) and NucliSENS EasyQ Enterovirus (bioMérieux, Durham, NC; 2008) (Table 48.3). Cepheid’s Xpert platform is a closed, integrated extraction and real-time amplification and detection system using TaqMan hydrolysis probe chemistry. This platform is capable of single unit testing, with a total testing time of approximately 1.5 h. bioMérieux’s NucliSENS EasyQ system is based on NASBA isothermal RNA amplification with real-time target detection using molecular beacons, with a total testing time of 5 h. Both assays target 5′ UTR of the enterovirus genome.
A representative list of ASRs available commercially is presented in Table 48.3. These ASRs can be used on different amplification and detection platforms, providing flexibility for the design of LDTs. Analytical assessments of the Xpert assay have demonstrated very good performance characteristics. In a multicenter study comparing Xpert to a combined standard of clinical and laboratory data to define true infection, Xpert demonstrated 94.7 % sensitivity and 100 % specificity, with an overall accuracy of 98.6 % [37]. When used in a point of care test setting to maximize clinical impact of the results, Xpert also performed well (100 % sensitivity, 98.9 % specificity) for samples for which a result was obtained [38]. However, the authors reported a 16 % invalid rate for Xpert EV on initial testing, and these samples were not included in the calculations of performance. This invalid rate was similar to an 8 % rate from a previous study that assessed the performance of Xpert during routine use [39]. These authors reported that the invalid rate could be reduced by sample dilution or a single freeze–thaw without impacting the assay performance characteristics.
Although US FDA-cleared in 2008, comparatively fewer studies have been published evaluating the performance of the EasyQ system (bioMérieux). One study conducted by Landry et al. prior to US FDA clearance of this system compared an early version of the real-time NASBA assay to the original NASBA assay combined with electrochemiluminescence (ECL) detection of enterovirus in CSF and stool samples [30]. The real-time NASBA assay was 91 % sensitive and 100 % specific compared to NASBA-ECL upon initial testing of 160 samples, with an indeterminate rate of 2.5 %. A more recent report assessing the CE-marked version of the real-time NASBA EasyQ assay demonstrated a 90 % sensitivity and 100 % specificity compared to an LDT using a conventional RT-PCR method [40]. Marlowe et al. evaluated the Xpert assay compared to both the EasyQ assay and a TaqMan-based LDT, with 25/25 positive samples detected by Xpert, 24/25 detected by the LDT, and 21/25 detected by EasyQ [41]. These authors also showed an initial 7.2 % (10/138) invalid rate for Xpert EV, which was attributed to a single lot of reagents, with eight of ten resolved upon repeat testing with a new reagent lot.
CE-marked assays for the detection of enteroviruses in CSF are available from bioMérieux/Argene Biosoft (Varilhes, France). Enterovirus Consensus Assay utilizes RT-PCR and stair primer technology that was designed to overcome the problems associated with sequence divergence in the primer-binding regions, thus ensuring the detection of all enteroviral serotypes [29]. The assay was shown to be more sensitive than assays using the original Rotbart [32, 33] and Zoll [36] primers, and only exhibited cross-reactivity with rhinovirus type 3. Studies have determined that the sensitivity of the assay is <0.4 TCID50 and can detect approximately six RNA copies per input reaction.
Implementation of molecular testing for enteroviruses into routine use can positively impact the management of patients with suspected meningitis. Multiple studies have shown shorter duration of hospitalization, decreased use of inappropriate antibiotics, reduced ordering of ancillary laboratory tests, and overall decreases in health-care costs when enterovirus results are rapidly available [23–26]. These benefits have the potential to be maximized with systems such as Xpert, and should continue to improve with the increased development of more on-demand, near-patient platforms.
Molecular detection of parechoviruses has been limited to the use of RT-PCR LDTs in a limited number of clinical laboratories. Similar to assays for detection of enteroviruses, most assays for the detection of parechoviruses target the 5′ UTR, with recently described assays capable of detecting all known parechovirus types [42]. Several studies which have retrospectively analyzed stored CSF specimens have demonstrated an overall prevalence for parechoviruses of 2–3 % in CNS infections, but prevalence in individual years can vary widely from 0–10 % [6, 43–46].
Herpesviruses
The herpesviruses, including herpes simplex virus types 1 and 2 (HSV-1, HSV-2), VZV, CMV, and HHV-6, are large (150–200 nm), enveloped viruses with a linear, double-stranded DNA genome packaged in an icosahedral capsid. These viruses cause a broad spectrum of viral CNS disease including meningitis, encephalitis, meningoencephalitis, myelitis, and polyradiculitis [1, 2]. Epstein-Barr virus (EBV) DNA has been detected in virtually all AIDS-related cases of primary CNS lymphomas [47]. CMV is discussed briefly under diseases associated with immunosuppression and in detail in Chap. 47. The role of HHV-7 in CNS disease remains unclear.
Herpes Simplex Virus
Epidemiology and Disease
HSV-2 is the usual cause of HSV meningitis and accounts for approximately 1–5 % of all cases of viral meningitis and 4–6 % of cases of viral encephalitis overall (adult and pediatric) [1–4, 48, 49]. The clinical course of the disease usually is self-limiting and generally not associated with permanent neurological damage. HSV-2 also causes Mollaret’s meningitis, a benign recurrent meningitis [50].
HSV-1 is responsible for the majority of HSV-associated encephalitis and for 10–20 % (approximately 1,250 cases per year in the USA) of all viral encephalitis cases (adult and pediatric). Approximately 30 % are primary HSV-1 infections, and the remaining cases are due to HSV reactivation. In contrast to HSV meningitis, HSV encephalitis can be a devastating disease, resulting in persistent focal neurologic disease due to damage to one or both temporal lobes [1–4, 48, 49]. Encephalitis occurs in approximately 60–75 % of babies with disseminated HSV disease, and the mortality rate for cases of HSV-2 encephalitis can be as high as 80 % without appropriate antiviral therapy [51].
Diagnosis
CSF viral cultures are positive only in approximately 15 % of HSV meningitis cases due to primary infection and, with the exception of neonatal infection, are rarely positive in cases of HSV encephalitis [1–3, 32, 33, 48, 49, 51]. Traditionally, the diagnosis of HSV encephalitis was dependent on obtaining tissue by brain biopsy for viral culture, electron microscopy, immunohistochemical staining, and the demonstration of intrathecal production of HSV-specific antibodies [1–3, 16–18, 49, 51].
Over the last decade, studies comparing HSV CSF PCR with brain biopsy and/or intrathecal HSV-specific antibody production for the diagnosis of HSV CNS infections have demonstrated sensitivities and specificities ranging from 96–98 % and 96–99 %, respectively [16, 51–53]. HSV PCR can identify infected persons who fail to either seroconvert with primary infection or demonstrate significant rises in antibody titers after reactivation. Finally, HSV PCR can aid in differentiating HSV antibody increases due to active HSV CNS infection from reactivation in persons with concomitant CNS disease not related to HSV. Another advantage of PCR assays for diagnosis of HSV encephalitis is that the assay sensitivity remains high (95 %) up to approximately 1 week after initiating therapy [52]. However, CSF samples collected early after symptom onset may be negative in some patients with HSV CNS disease, so repeating the lumbar puncture and HSV PCR testing is recommended in such cases when HSV infection is strongly suspected [54]. Caution should be exercised if using CSF PCR for test of cure since DNA can be slow to clear from the CSF (as many as 21 % of CSF specimens can remain PCR positive for more than 15 days after successful completion of therapy) [52]. Although HSV viral loads are not correlated with prognosis [55, 56], persistent detection of HSV in the CSF of neonates with encephalitis has been shown to be a poor prognostic indicator [56, 57].
Varicella-Zoster Virus
Epidemiology and Disease
The most common manifestation of VZV resulting from primary infection is chickenpox and generally occurs in early childhood [58]. VZV-associated neurologic syndromes include acute cerebellar ataxia, diffuse or focal encephalitis, meningitis, transverse myelitis, and Reye syndrome [1, 2, 58]. Herpes zoster (also known as shingles), resulting from the reactivation of latent VZV infection, occurs in approximately 20 % of immunocompetent persons (generally 45 years or older) and the incidence is approximately 15 % higher in persons with immunosuppression [58, 59]. In a small percentage of all zoster cases, severe complications can occur, including meningitis, encephalitis, myelitis, Ramsay Hunt syndrome, Guillain-Barre syndrome, and contralateral hemiplegia [58–60]. Ocular manifestations are more common in reactivation disease, such as herpes zoster opthalmicus and acute retinal necrosis, especially in immunocompromised individuals and those not treated with antiviral therapy [61, 62]. Interestingly, concomitant herpes zoster rash may only be present in less than half of patients with VZV CNS disease [63].
Diagnosis
Studies have demonstrated that the recovery of VZV in culture is poor (20 % positive), as is serologic diagnosis (48 % positive) [64]. Antigen detection by immunofluorescent staining improves detection from vesicular lesions (82 % positive), whereas PCR was the most sensitive, with a detection rate of approximately 95 % [64]. Based on improved detection with PCR assays, VZV accounts for 6–30 % of all herpesviruses detected from CSF [20, 21, 64–71]. However, results need to be interpreted cautiously since VZV DNA has been detected in CSF without overt disease, particularly in immunosuppressed individuals.
Human Herpes Virus 6
Epidemiology and Disease
HHV-6 is generally acquired early in life and is manifested as exanthem subitum (more commonly known as roseola), or as a non-exanthemous febrile illness, sometimes accompanied by severe neurologic manifestations, including febrile seizures, meningitis, meningoencephalitis, and encephalitis [72]. Detection of HHV-6 in children with febrile seizures is important for identifying children with a potential for the recurrence of seizure episodes due to ongoing HHV-6 infection. Immunocompromised patients can manifest severe HHV-6 reactivation infections, including encephalitis [72].
Diagnosis
Culture confirmation of HHV-6 infection is not routinely performed in clinical virology laboratories due to the complexity of the testing, which requires purification and culture of patient lymphocytes or co-cultivation of activated patient lymphocytes and activated human umbilical cord blood lymphocytes [72]. Serologic confirmation of the disease depends on the detection of IgM in primary infection or conversion from either a negative to a positive IgG antibody response or a fourfold or greater rise in IgG antibody titer [72]. However, antibody titers can be difficult to interpret for several reasons: IgM antibodies may not develop in some children, can be positive with HHV-6 reactivation, and can remain positive for extended periods of time; and significant rises in HHV-6-specific IgG antibody titers can be found during infections with other herpesviruses. The detection of HHV-6 DNA in the CNS by PCR can support the diagnosis; however, in a percentage of cases, HHV-6 DNA can be found in normal brain tissue and in the CSF of children without evidence of CNS disease [72]. Although rare, chromosomal integration of HHV-6 can complicate the interpretation of positive molecular tests from body fluids, and may result in the misdiagnosis of acute CNS infection [73]. Quantitative methods may be more accurate for correlating the presence of HHV-6 with active disease [74, 75].
Molecular Tests for Herpesviruses
Comparisons of the sensitivity of HSV PCR to viral culture, antibody detection, and direct immunofluorescence assays have clearly established the utility of PCR as a first-line diagnostic test in the clinical laboratory [7, 16, 18, 19]. Most PCR assays are applicable to the majority of conventional or real-time PCR instruments. The assays for herpesviruses use different primer sets targeting a number of genes including: DNA polymerase gene (HSV-1, HSV-2, CMV, EBV, VZV, HHV-6); UL42, glycoprotein B (gB), glycoprotein D (gD), or thymidine kinase (TK) genes (HSV); genes 28 and 29 (VZV); and major capsid protein, U89/U90 (HHV-6). Identification of the specific herpesvirus is achieved using either traditional methods, including the use of species-specific primers, hybridization with species specific probes, agarose gel electrophoresis, restriction enzyme analysis, ELISA-based colorimetric detection methods [20, 21, 64, 66–68, 70], or fluorescent probe technologies that permit real-time detection [65, 69, 71]. One commercial assay has been US FDA-cleared for the detection of herpesviruses in CSF (Table 48.3, Simplexa™ HSV 1 and 2 Direct Kit, Focus Diagnostics, Cypress, CA). Two assays are US FDA-cleared for the detection of HSV from oral and anogenital lesions: the Multicode—RTx HSV 1 and 2 kit (EraGen Biosciences, Madison, WI) and the IsoAmp HSV assay (Biohelix, Beverly, MA). A third assay is US FDA-cleared for the detection of HSV from anogenital lesions: the BD ProbeTec™ Herpes Simplex Viruses (HSV 1 and 2) Q x Assay (Becton Dickinson, Sparks, MD). None of these US FDA-cleared HSV tests for lesions should be used for the diagnosis of HSV CNS infections.
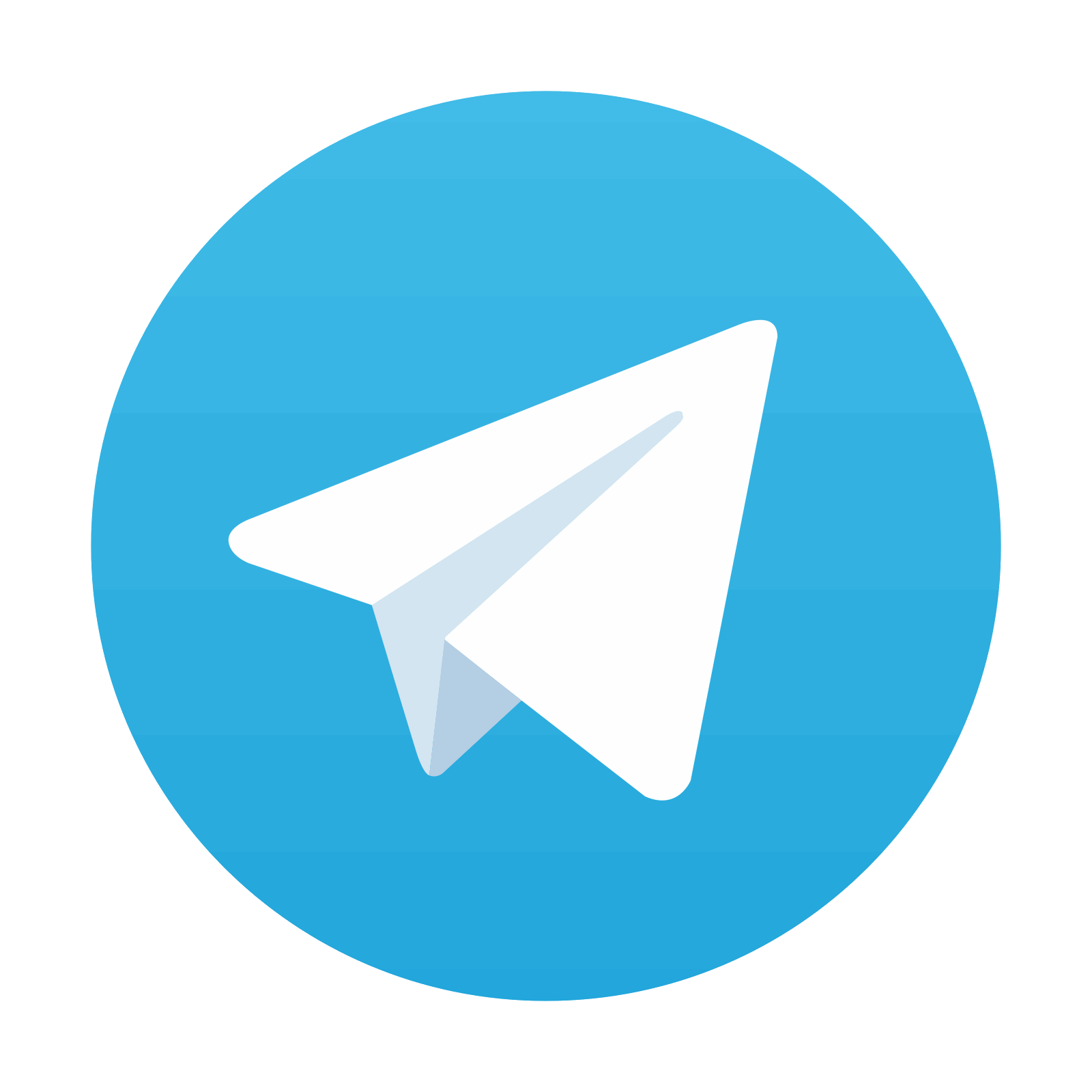
Stay updated, free articles. Join our Telegram channel

Full access? Get Clinical Tree
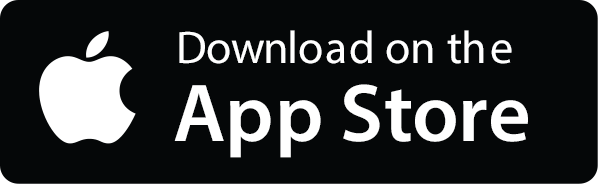
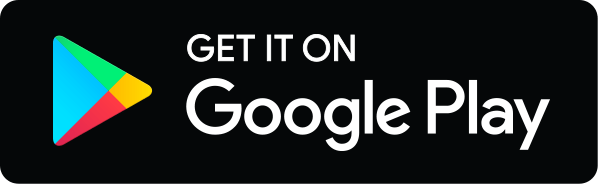