mtDNA mutations
Gene
Associated disorders
m.583G>A
MT–TF
MELAS, mitochondrial myopathy and exercise intolerance [27]
m.1494C>T
MT–RNR1
Maternally inherited deafness or aminoglycoside-induced deafness [27]
m.1555A>G
MT–RNR1
Account for majority of patients with mitochondrial non-syndromic hearing loss and aminoglycoside induced hearing loss [28]
m.1606G>A
MT–TV
Ataxia, myoclonus and deafness [27]
m.3243A>G
MT–TL1
MELAS (m.3243A>G present in ~80 % of cases) [14]; MIDD (m.3243A>G present in ~2–7 % of patients) [25]; LS [14]; Hypertrophic cardiomyopathy (m.3243A>G present in ~10 % of Finnish patients) [25]; Sensorineural hearing loss, focal segmental glomerulosclerosis, cardiac plus multi-organ dysfunction [27]
m.3271T>C
MT–TL1
MELAS (m.3271T>C present in ~7.5 % of cases) [29]
m.3256C>T
MT–TL1
MELAS [27]
m.3260A>G
MT–TL1
Maternal myopathy and cardiomyopathy [27]
m.3291 T>C
MT–TL1
MELAS, myopathy, deafness plus cognitive impairment [27]
m.3302A>G
MT–TL1
Mitochondrial myopathy [27]
m.3303C>T
MT–TL1
Maternal myopathy and cardiomyopathy [27]
m.3460G>A
MT–ND1
m.3635G>A
MT–ND1
LHON [27]
m.3697G>A
MT–ND1
MELAS/LS/LHON and dystonia [27]
m.3700G>A
MT–ND1
LHON [27]
m.3733G>A
MT–ND1
LHON [27]
m.3733G>C
MT–ND1
LHON [31]
m.3890G>A
MT–ND1
Progressive encephalomyopathy/LS/optic atrophy [27]
m.4171C>A
MT–ND1
LHON [27]
m.4298G>A
MT–TI
Chronic progressive external ophthalmoplegia/multiple sclerosis [27]
m.4300A>G
MT–TI
m.4308G>A
MT–TI
Chronic progressive external ophthalmoplegia [27]
m.4332G>A
MT–TQ
Encephalopathy/MELAS [27]
m.5537AinsT
MT–TW
LS [27]
m.5650G>A
MT–TA
Myopathy [27]
m.5703G>A
MT–TN
Chronic progressive external ophthalmoplegia/mitochondrial myopathy [27]
m.7445A>G
MT–CO1
Sensorineural hearing loss [27]
m.7472insC (=C7471CC)
MT–TS1
Progressive encephalopathy/ataxia, myoclonus and deafness/motor neuron disease-like [27]
m.7497G>A
MT–TS1
Mitochondrial myopathy/exercise intolerance [27]
m.7511T>C
MT–TS1
Sensorineural hearing loss [27]
m.8344A>G
MT–TK
m.8356T>C
MT–TK
m.8363G>A
MT–TK
m.8993T>G
MT–ATP6
m.8993T>C
MT–ATP6
m.9176T>G
MT–ATP6
m.9176T>C
MT–ATP6
m.9185T>C
MT–ATP6
LS/ataxia syndromes/NARP-like disease [27]
m.10010T>C
MT–TG
Progressive encephalopathy [27]
m.10158T>C
MT–ND3
LS [27]
m.10191T>C
MT–ND3
LS/Leigh-like disease/epilepsy, strokes, optic atrophy and cognitive decline [27]
m.10197G>A
MT–ND3
LS/dystonia/stroke/LHON and dystonia [27]
m.10663T>C
MT–ND4L
LHON [27]
m.11777C>A
MT–ND4
LS [27]
m.11778G>A
MT–ND4
m.12147G>A
MT–TH
MERFF-MELAS/encephalopathy [27]
m.12315G>A
MT–TL2
Chronic progressive external ophthalmoplegia/Kearns–Sayre syndrome [27]
m.12706T>C
MT–ND5
LS [27]
m.13513G>A
MT–ND5
m.13514A>G
MT–ND5
LS/MELAS [13]
m.14459G>A
MT–ND6
m.14482C>G
MT–ND6
LHON [27]
m.14482C>A
MT–ND6
LHON [27]
m.14484T>C
MT–ND6
m.14487T>C
MT–ND6
m.14495A>G
MT–ND6
LHON [27]
m.14568C>T
MT–ND6
LHON [27]
m.14674T>C
MT–TE
Reversible COX deficiency myopathy [27]
m.14709T>C
MT–TE
Single Large-Scale Rearrangements
More than 120 single deletions, duplications and complex rearrangements associated with a primary mtDNA disorder have been published and recorded in the mtDNA mutation database, Mitomap (www.mitomap.org). The size of disease-causing mtDNA single deletions varies from several base pairs to 10 kb. The large-scale single deletions (>1 kb) are the most common rearrangements and account for approximately 97 % of the reported pathogenic deletions of the mtDNA and >99 % of patients with mtDNA deletion associated diseases. Greater than 80 % of deletions are associated with direct repeats of 3–13 bp in length (www.mitomap.org). The most common large-scale deletions are between a 13 bp direct repeat from m.8470 to m.8482 in the ATPase8 gene and from m.13447 to m.13459 in the ND5 gene. Large duplications of mtDNA almost always coexist with large-scale single deletions and have been observed in a subset of patients who harbor large-scale mtDNA single deletions [41–45]. MtDNA duplications alone are not disease causing, although they may have modifying effects on the phenotype caused by mtDNA deletions [42, 43]. In about 20 % of patients with primary mtDNA disorders, the disorder is caused by large-scale single deletions in mtDNA [1].
Patients with large-scale single deletions usually have one of the three mitochondrial DNA deletion syndromes: Pearson syndrome, KSS, or progressive external ophthalmoplegia (PEO). The clinical features of the major mtDNA deletion syndromes are described in Table 10.2. In Pearson syndrome, mtDNA deletions are usually more abundant in blood than in other tissues. In PEO, mtDNA deletions are confined to skeletal muscle. In KSS, approximately 90 % of patients have a large-scale (i.e., 1.1–10 kb) mtDNA deletion. Deletions are usually present in all tissues of individuals with KSS, and may be identified in blood leukocytes. However, in many cases, the level of heteroplasmy of the deletion is too low in blood cells to be detected, and a muscle biopsy may be necessary [41]. Pearson syndrome patients who survive infancy may develop KSS at a later age [46]. An individual may have a large-scale mtDNA deletion abundant in blood and undetectable in muscle at an early age and have Pearson syndrome. If this individual survives, the same deletion can be abundant in muscle and undetectable in blood at a later age and cause KSS.
mtDNA deletion syndrome | Disease characteristics | Characteristics of mtDNA deletions |
---|---|---|
KSS | A triad of (1) onset <20 yo, (2) pigmentary retinopathy, and (3) PEO, plus at least one of the following: cardiac conduction block, cerebrospinal fluid protein concentration greater than 100 mg/dL, or cerebellar ataxia. | ~90 % have a large-scale 1.1–10 kb deletion usually present in all tissues, but most abundant in muscle, and often undetectable in blood cells. A deletion of 4,977 bp is the most common. Over 120 deletions have been associated with KSS. Large-scale duplications have also been reported. |
PEO | Ptosis, ophthalmoplegia, variably severe proximal limb weakness; relatively benign, may be the early sign of KSS. | Deletion/duplication analysis is estimated to identify a deletion in approximately 50 % of patients. Deletions are confined to skeletal muscle. |
Pearson syndrome | Sideroblastic anemia, exocrine pancreas dysfunction, usually fatal in infancy. Children who survive the disease usually go on to develop KSS. | Deletions are usually more abundant in blood than other tissue types. Deletion load gradually decreases in blood and increases in muscle as it evolving to PEO and KSS. |
Copy Number Changes (Depletion or Over-Replication)
Abnormal amounts of mtDNA, either decreases of copy number (depletion) or increases of copy number (over-replication), can be indicative of mitochondrial dysfunction. MtDNA depletion syndrome is a group of mitochondrial disorders characterized by a reduced amount of mitochondrial DNA in tissues. The disorders associated with mtDNA depletion syndrome generally involve neurological symptoms that occur during infancy or childhood. Symptoms may include muscle weakness, hypotonia, exercise intolerance, developmental delay, lactic acidosis, encephalopathy, hepatopathy, or myopathy. MtDNA depletion syndrome is usually caused by mutations in the nuclear genes involved in mtDNA biosynthesis or maintenance, such POLG, TYMP, C10ORF2, DGUOK, MPV17, etc.
Mitochondrial over-replication can be a cellular response to mitochondrial dysfunction and is characterized by ragged red fibers in the affected muscle specimens of patients with mtDNA mutations in tRNA genes or with large-scale mtDNA deletions [47, 48]. Over-replication of mtDNA also has been reported in a later-onset, mild KSS patient with a high heteroplasmy (92 %) for a deletion in the mtDNA [49].
Mutations in the Nuclear Genes
Nuclear genes encode the structural protein subunits that are components of the mitochondrial OXPHOS complexes, as well as proteins involved in biosynthesis, maintenance, transcription and translation of the mitochondrial DNA, proteins involved in the assembly of the OXPHOS complexes, and cofactors of the electron transport chain that are indirectly involved in the primary function of the OXPHOS system. Proteins needed for the assembly of an OXPHOS complex, referred to as assembly factors, are not usually components of the final structure. Fewer than 100 nuclear genes have been associated with disease-causing mutations for a primary mitochondrial OXPHOS disorder. These nuclear genes encode proteins from all the above categories. Although most of these nuclear gene-encoded proteins are synthesized in the cell cytoplasm and imported into the mitochondria, some of these proteins have dual localization (located in both mitochondria and cytoplasm), such as ISCU and LRPPRC, and some are localized in the cytoplasm but not localized in mitochondria, such as the small subunit of P53-inducible ribonucleotide reductase encoded by the RRM2B gene and thymidine phosphorylase encoded by the TYMP gene. As mtDNA genes also are involved in the structure and/or function of mitochondria, relevant mtDNA genes are discussed with nuclear genes in this section.
Genes Encoding OXPHOS Complex Structural Subunits or Assembly Factors
Complex I (CI) deficiency is the most common cause of mitochondrial disorders and accounts for about one-third of patients with primary mitochondrial disorders [50, 51]. Mutations in the mtDNA account for about 29 % of patients with confirmed isolated CI deficiency, while the rest are considered to be due to nuclear gene defects [8]. Mutations have been identified in 17 of the 39 nuclear genes encoding the structural subunits and in 10 nuclear genes encoding the assembly factors for CI (see Table 10.3). Mutations in these genes have been seen in approximately 20 % of patients with isolated CI deficiency [8]. Each gene accounts for less than 5 % of patients with CI deficiency [52]. The diseases resulting from these mutations range from severe lethal infantile mitochondrial disease, congenital lactic acidosis, encephalocardiomyopathy, and LS to adult onset muscle weakness, exercise intolerance, liver dysfunction, ataxia, and progressive neurodegeneration [52, 53]. Some genes with mutations expected to cause combined (multiple) OXPHOS deficiency, such as MT–TL1, MT–TS2, and MT–TW in the mtDNA, and POLG, SUCLA2, C10ORF2, and TAZ in the nuclear genome, can be associated with an isolated complex deficiency (Table 10.3). In contrast, patients with mutation(s) in CI subunit genes (either mtDNA or nuclear), can present with combined Complex I and III deficiency [54, 55], likely due to supercomplex formation of the respiratory chain [56–58].
Table 10.3
Genes associated with isolated OXPHOS deficiency and associated diseases
Isolated OXPHOS complex deficiency | Genes | Diseases and clinical features | References |
---|---|---|---|
CI | mtDNA-genes encoding structural subunits: ND1; ND2; ND3; ND4; ND4L; ND5; ND6 | LHON, LS, NARP, MELAS | |
nDNA-genes encoding structural subunits: NDUFS1; NDUFS2; NDUFS3; NDUFS4; NDUFS6; NDUFS7; NDUFS8; NDUFV1; NDUFV2; NDUFA1; NDUFA2; NDUFA9; NDUFA10; NDUFA11; NDUFA12; NDUFB3; NDUFB9 | Progressive encephalomyopathy; leukoencephalopathy with macrocephaly; LS; LLS; leukoencephalopathy; LIMD; cardiomyopathy and encephalopathy; progressive cavitating leukoencephalopathy; hypertrophic cardiomyopathy and encephalomyopathy; progressive neurodegeneration | ||
nDNA-genes encoding assembly factors: NDUFAF1; NDUFAF2; NDUFAF3 (C3ORF60); NDUFAF4 (C6orf66); NDUFAF5 (C20orf7); NDUFAF6 (C8ORF38); ACAD9; FOXRED1; NUBPL | Exercise intolerance; hypertrophic cardiomyopathy; encephalopathy; progressive encephalopathy; LS; encephalomyopathy; lactic acidosis; encephalomyopathy with macrocephaly; leukoencephalopathy, Leigh-like syndrome | ||
Other genes in the mtDNA: MT-TL1; MT-TS2; MT-TW | MELAS; Mitochondrial Myopathy; Mitochondrial Encephalopathy | ||
Other nDNA-encoded genes: POLG; SUCLA2; C10ORF2 | Liver involvement; encephalopathy; 3-methylglutaconic aciduria; epilepsy | ||
CII | nDNA-genes encoding structural subunits: SDHA | LS; cardiomyopathy | |
nDNA-genes encoding assembly factor: SDHAF1 | Infantile leukoencephalopathy | [84] | |
CIII | mtDNA-genes encoding structural subunits: MT-CYB | LHON; encephalopathy; cardiomyopathy, myopathy | |
nDNA-genes encoding structural subunit: UQCRB; UQCRC2; UQCRQ; CYC1 | Hypoglycemia; liver dysfunction; lactic acidosis; psychomotor retardation with extrapyramidal signs | ||
nDNA-genes encoding assembly factors: BCS1L; TTC19 | Encephalopathy; liver failure; tubulopathy; GRACILE syndrome; Björnstad syndrome | ||
CIV | mtDNA-genes encoding structural subunits: MT-CO1, MT-CO2, MT-CO3 | Encephalopathy; myopathy; sideroblastic anemia; myoglobinuria; MELAS | |
nDNA-genes encoding structural subunits: COX6B1; COX4I2 | Infantile encephalomyopathy, or exocrine pancreatic insufficiency and anemia | [90] | |
nDNA-genes encoding assembly factors: SURF1; SCO1; SCO2; COX10; COX14 (C12ORF62); COX15; COX20; LRPPRC; COA5 (C2orf64) | LS; French-Canadian LS; encephalopathy; cardiomyopathy; myopathy; liver failure; tubulopathy | ||
Other genes: ETHE1; FASTKD2; PET100; TACO1 | Ethylmalonic encephalopathy; Encephalomyopathy; LS | ||
CV | mtDNA-genes encoding structural subunits: ATP6; ATP8 | LS; NARP; cardiomyopathy | |
nDNA-genes encoding structural subunits: ATP5E | 3-methylglutaconic aciduria; lactic acidosis; mild mental retardation; peripheral neuropathy | [99] | |
nDNA-encoded assembly factor: ATPAF2 (ATP12); TMEM70 | Encephalopathy, lactic acidosis, cardioencephalomyopathy | ||
Other genes: SLC25A3 (PHC ) | Lactic acidosis; hypertrophic cardiomyopathy; muscular hypotonia | [103] |
Although Complex II (CII) has four known genes (SDHA, SDHB, SDHC and SDHD) encoding the structural subunits and two known genes (SDHAF1 and SDHAF2) encoding the assembly factors, only two genes, SDHA and SDHAF1, have been associated with CII deficiency, and present as LS or mitochondrial encephalopathy (see Table 10.3). The rest of the genes, SDHB, SDHC SDHD, and SDHAF2, are considered tumor suppressor genes, with mutations associated with hereditary paraganglioma and pheochromocytoma syndromes only, suggesting that mutations in these genes are involved in tumorigenesis [59].
Complex III (CIII) deficiency is relatively rare and accounts for only about 7 % of patients with an OXPHOS complex deficiency [51, 60]. Of the 11 structural subunits of CIII, mutations have been reported in the only subunit encoded by the MT–CYB gene in the mtDNA and 4 (UQCRB, UQCRC2, UQCRQ and CYC1) of the 10 subunits encoded by nuclear genes (Table 10.3). More than 20 pathogenic mutations in the MT–CYB gene account for the vast majority of known pathogenic mutations in the CIII subunits and most of these mutations are located outside of the transmembrane domain of the protein [61]. The UQCRB, UQCRQ and UQCRC2 genes each have one homozygous pathogenic mutation described in a single family and the CYC1 gene has two different homozygous pathogenic mutations identified in two unrelated patients. In addition, mutations in two genes (BCS1L and TTC19) encoding CIII assembly factors are associated with primary mitochondrial disorders due to CIII deficiency (Table 10.3). More than 20 pathogenic mutations in the BCS1L gene are in the human gene mutation database (HGMD) (www.hgmd.org) and account for the majority of patients with CIII deficiency. Clinical features range from mild congenital sensorineural hearing loss and distorted hair (pili torti), the hallmarks of Björnstads syndrome, tubulopathy alone or combined with cholestasis, and/or encephalopathy to GRACILE syndrome (growth retardation, amino aciduria, cholestasis, iron overload, lactic acidosis, and early death) [62–64].
Complex IV (CIV; Cytochrome c oxidase or COX) is the terminal component of the respiratory chain catalyzing the reduction of molecular oxygen to water. COX is composed of three catalytic subunits encoded by mtDNA genes and ten accessory subunits encoded by nuclear genes. The accessory subunits are involved in structural stabilization, assembly of the complex, and modulation of COX catalytic activity. Disease-causing mutations occur in all three mtDNA genes (MT–CO1, MT–CO2 and MT–CO3) encoding catalytic subunits of COX, but almost all the mutations were seen in a single family and are considered “private” mutations. Of the ten genes encoding accessory subunits of COX, only COX6B1 and COX4I2 (Table 10.3) have pathogenic mutations. In addition, mutations occur in nine nuclear genes (SURF1; SCO1; SCO2; COX10; COX14 [C12ORF62]; COX15; COX20; LRPPRC; COA5 [C2orf64]) encoding COX assembly factors that are not themselves components of the final COX structure, and account for the majority of patients with isolated COX deficiency (Table 10.3). The SURF1 gene represents the most common gene accounting for 25–75 % of LS associated with COX deficiency [65]. Furthermore, mutations in ETHE1, FASTKD2, and TACO1 also are associated COX deficiency (Table 10.3).
Complex V (ATP synthase) deficiency most commonly is caused by mutations in the mtDNA encoded ATP6 and ATP8 proteins, and as described earlier in this chapter, m.8993T>G and m.8993T>C are the most common mutations (Table 10.1). The severity of disease caused by these mutations depends on mutation load: a low mutation load is asymptomatic; an intermediate mutation load causes NARP; and a high mutation load causes MILS [66, 67]. Only two of the approximately 16 nuclear genes encoding the subunits of complex V (ATP5E) and two genes encoding the assembly factors, ATPAF2 (ATP12) and TMEM70, have mutations which cause ATP synthase deficiency (Table 10.3). The presentation of patients with isolated ATP synthase deficiency caused by mutations in nuclear genes is remarkably homogeneous with neonatal onset, lactic acidosis, hypertrophic cardiomyopathy, psychomotor retardation, and, in most cases, 3-methyl-glutaconic aciduria. These patients usually have diminished content of ATP synthase and lack striatal brain involvement [68].
Genes Encoding Proteins Essential for MtDNA Biosynthesis and/or Maintenance
Although mtDNA resides in mitochondria, its biosynthesis (replication), maintenance, transcription, and translation are controlled by proteins encoded by nuclear genes, synthesized in the cytoplasm, and imported into mitochondria [171]. The mtDNA copy number is related to energy demand, i.e., tissues with the highest respiratory demand, such as muscle, liver, heart, and brain, have the greatest number of mtDNA copies per cell. Normal mtDNA replication and maintenance is important for both the quality and quantity of mtDNA and requires normal function of the mtDNA replisome as well as normal mitochondrial deoxynucleoside triphosphate (dNTP) pool maintenance. Major proteins or enzymes involved in mtDNA replication include DNA polymerase gamma (with its catalytic subunits encoded by the POLG gene and its accessory subunit encoded by the POLG2 gene) and Twinkle helicase encoded by the C10ORF2 gene (also known as PEO1). Major proteins involved in the maintenance of the large dNTP pool are encoded by genes including TK2, TYMP, DGUOK, RRM2B, SUCLA2, SUCLG1, etc [24]. Mutations in genes involved in mtDNA replication or dNTP pool maintenance may cause quality or quantity defects of mtDNA. To date, disease-causing mutations have been identified in many genes involved in mtDNA replication or dNTP pool maintenance (Table 10.4). Mutations in POLG are the most common and account for approximately 25 % of all patients with mitochondrial disease presentations [172, 173]. Mutations in POLG, POLG2, ANT1, C10ORF2, RRM2B, and OPA1 have been reported to be associated with autosomal-dominant external ophthalmoplegia (adPEO) with multiple mtDNA deletions (Table 10.4). Mutations in POLG, C10ORF2 (PEO1), RRM2B, and SLC25A4 (ANT1) account for approximately 45 %, 15–35 %, 9 %, and 4–15 % of familial PEO with multiple deletions, respectively. Mutations in POLG2 are rare and account for less than 2 % of adPEO [173, 174]. Mutations in POLG and RRM2B are associated with autosomal-recessive external ophthalmoplegia (arPEO) with multiple mtDNA deletions (Table 10.4). Mutations in POLG, C10ORF2, RRM2B, DGUOK, MPV17, TK2, SUCLA2, SUCLG1, and TYMP are associated with different types of mtDNA depletion syndromes (Table 10.4). Approximately 69 % of patients with myopathic mtDNA depletion syndrome and more than 84 % of patients with hepatocerebral mitochondrial DNA depletion syndrome harbor mutations in one of these nine genes [175]. Mutations in genes involved in mtDNA biosynthesis and maintenance account for approximately 40 % of patients with combined OXPHOS deficiency [176].
Table 10.4
Genes associated with combined/multiple OXPHOS deficiency and associated diseases
Combined OXPHOS complex deficiency | Genes | Diseases and clinical features |
---|---|---|
CI + CIII + CIV | Genes involved in mtDNA replication | |
POLG | Mitochondrial DNA depletion syndrome 4A (Alpers type), MTDPS4A [104]; Mitochondrial DNA depletion syndrome 4B (MNGIE type), MTDPS4B [105]; adPEO with multiple mtDNA deletions [106]; arPEO with multiple mtDNA deletions [107]; Mitochondrial recessive ataxia syndrome, includes sensory ataxic neuropathy, dysarthria, and ophthalmoparesis (SANDO) and spinocerebellar ataxia with epilepsy (SCAE) [108, 109] | |
POLG2 | adPEO mitochondrial DNA deletions [110] | |
C10ORF2 | ||
Genes involved in dNTP pool maintenance | ||
DGUOK | Mitochondrial DNA depletion syndrome 3, MTDPS3 (hepatocerebral type) [113] | |
TK2 | Mitochondrial DNA depletion syndrome 2 (myopathic type), MTDPS2 [114] | |
TYMP | Mitochondrial DNA depletion syndrome 1 (MNGIE type); MTDPS1 [115] | |
RRM2B | Mitochondrial DNA depletion syndrome 8A (encephalomyopathic type with renal tubulopathy), MTDPS8A [116]; Mitochondrial DNA depletion syndrome 8B (MNGIE type), MTDPS8B [117] | |
SLC25A4 | ||
SUCLG1 | ||
SUCLA2 | ||
Other | ||
OPA1 | ||
MPV17 | ||
CI + CIII/CIV (or + CV) | Genes involved in tRNA modification | |
PUS1 | Mitochondrial myopathy; lactic acidosis; sideroblastic anemia 1 [129] | |
TRMU | Liver failure, acute infantile [130] | |
Elongation factors | ||
GFM1 | ||
TUFM | COXPD4 (OMIM#610678); Lactic acidosis; fatal encephalopathy; diffuse cystic leukodystrophy; micropolygyria [133] | |
TSFM | COXPD3 (OMIM#610505); Progressive encephalomyopathy or concentric hypertrophic cardiomyopathy [134] | |
Ribosomal protein | ||
MRPL3 | COXPD9 (OMIM#614582); Mitochondrial cardiomyopathy [135] | |
MRPL44 | COXPD15 (OMIM#615395); Hypertrophic cardiomyopathy [136] | |
MRPS16 | COXPD2 (OMIM#610678); Agenesis of the corpus callosum; dysmorphism; fatal neonatal lactic acidosis [137] | |
MRPS22 | ||
Genes encoding aminoacyl-tRNA synthetase | ||
AARS2 | COXPD8 (OMIM#614096); Fatal infantile hypertrophic mitochondrial cardiomyopathy [140] | |
DARS2 | Leukoencephalopathy with brain stem and spinal cord involvement and lactate elevation [141] | |
EARS2 | COXPD12 (OMIM#614924) [142] | |
FARS2 | COXPD14 (OMIM#614946) [143] | |
HARS2 | Ovarian dysgenesis; sensorineural hearing loss [144] | |
LARS2 | Perrault syndrome 4 [145] | |
MARS2 | Spastic ataxia 3 [146] | |
RARS2 | Pontocerebellar hypoplasia, type 6; profound developmental delay; progressive severe microcephaly; hypotonia; cerebral, pontine, and cerebellar atrophy [147] | |
SARS2 | Hyperuricemia; pulmonary hypertension; renal failure; alkalosis [148] | |
YARS2 | Mitochondrial myopathy; lactic acidosis; sideroblastic anemia 2 [149] | |
Genes encoding peptide release factor | ||
C12orf65 | COXPD7 (OMIM#613559); LS; optic atrophy; ophthalmoplegia [150] | |
Other | ||
ELAC2 | COXPD17 (OMIM#615440) [151] | |
MTFMT | COXPD15 (OMIM#614947) [152] | |
MTO1 | COXPD10 (OMIM#614702) [153] | |
NDUFS4 | Infantile encephalopathy; microcephaly; lactic academia [54] | |
TAZ | Barth Syndrome [154] | |
CI–III + CII–III | MtDNA encoded genes with mutations causing CoQ10 deficiency | |
MT–TL1 | (Secondary) CoQ10 deficiency in MELAS [155] | |
Nuclear genes with mutations causing CoQ10 deficiency | ||
ADCK3 (CABC1) | CoQ10 deficiency and progressive cerebellar atrophy with cerebellar ataxia and seizures [156] | |
APTX | ||
BRAF | (Secondary) CoQ10 deficiency with multisystemic infantile presentation in one patient with cardiofaciocutaneous syndrome | |
COQ2 | ||
COQ6 | Steroid-resistant nephrotic syndrome (SRNS) with sensorineural deafness (SND) [161] | |
PDSS1 | CoQ10 deficiency and multisystem disease with early-onset deafness, encephaloneuropathy, obesity, livedo reticularis, and valvulopathy [162] | |
PDSS2 | CoQ10 deficiency with infantile onset fatal LS and nephrotic syndrome [163] | |
COQ9 | CoQ10 deficiency with neonatal lactic acidosis, and multisystem disease [164] | |
ETFDH | (Secondary) Myopathic form of CoQ10 deficiency and multiple acyl-CoA deficiency [165] | |
CIII + CIV | PNPT1 | COXPD13 (OMIM#614932) PubMed: [166] |
CI + CII + CIV | GFER | Autosomal-recessive myopathy with cataract [167] |
CI + CII + CIII | ISCU | Hereditary myopathy with lactic acidosis [168] |
CI + CII + CIII | BOLA3 | Multiple mitochondrial dysfunctions syndrome 2; fatal deficiency of multiple respiratory chain and 2-oxoacid dehydrogenase enzymes [169] |
NFU1 | Multiple mitochondrial dysfunctions syndrome 1; fatal deficiency of multiple respiratory chain and 2-oxoacid dehydrogenase enzymes [169] | |
CI + CIII + CIV + CV | RMND1 | COXPD11 (OMIM#614922) [170] |
CI + CII + CIII + CIV + CV | AIFM1 | COXPD6 (OMIM#300816) [76] |
Genes Encoding Mitochondrial Translational Machinery
The 13 proteins encoded by mtDNA are synthesized in mitochondria using a nonuniversal genetic code as follows: UGA codes for tryptophan (W) instead of a stop (X) codon; AGA & AGG codes for a stop (X) codon instead of arginine (R); and AUA codes for methionine (M) instead of isoleucine (I). The mitochondrial translational machinery consists of two rRNAs and 22 tRNAs encoded by mtDNA and approximately 100 proteins encoded by nuclear genes, including initiation, elongation, and termination translation factors; up to 81 mitochondrial ribosomal proteins (MRPs); and approximately 19 mitochondrial aminoacyl-tRNA synthetases and methionyl-tRNA transformylase [177, 178]. Theoretically, mitochondrial protein synthesis deficiency can be caused by mutations in any of the components of the mitochondrial translational machinery and can result in OXPHOS deficiency affecting theoretically all complexes containing mtDNA-encoded subunits (CI, CIII, CIV, and CV).
To date, in addition to the two rRNAs and 22 tRNAs encoded by mtDNA with many disease-causing mutations reported to be associated with combined (multiple) complex deficiency (www.mitomap.org), pathogenic mutations associated with combined OXPHOS deficiency also have been reported in many nuclear genes encoding components of the mitochondrial translational machinery, including two genes involved in tRNA modification (PUS1 and TRMU), three genes encoding elongation factors (GFM1, TUFM, and TSFM), ten genes encoding aminoacyl-tRNA synthetase (AARS2, DARS2, EARS2, FARS2, HARS2, LARS2, MARS2, RARS2, YARS2, and SARS2), and one gene encoding peptide release factor (C12orf65) (Table 10.4). Of all the 81 human MRPs, mutations have been found in only four: MRPS16, MRPS22, MRPL3 and MRPL44 (see Table 10.4). Mutations in both MRPS16 and MRPS22 genes result in a marked decrease in the 12S rRNA transcript level, probably caused by impaired assembly of the mito-ribosomal small subunit, generating unincorporated and instable 12S rRNA [137, 138]. Similarly, mutations in both MRPL3 and MRPL44 genes result in a marked decrease in the 16S rRNA transcript level [135, 136].
Genes Involved in Mitochondrial Dynamics
Mitochondrial fusion and fission (collectively termed mitochondrial dynamics) are important for mitochondrial inheritance and for the maintenance of mitochondrial functions [179]. Three nuclear-gene encoded proteins are required for mitochondrial fusion: Mitofusins 1 and 2 (Mfn1 and Mfn2) for outer membrane fusion, and Opa1 for inner membrane fusion [180]. Dynamin-related protein Drp1 is required for mitochondrial division (fission) [181, 182]. OPA1 mutations cause autosomal-dominant optic atrophy [183, 184]. In most cases, this disease specifically involves degeneration of retinal ganglion cells. However, more diverse OPA1-related phenotypes have been described recently, and they include disorders of mtDNA maintenance [180]. Mutations in the MFN2 gene cause the autosomal-dominant Charcot–Marie–Tooth disease type 2A, which is a peripheral neuropathy caused by degeneration of axons in long sensory and motor nerves of the distal extremities [185]. A mutation in the DNM1L gene has been reported in a patient with lethal encephalopathy due to defective mitochondrial and peroxisomal fission. The patient presented in the first days of life with microcephaly, abnormal brain development, optic atrophy and hypoplasia, and lactic acidemia, and died at age 37 days. Overexpression of the mutant DNM1L from the patient in fibroblasts from control subjects induced aberrant mitochondrial and peroxisomal phenotypes, indicating that the mutation acted in a dominant-negative manner [186].
Genes Involved in CoQ10 Biosynthesis or Metabolism
Coenzyme Q10 (CoQ10), or ubiquinone, is a mobile lipophilic electron carrier critical for electron transfer by the mitochondrial inner membrane respiratory chain from Complex II and I to Complex III. Intracellular synthesis is the major source of CoQ10, although a small proportion is acquired through diet. CoQ10 is synthesized in the mitochondrial inner membrane. At least 12 genes are involved in COQ10 biosynthesis [187]. Primary CoQ10 deficiency is a rare, clinically heterogeneous autosomal recessive disorder with six major phenotypes [187, 188]: (1) an encephalomyopathic form with seizures and ataxia; (2) a multisystem infantile form with encephalopathy, cardiomyopathy, and renal failure; (3) a predominantly cerebellar form with ataxia and cerebellar atrophy; (4) LS with growth retardation; (5) an isolated myopathic form; and (6) nephrotic syndrome. Typical CoQ10 deficiency has relatively normal isolated Complex I, II, and III activity, but deficient I + III and II + III activity. Mutations in any of the genes involved in COQ10 biosynthesis may cause CoQ10 deficiency. To date, mutations in six nuclear genes, COQ2, ADCK3 (CABC1, COQ8), COQ6, COQ9, PDSS1, and PDSS2 have been reported to be associated with primary CoQ10 deficiency (Table 10.4). CoQ10 levels also can be affected by other genetic defects (such as mutations of mtDNA, ETFDH, APTX, and BRAF genes) that are not directly related to the CoQ10 biosynthetic process (see Table 10.4). CoQ10 deficiency can be treated by supplementation with CoQ10.
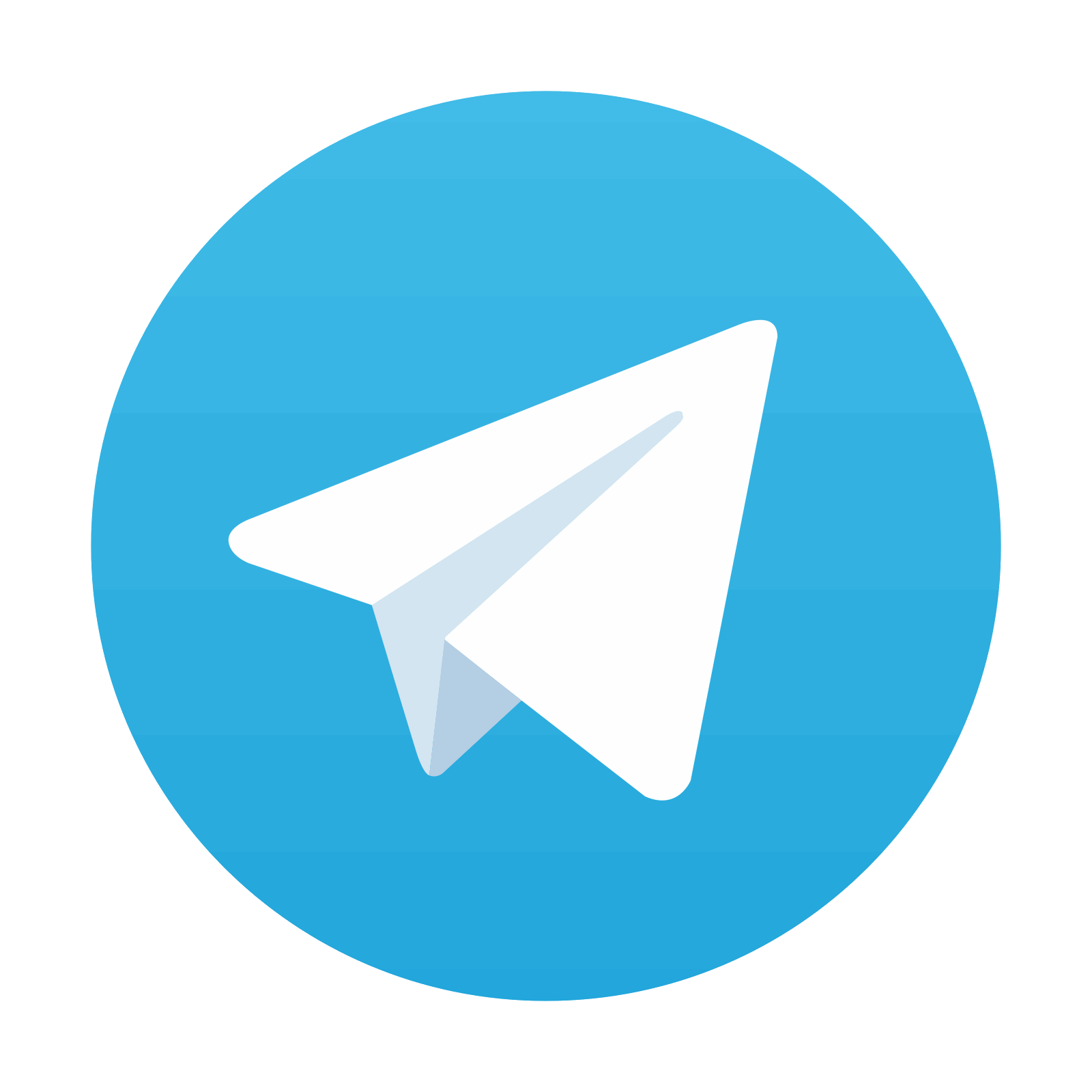
Stay updated, free articles. Join our Telegram channel

Full access? Get Clinical Tree
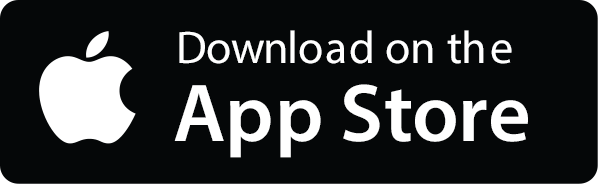
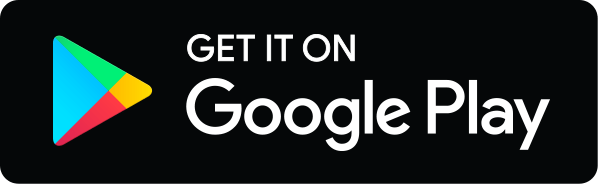