Figure 18–1 CNS neurotransmission and sites of drug action. CNS drugs act primarily by affecting the synthesis, storage, release, reuptake, or degradation of neurotransmitters (NTs) or by activating receptors. NTs are synthesized from precursors accumulated or synthesized in the neurons. The NTs are stored in vesicles whose membranes contain proteins involved in NT release (synaptobrevin and synaptotagmin). The NTs are released when an action potential–mediated calcium influx initiates interaction of synaptobrevin and synaptotagmin with neuronal membrane–docking proteins (syntaxin and neurexin). This leads to docking and exocytosis. Synaptic NTs can activate presynaptic (R3) or postsynaptic receptors (R1, R2). The action of NTs is terminated by reuptake into the presynaptic neuron or by enzymatic degradation.
Following exocytosis, the neurotransmitter may activate presynaptic and postsynaptic receptors. A neurotransmitter’s action is then terminated either by its reuptake into the presynaptic neuron or by its degradation to inactive compounds, with degradation catalyzed by enzymes located on presynaptic and postsynaptic neuronal membranes or within the cytoplasm.
Neurotransmitters can also diffuse from the synapse of their origin to affect neurons in the surrounding vicinity. In this way, different neurotransmitters released from different types of neurons form a chemical milieu, as described previously. The net influence of the chemical milieu on neurotransmission depends on the concentrations of the excitatory and inhibitory neurotransmitters acting at a particular synapse.
Excitatory and Inhibitory Neurotransmission
CNS neurotransmitters can evoke either an excitatory or an inhibitory synaptic membrane potential and trigger effects at presynaptic and postsynaptic sites on target neurons. If an excitatory postsynaptic membrane potential reaches firing threshold, an action potential is conducted along the dendritic and axonal membrane and evokes the release of a neurotransmitter from the nerve terminal. An inhibitory postsynaptic membrane potential hyperpolarizes the neuronal membrane and inhibits the firing of action potentials. Depending on whether a presynaptic membrane potential is excitatory or inhibitory, it will increase or decrease the release of a neurotransmitter from the neuron. Presynaptic receptors, also called autoreceptors, can also be coupled with cyclic adenosine monophosphate (cAMP) or other second messengers that modulate neurotransmitter release.
As shown in Box 18–1, the interaction of multiple neurotransmitters at a particular site in a neuronal tract enables the complex interplay of various neuronal systems and contributes to the wide range of functional expression exhibited in the CNS. For example, inhibition of the release of an inhibitory neurotransmitter will actually increase neurotransmission in the target neuron. Similarly, drugs can act in complex ways to affect neurotransmission. Ethanol (ethyl alcohol), for instance, can diminish the inhibitory influence of the cerebral cortex on certain human behaviors and thereby increase drug-induced behaviors, a phenomenon called behavioral disinhibition. Ethanol and other CNS depressants, initially or at low doses, exert their effects on the smaller and more numerous inhibitory neurons, creating disinhibition via excitation due to removal of inhibitory neurotransmitters. At higher doses, larger excitatory neurons are inhibited and profound depression of CNS activity can occur.
BOX 18–1 PATTERNS OF NEUROTRANSMISSION IN THE CENTRAL NERVOUS SYSTEM
A, B, C, D, E, and F are neurons in a tract that projects from left to right. Neurons B, D, and E release excitatory neurotransmitters (+). Neurons A and F release inhibitory neurotransmitters (−). The net effect of neuronal interactions on neurotransmission from B to C is shown in the table below. Each interaction presupposes that the other neurons are quiescent at that time. Other, more complex interactions are possible.
Interaction | Effect on Neurotransmission (B → C) |
---|---|
A → B | Decreased |
D → A → B | Greatly decreased |
F → A → B | Increased |
E → B | Increased |
Fast versus Slow Signals
Neurotransmitters in the CNS can be characterized as slow or fast, depending on the receptors that are activated and the persistence of signal transduction pathways. The best examples of fast neurotransmitters are γ-aminobutyric acid (GABA) and glutamate acting at ligand-gated ion channels. Binding of these amino acid neurotransmitters directly to subunits of the ion channel protein directly initiates ion flow with a signal that lasts for only a few milliseconds. Examples of slow neurotransmitters are norepinephrine and serotonin acting at G protein–coupled receptors. These activated G protein–coupled receptor proteins initiate a slower, multistep process with alterations in second messengers and membrane effects that can last from many milliseconds to as long as a second. A slow (long-acting) signal can influence the overall tone of a neuron because it can modulate the signals of several other fast neurotransmitters acting on the same neuron. For this reason, slow neurotransmitters can also be called neuromodulators.
Neurotransmitters and Receptors
Important neurotransmitters in the CNS include acetylcholine and several amino acids, biogenic amines, and neuropeptides. Table 18–1 lists the names, receptors, mechanisms of signal transduction, and functions of the major neurotransmitters.
The receptors can be divided into two basic groups: ionotropic receptors, also called ligand-gated ion channels, which are directly associated with ion channels, and metabotropic receptors, which are typical G protein–coupled receptors (see Chapter 3). Although this terminology is most frequently applied to receptors for amino acid neurotransmitters (e.g., GABA and glutamate), it is equally appropriate for other classes of neurotransmitter receptors.
The mechanisms of signal transduction for neurotransmitters in the CNS are similar to those for neurotransmitters in the autonomic nervous system. The activation of ionotropic receptors alters chloride, sodium, potassium, or calcium influx and thereby evokes excitatory or inhibitory membrane potentials. The linkage of metabotropic receptors with G proteins leads to activation or inhibition of adenylyl cyclase and alteration in the levels of intracellular cAMP, or activation of phospholipase C and the formation of inositol triphosphate and diacylglycerol. Metabotropic receptor activity can also modulate ion channel activity via second messengers (most notably calcium) that activate protein kinases responsible for the phosphorylation of ion channels. Signal transduction for other receptors in the CNS is discussed in Chapter 3.
Acetylcholine
Acetylcholine, synthesized from acetyl coenzyme A and choline, is degraded to acetate and choline by the enzyme acetylcholinesterase (Fig. 18–2). Acetylcholine receptors (also known as cholinergic receptors) consist of two main types: muscarinic receptors and nicotinic receptors. The properties and mechanisms of these receptors are compared in Table 6–1.
Drugs can affect acetylcholine neurotransmission by activating or blocking acetylcholine receptors or by inhibiting cholinesterase. The general pharmacologic properties of acetylcholine receptor agonists and antagonists are described in Chapters 6 and 7, respectively.
In the CNS, acetylcholine acts as an excitatory or inhibitory neurotransmitter in a number of neuronal tracts, including those that innervate the hippocampus, cerebral cortex, and basal ganglia. These tracts participate in memory, sensory processing, and motor coordination, respectively.
Amino Acids
Several amino acids are important neurotransmitters in the brain and spinal cord. Some of them, most notably, GABA and glycine, are inhibitory. Others, such as glutamate and aspartate, are excitatory.
γ-AMINOBUTYRIC ACID
GABA, which is synthesized from glutamic acid, is the most ubiquitous inhibitory neurotransmitter in the brain and spinal cord. Its receptors are the ionotropic GABAA receptors and the metabotropic GABAB receptors. Most drugs affecting GABA neurotransmission primarily activate or inhibit the GABAA-chloride ion channel complex. This ion channel complex contains receptors for several types of drugs, including the benzodiazepines and barbiturates (see Chapter 19), general anesthetics (Chapter 21), and alcohol (Chapter 25). Drugs acting at the GABAB receptor are used to control spasticity (Chapter 24). The functions of GABA include regulation of neuronal excitability throughout the CNS and motor coordination.
GLYCINE AND TAURINE
Glycine is a major inhibitory transmitter in the spinal cord. Its strychnine-sensitive receptors are coupled with the chloride ion channel, and activation of these receptors leads to membrane hyperpolarization. The inhibitory actions of glycine are potently antagonized by the alkaloid strychnine, a convulsant poison used as a rodenticide. Strychnine poisoning causes disinhibition of motoneurons, leading to convulsions and death through respiratory failure. Glycine also acts as a co-agonist to the excitatory amino acid, glutamate, at NMDA receptors, by binding to an allosteric strychnine-insensitive site.
Taurine is a sulfur-containing amino acid that is postulated to act as a neurotransmitter or neuromodulator in the CNS. Taurine is believed to activate both strychnine-sensitive and strychnine-insensitive types of glycine-binding sites.
GLUTAMATE AND ASPARTATE
Glutamate and aspartate are acidic amino acids that function as excitatory neurotransmitters throughout the CNS. Their ionotropic receptors consist of three types that differ in their subunit composition and are named for drugs that show the most selectivity for each type: NMDA (n-methyl-d-aspartate), AMPA (α-amino-3-hydroxy-5-methyl-4-isoxazole propionate), and kainate receptors. These receptors are excitatory because their associated ion channels allow the flow of sodium or calcium ions into neurons causing depolarization. Metabotropic glutamate receptors consist of two main classes that are either coupled to phospholipase C and intracellular calcium signaling or are negatively coupled to adenylyl cyclase. Glutamate and aspartate participate in the long-term potentiation needed for learning and memory and have a role in neuronal toxicity and apoptosis (cell death) evoked by trauma and ischemia. Antagonists of these excitatory amino acid receptors are used in the treatment of seizures (Chapter 20) and may find use in blocking the overexcitation of neurons that occurs following stroke and other disorders.
Biogenic Amines
The biogenic amines or monoamines that function as CNS neurotransmitters are the catecholamines, dopamine and norepinephrine; and serotonin (5-hydroxytryptamine); and histamine. These neurotransmitters, which are formed by decarboxylation of amino acids, are catabolized in part by the enzyme, monoamine oxidase.
DOPAMINE
Dopamine is a major CNS neurotransmitter that binds to five types of dopamine receptors. The D1 and D5 receptors activate adenylyl cyclase and thereby increase cAMP levels. In contrast, the D2, D3, and D4 receptors inhibit adenylyl cyclase and decrease cAMP levels. As shown in Table 18–2, levodopa, clozapine, and other drugs have effects on various steps in the synthesis and metabolism of dopamine. Dopamine is found in several neuronal tracts originating from the ventral tegmental area, plays a significant role in behavioral and drug reinforcement, and regulates emesis (vomiting), prolactin release, mood states, motor coordination, and olfaction. Its degradation by monoamine oxidase results in the formation of homovanillic acid, a metabolite that is subsequently excreted in the urine (Fig. 18–3). Inhibitors of monoamine oxidase increase dopamine levels in the brain and are used for the treatment of Parkinsonism.
NOREPINEPHRINE
Norepinephrine, which is formed from dopamine, is degraded by monoamine oxidase and catechol-O-methyltransferase to a number of metabolites. The major metabolite excreted in the urine is 3-methoxy-4-hydroxy-mandelic acid, or vanillylmandelic acid. The receptors for norepinephrine are α-adrenoceptors and β-adrenoceptors (also known as α- and β-adrenergic receptors), and their classification and properties are described in detail in Chapter 8. Norepinephrine is associated with several neuronal tracts projecting from the locus ceruleus in the medulla (brainstem) to the thalamus, cerebral cortex, cerebellum, and spinal cord, and it is found in tracts projecting from the midbrain to the hypothalamus. This ubiquitous neurotransmitter participates in the regulation of anxiety, cerebellar function, learning, memory, mood, sensory processing (including pain), and sleep. Drugs can alter norepinephrine neurotransmission by activating or blocking its receptors or by inhibiting its presynaptic neuronal uptake.
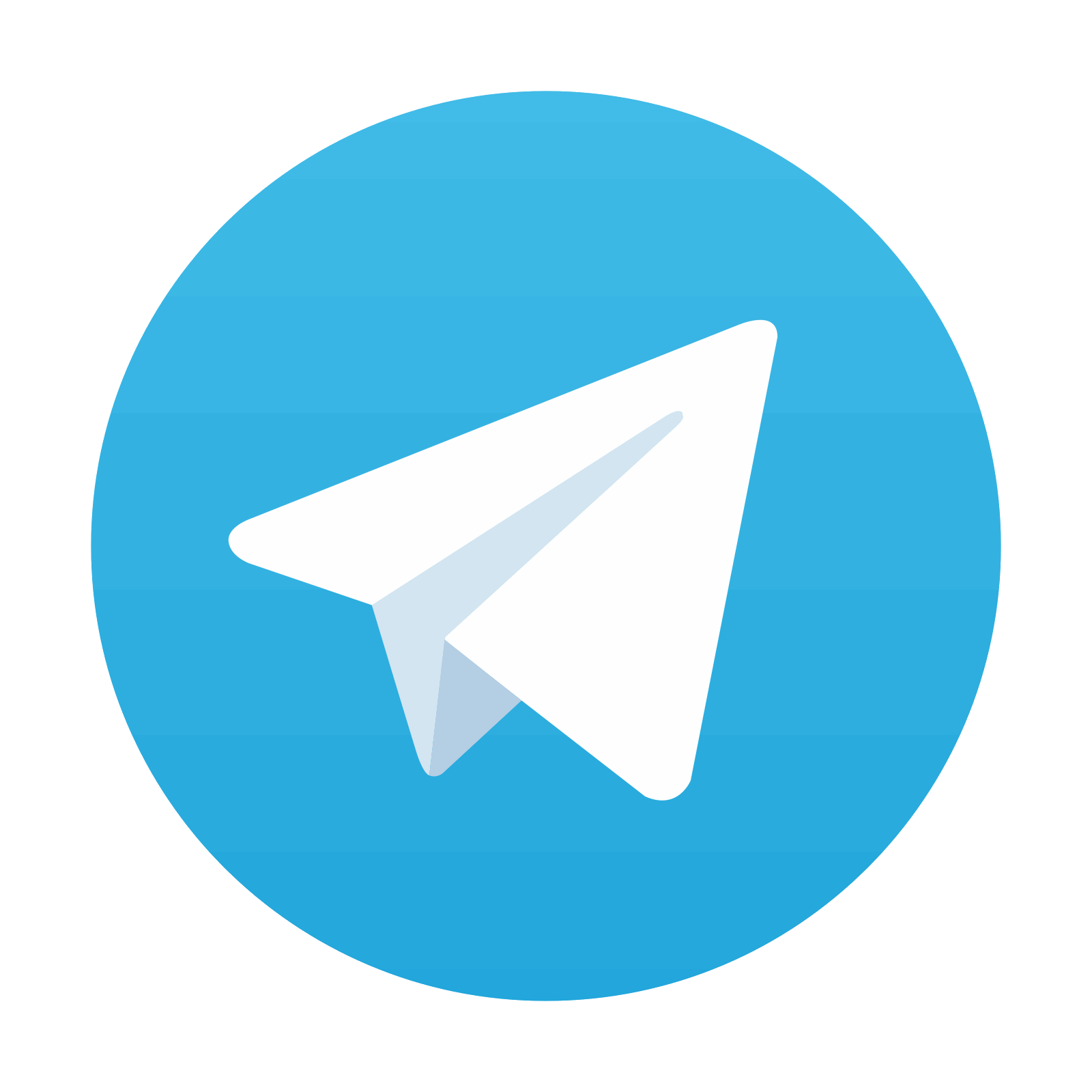
Stay updated, free articles. Join our Telegram channel

Full access? Get Clinical Tree
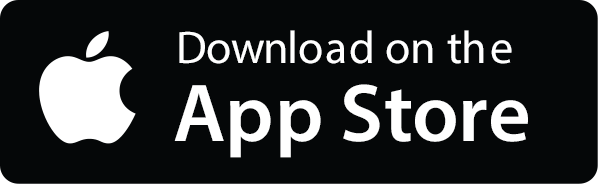
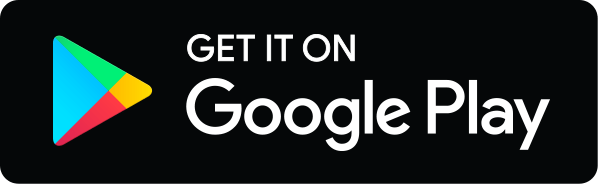