Figure 8–1 Primary tissue locations of α-adrenoceptors and β-adrenoceptors. Whereas α1– and β2-adrenoceptors are primarily located in smooth muscle, β1-adrenoceptors are predominantly found in cardiac tissue. Some α2-adrenoceptors are located on sympathetic neurons, where they produce feedback inhibition of neurotransmitter release. Other α2– and β2-adrenoceptors are located in blood platelets and a variety of organ tissues. The α- and β-adrenoceptors are also found in the central nervous system.
α-Adrenoceptors
The α-adrenoceptors can be differentiated on the basis of their location and function. The α1-adrenoceptors are primarily located in smooth muscle at sympathetic neuroeffector junctions, but these receptors are also found in exocrine glands and the central nervous system. Three subtypes of α1-adrenoceptors have been identified (α1A, α1B, and α1D), but the functional roles of these α1-receptor subtypes have not been clearly established. The α2-adrenoceptors are widely distributed in presynaptic neurons, various tissues, and blood platelets (see Fig. 8–1), and subtypes of these receptors have also been identified.
The α1-adrenoceptors mediate contraction of vascular smooth muscle, the iris dilator muscle, and smooth muscle in the lower urinary tract (bladder, urethra, and prostate). The α2-adrenoceptors located on sympathetic postganglionic neurons serve as autoreceptors whose activation leads to feedback inhibition of norepinephrine release from nerve terminals. The α2-receptors are also found in blood platelets and in ocular, adipose, intestinal, hepatic, renal, and endocrine tissue. In blood platelets, α2-receptors mediate platelet aggregation. In the pancreas, α2-receptors mediate the inhibition of insulin secretion that occurs when the sympathetic nervous system is activated.
β-Adrenoceptors
Table 8–1 summarizes the effects of three subtypes of β-adrenoceptors.
Activation of β1-adrenoceptors produces cardiac stimulation, leading to a positive chronotropic effect (increased heart rate), a positive inotropic effect (increased contractility), and a positive dromotropic effect (increased cardiac impulse conduction velocity). Activation of β1 receptors also increases renin secretion from renal juxtaglomerular cells.
The β2-adrenoceptors mediate relaxation of bronchial, uterine, and vascular smooth muscle (see Fig. 11–3). In skeletal muscle, β2-receptors mediate potassium uptake. In the liver, they mediate glycogenolysis, which increases the glucose concentration in the blood. Whereas epinephrine and norepinephrine are equally potent at β1-receptors in cardiac tissue, epinephrine is more potent than norepinephrine at β2-receptors in smooth muscle.
Activation of β3-adrenoceptors produces lipolysis, a process in which the hydrolysis of triglycerides in adipose tissue leads to the release of fatty acids into the circulation. Investigators are currently searching for selective β3-adrenoceptor agonists because they believe that the lipolytic effect of these agonists would be useful in the treatment of obesity.
Dopamine Receptors
Dopamine receptors are activated by dopamine but not by other adrenoceptor agonists. Several subtypes exist, including the D1-receptors, which mediate muscle relaxation in vascular smooth muscle, and the D2-receptors, which modulate neurotransmitter release.
Imidazoline Receptors
Imidazoline receptors are activated by adrenoceptor agonists and other substances that contain an imidazoline structure. One of the best-studied examples of these substances is clonidine. The imidazoline receptors are found in the central nervous system and a number of peripheral tissues. The antihypertensive effect of clonidine appears to result from activation of both α2-adrenoceptors and imidazoline receptors in the central nervous system, leading to a reduced sympathetic outflow to the heart and vascular smooth muscle. The pharmacologic properties of antihypertensive imidazoline drugs are discussed in greater detail in Chapter 10.
Signal Transduction
The adrenergic, dopamine, and imidazoline receptors are guanine nucleotide protein–binding (G protein–binding) receptors that are located in cell membranes of target tissues.
Activation of α1-adrenoceptors is coupled with activation of phospholipase C, which catalyzes the release of inositol triphosphate (IP3) from membrane phospholipids. In smooth muscle, IP3 stimulates the release of calcium from the sarcoplasmic reticulum, and this leads to muscle contraction. This is the mechanism by which α1-adrenoceptor agonists cause vasoconstriction and increase blood pressure. In exocrine glands, formation of IP3 leads to calcium release and gland secretion. Activation of α2-adrenoceptors leads to inhibition of adenylyl cyclase and a decrease in the levels of cyclic adenosine monophosphate (cAMP) in sympathetic neurons and other tissues. This is the mechanism responsible for a decrease in aqueous humor secretion and for other effects of α2-adrenoceptor agonists. Activation of D2-receptors also reduces cAMP formation.
Activation of β-adrenoceptors and D1-receptors leads to stimulation of adenylyl cyclase and an increase in the levels of cAMP in cardiac tissue and smooth muscle. Cyclic AMP activates protein kinase A, which phosphorylates other proteins and enzymes. The cellular response depends on the specific proteins that are phosphorylated in each tissue. In cardiac tissue, calcium channels are phosphorylated, thereby augmenting calcium influx and cardiac contractility. In smooth muscle, cAMP produces muscle relaxation via effects on multiple targets, including potassium channels, calcium channels, and myosin light chain kinase (see Fig. 11–3).
Classification of Adrenoceptor Agonists
The adrenoceptor agonists mimic the effect of sympathetic nervous system stimulation and, therefore, are also called sympathomimetic drugs. These drugs can be divided into three groups on the basis of their mode of action. The direct-acting agonists bind to and activate adrenoceptors. As shown in Figure 8–2, the indirect-acting agonists increase the stimulation of adrenoceptors by increasing the concentration of norepinephrine at sympathetic neuroeffector junctions in one of two ways. Cocaine inhibits the catecholamine transporter located in the plasma membrane of the presynaptic sympathetic neuron and thereby decreases the neuronal reuptake of norepinephrine and increases its synaptic concentration. Amphetamine and related drugs are transported into the sympathetic nerve terminal by the catecholamine transporter. Once inside the sympathetic neuron, amphetamines inhibit the storage of norepinephrine by neuronal vesicles. This increases the cytoplasmic concentration of norepinephrine, leading to reverse norepinephrine transport into the synapse by the catecholamine transporter. The mixed-acting agonists (e.g., ephedrine) have both direct and indirect actions.
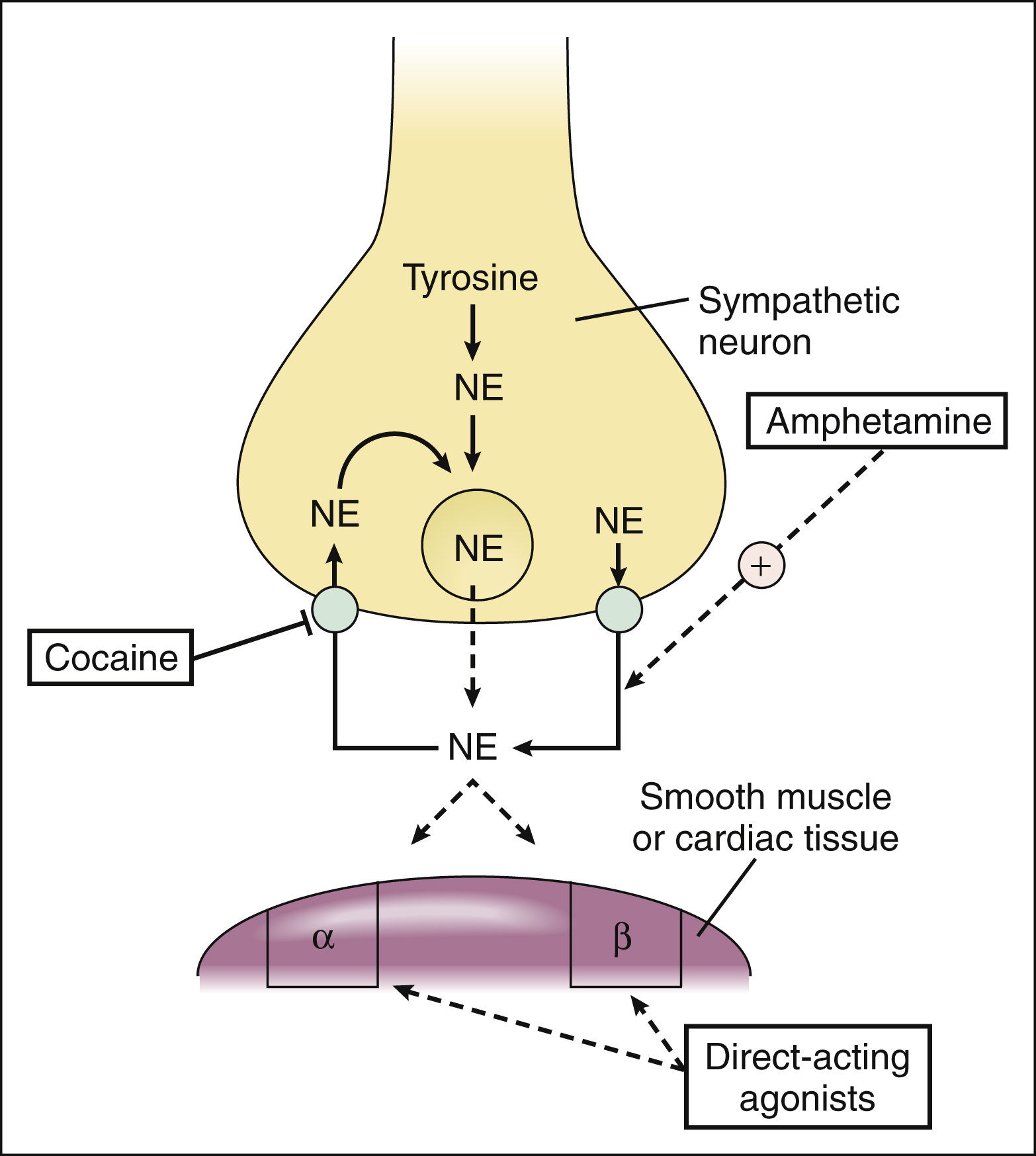
Figure 8–2 Mechanisms of indirect-acting and direct-acting adrenoceptor agonists. Cocaine blocks norepinephrine reuptake by the catecholamine transporter. Amphetamine inhibits the storage of norepinephrine by neuronal vesicles, leading to reverse transport of norepinephrine into the synapse by the catecholamine transporter. Direct-acting agonists bind to and activate adrenoceptors. α = α-adrenoceptor; β = β-adrenoceptor; NE = norepinephrine.
DIRECT-ACTING ADRENOCEPTOR AGONISTS
The direct-acting agonists can be subdivided into catecholamines and noncatecholamines.
Catecholamines
The naturally occurring catecholamines include norepinephrine, an endogenous sympathetic neurotransmitter; epinephrine, the principal hormone of the adrenal medulla; and dopamine, the precursor to norepinephrine and epinephrine. Synthetic catecholamines include isoproterenol and dobutamine.
General Properties
CHEMISTRY AND PHARMACOKINETICS
Each catecholamine consists of the catechol moiety and an ethylamine side chain (Fig. 8–3). The catecholamines are rapidly inactivated by monoamine oxidase (MAO) and catechol-O-methyltransferase (COMT), enzymes found in the gut, liver, and other tissues. The drugs have low oral bioavailabilities and short plasma half-lives. For these reasons, they must be administered parenterally when a systemic action is required (e.g., in the treatment of patients who are in anaphylactic shock).
MECHANISMS AND EFFECTS
As shown in Figure 8–3 and Table 8–2, the various catecholamines differ in their affinities and specificities for receptors. The size of the alkyl substitution on the amine nitrogen (R2) determines the relative affinity for α- and β-adrenoceptors. Drugs with a large alkyl group (e.g., isoproterenol) have greater affinity for β-adrenoceptors than do drugs with a small alkyl group (e.g., epinephrine). Epinephrine is a potent agonist at all α- and β-adrenoceptors. Norepinephrine differs from epinephrine only in that it has greater affinity for β1-adrenoceptors than for β2-adrenoceptors. Because of this difference, norepinephrine constricts all blood vessels, whereas epinephrine constricts some blood vessels but dilates others. Isoproterenol is considered to be a selective β1– and β2-adrenoceptor agonist because it has little affinity for α-receptors. Dobutamine primarily stimulates β1-receptors but has minor stimulatory effects on β2– and α-receptors. Dopamine activates D1-, β1-, and α-receptors. Unlike the other catecholamines, dopamine also stimulates the release of norepinephrine from sympathetic neurons. For this reason, dopamine is both a direct-acting and an indirect-acting receptor agonist.
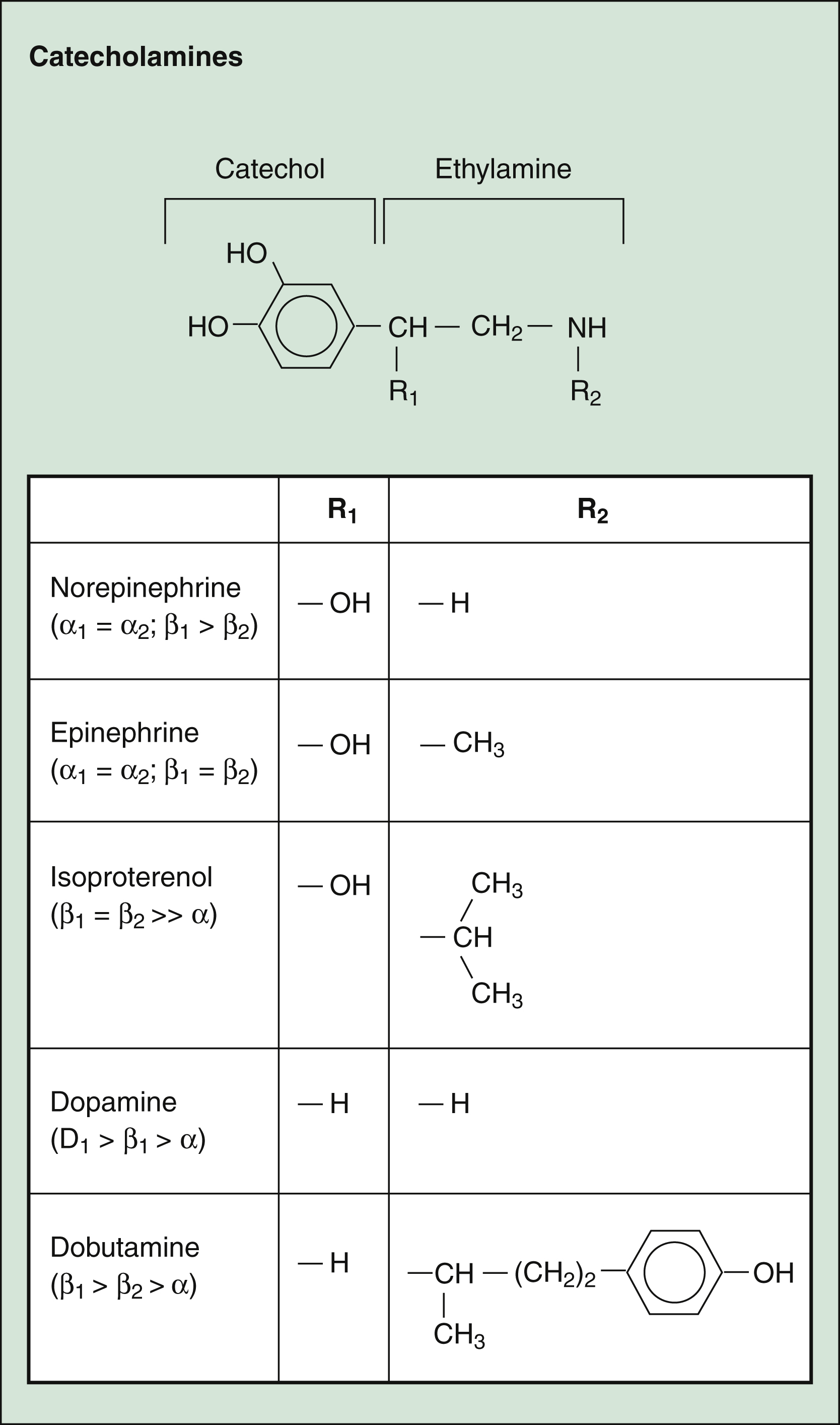
Figure 8–3 Structures of selected adrenoceptor agonists. Catecholamines contain the catechol moiety and an ethylamine side chain. The amine nitrogen substitution (R2) determines the relative affinity for α- and β-adrenoceptors, with larger substitutions (e.g., in isoproterenol and dobutamine) decreasing the affinity for α-adrenoceptors and increasing the affinity for β-adrenoceptors. Note that dopamine has an even higher affinity for dopamine D1 receptors than for adrenoceptors.
TABLE 8–2 Pharmacologic Effects and Clinical Uses of Adrenoceptor Agonists
Drug | Pharmacologic Effect (and Receptor) | Clinical Use |
---|---|---|
Direct-Acting Catecholamines | ||
Dobutamine | Cardiac stimulation (β1) and vasodilation (β2) | Cardiogenic shock, acute heart failure, and cardiac stimulation during heart surgery |
Dopamine∗ | Renal vasodilation (D1), cardiac stimulation (β1), and increased blood pressure (β1 and α1) | Cardiogenic shock, septic shock, heart failure, and adjunct to fluid administration in hypovolemic shock |
Epinephrine | Vasoconstriction and increased blood pressure (α1), cardiac stimulation (β1), and bronchodilation (β2) | Anaphylactic shock, cardiac arrest, ventricular fibrillation, reduction in bleeding during surgery, and prolongation of the action of local anesthetics |
Isoproterenol | Cardiac stimulation (β1) and bronchodilation (β2) | Asthma, atrioventricular block, and bradycardia |
Norepinephrine | Vasoconstriction and increased blood pressure (α1) | Hypotension and shock |
Direct-Acting Noncatecholamines | ||
Albuterol | Bronchodilation (β2) | Asthma |
Apraclonidine | Decreased aqueous humor formation (α2) | Short-term control of intraocular pressure |
Clonidine | Decreased sympathetic outflow from central nervous system (α2 and imidazoline) | Hypertension, opioid dependence |
Dexmedetomidine | Sedation (α2) | Adjunct to anesthesia |
Midodrine | Vasoconstriction (α1) | Orthostatic hypotension |
Oxymetazoline | Vasoconstriction (α1) | Nasal and ocular decongestion |
Phenylephrine | Vasoconstriction, increased blood pressure, and mydriasis (α1) | Nasal and ocular decongestion, mydriasis, maintenance of blood pressure and treatment of shock |
Ritodrine | Uterine relaxation (β2) | Premature labor |
Terbutaline | Bronchodilation (β2) | Asthma |
Indirect-Acting Agents | ||
Amphetamine | Increase in norepinephrine release, central nervous system stimulation | Narcolepsy, attention-deficit disorder |
Cocaine | Inhibition of norepinephrine uptake | Local anesthesia |
Mixed-Acting Agents | ||
Ephedrine | Vasoconstriction (α1) | Nasal decongestion |
Pseudoephedrine | Vasoconstriction (α1) | Nasal decongestion |
∗ Dopamine is a catecholamine with mixed action.
(1) CARDIOVASCULAR EFFECTS. Figure 8–4 compares the cardiovascular effects when norepinephrine, epinephrine, isoproterenol, and dopamine are given by intravenous infusion.
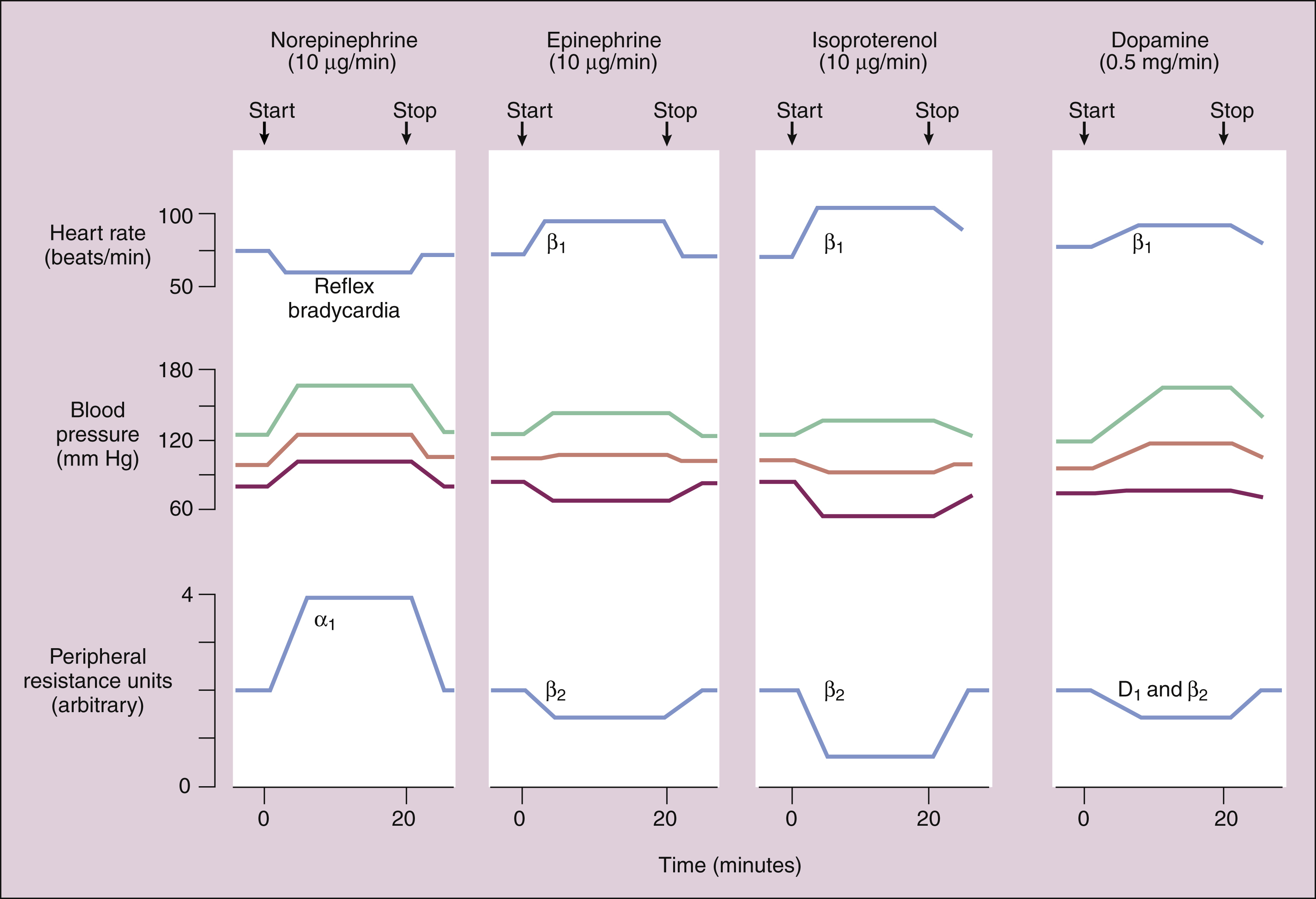
Figure 8–4 Comparison of the cardiovascular effects of four catecholamines when a low dose of each drug is given by intravenous infusion. Arrows indicate when the infusion was started and stopped. The blood pressure recordings show systolic, diastolic, and mean arterial pressure. Peripheral resistance is expressed on an arbitrary scale, ranging from 0 to 4 units. The reflex mechanism, adrenoceptors (α1, β1, and β2), or dopamine (D1) receptors responsible for changes in the heart rate and peripheral resistance are illustrated. Norepinephrine increases peripheral resistance and blood pressure, and this leads to reflex bradycardia. Epinephrine increases heart rate while reducing peripheral resistance, and the mean arterial blood pressure increases slightly. Isoproterenol increases heart rate but significantly lowers peripheral resistance, and the mean arterial pressure declines. Dopamine increases heart rate (and increases cardiac output) while lowering vascular resistance, and the mean arterial pressure increases.
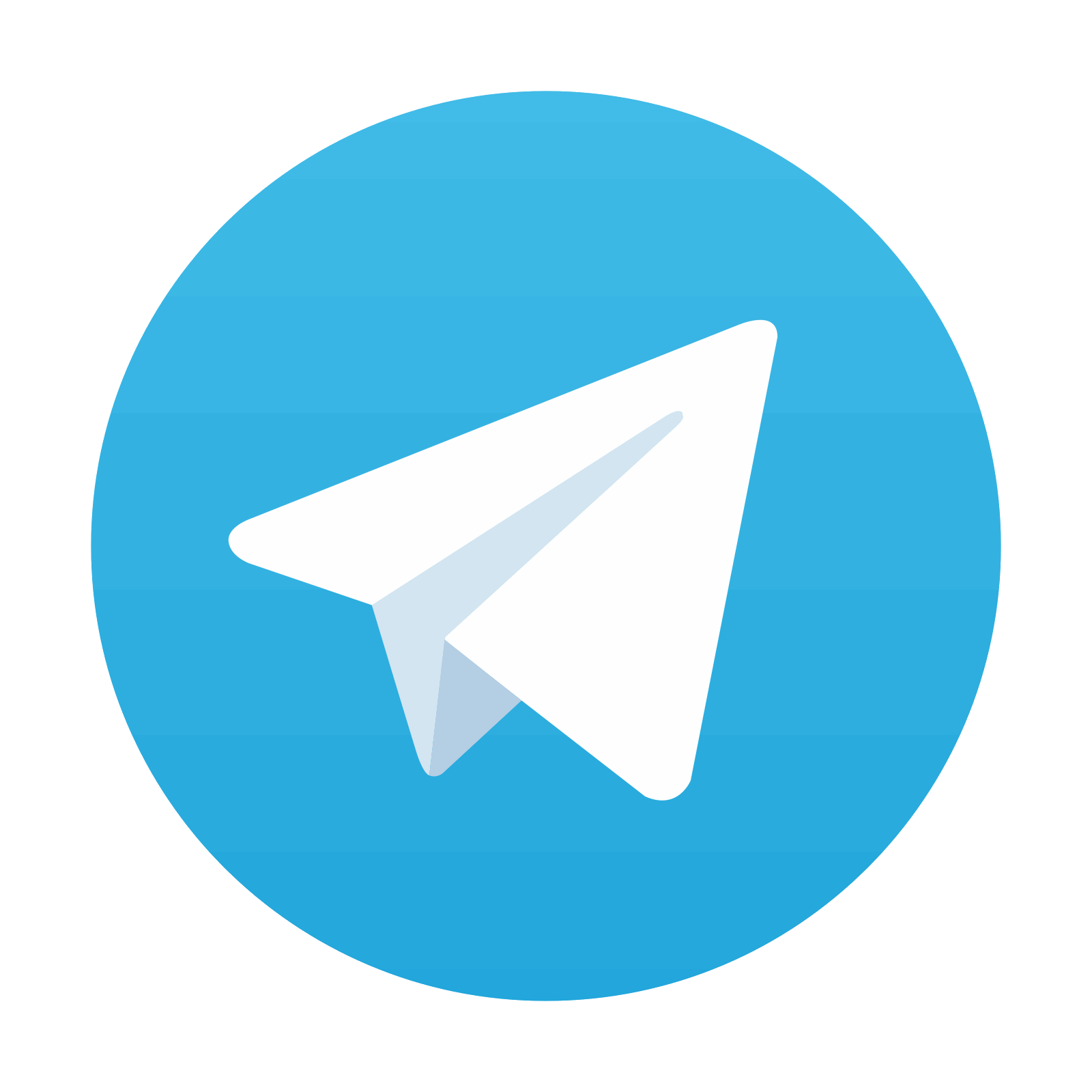
Stay updated, free articles. Join our Telegram channel

Full access? Get Clinical Tree
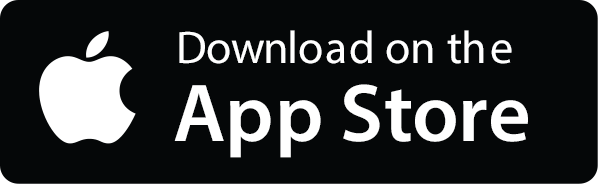
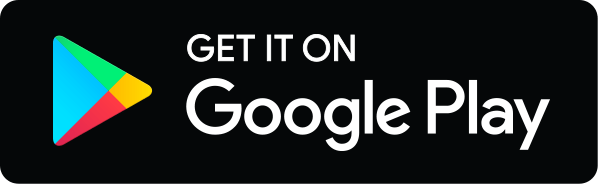
