Virus Entry and Uncoating
Ari Helenius
Viral particles have a single mission: to transport the viral genome from an infected host cell to a noninfected host cell and to deliver it into the cytoplasm or the nucleus in a replication-competent form. The target can be a neighboring cell, a cell elsewhere in the host organism, or a cell in another organism. The process starts in an infected cell with the packaging of the viral genome and accessory proteins into a new virus particle, which is released into the extracellular space. When the virus contacts the surface of a new host cell, a complex series of events ensues tightly coordinated in time and space. These events include binding to receptors and signaling, often followed by endocytic internalization, vesicular trafficking, membrane penetration, cytosolic transport, and nuclear import (Fig. 4.1). Uncoating is an integral part of the process; the virus particle is modified, destabilized, disassembled, and eventually the genome, present in a protected and condensed form in the virion, is decondensed and exposed in a replication- or transcription-competent form. The progression of a virus particle through its entry program depends critically on cellular functions. The Trojan horse strategy that is used is necessary because the particles are simple and capable of limited independent functions.
This chapter describes some of the general concepts that govern cellular entry of animal viruses. For information about the entry of specific viruses and virus families, the reader is referred to the virus chapters. Information relevant to the topics covered here also can be found in numerous reviews that cover early virus cell interactions.43,81,100,109,110,124,150,167,212
The Barriers
The first barrier that incoming viruses must overcome is the glycocalyx, a layer of glycoconjugates that covers the external surface of cells. It is composed of glycoproteins, glycolipids, and proteoglycans. The composition and thickness of this layer is variable. By binding to oligosaccharides, many viruses make use of the glycocalyx for initial attachment.
The next barrier is the plasma membrane. Responsible for the cell’s exchanges with the environment, it is the most complex and most dynamic of all cell membranes. The composition and properties are regulated by the endocytic and secretory pathways and by a continuous association and disassociation of proteins that interact with the cytosolic leaflet. The plasma membrane is a highly sensitive organ for recognizing and responding to external stimuli. Viruses take advantage of this during entry.
After clearing the plasma membrane by direct penetration or by exploiting endocytic pathways, viruses and viral capsids have to reach sites deeper in the cytoplasm. The cortical actin network underneath the plasma membrane and extreme crowding constitute major barriers to movement within the cytoplasm.184 Finally, because many viruses replicate in the nucleus, the genome and accessory proteins must travel to the nucleus and cross the nuclear envelope. This requires cooperation between the incoming virus and the nuclear import machinery.211
Virus Binding to the Cell Surface
Viruses can only infect cells to which they can bind. Binding occurs to attachment factors and virus receptors on the surface of the cell. To a large extent, the identity, distribution, and behavior of these cellular components determine which cell types, tissues, and organisms a virus can infect. The receptors also define, in part, the pathogenic potential of a virus as well the nature of the disease that it causes.
Virus receptors can be defined as cell surface molecules that bind the incoming viruses to the cell, and, in addition, promote entry by (a) inducing conformational changes in the virus that lead to priming, association with other receptors, membrane fusion, and penetration; (b) transmitting signals through the plasma membrane that lead to virus uptake or penetration and prepare the cell for the invasion; or (c) guiding bound virus particles into a variety of endocytic pathways.124 Attachment factors help to concentrate the particles on the cell’s
surface, thus enhancing entry and infection. Unlike receptors, however, they do not actively promote entry and mediate signals. Often, the interactions with attachment factors are not highly specific. In practice, the distinction between a receptor and an attachment factor is not always straightforward because the consequences of virus binding to a given surface component are difficult to assess experimentally and can vary depending on cell type and conditions.
surface, thus enhancing entry and infection. Unlike receptors, however, they do not actively promote entry and mediate signals. Often, the interactions with attachment factors are not highly specific. In practice, the distinction between a receptor and an attachment factor is not always straightforward because the consequences of virus binding to a given surface component are difficult to assess experimentally and can vary depending on cell type and conditions.
Receptors and attachment factors constitute a diverse collection of proteins, carbohydrates, and lipids with physiologic functions unrelated to pathogen interaction. Ranging from abundant and ubiquitous to rare and species specific, they differ from one virus to the next. In the past few decades, an impressive number have been identified in different virus and host cell systems. As shown in Table 4.1, which gives a partial list, receptors include ligand-binding receptors, glycoproteins, ion channels, gangliosides, carbohydrates, proteoglycans, and so on. Some families of surface molecules seem to be favored; the immunoglobulin-G superfamily of transmembrane proteins, proteoglycans, and glycoconjugates with terminal sialic acid residues, belong to these. In addition, a large group of viruses use integrins as their receptors.190
Many viruses use multiple attachment factors and receptors. They interact with them in parallel or in series, or they use different receptors for entry into different cell types. When multiple receptors are required for productive entry, it is the convention to call the one contacted first a receptor and the subsequent ones coreceptors. A good example is human immunodeficiency virus type 1 (HIV-1), which makes use of heparan sulfate proteoglycans as attachment factors, CD4 as a receptor,25 and CXCR4 and CCR5 (or a related chemokine receptor) as coreceptors.13,127 In this case, the two receptors are used to induce stepwise conformational changes in the spike glycoprotein. In other cases (e.g., adenovirus 2 and coxsackie B virus), two receptors seem to be needed to overcome anatomic and topological barriers.39,131 Herpes viruses are able to infect a variety of cell types, probably in part because they possess proteins that can bind to several different receptors.185 Cases are also seen where unrelated viruses make use of the same receptors. One well-studied example is coxsackie and adenovirus receptor (CAR) (Table 4.1), which is used as a receptor both by adenovirus 2 and 5 and coxsackie B viruses.219
The interaction between a viral surface protein and a receptor can be highly specific, but the affinity is often low. However, the presence of multiple, closely spaced binding sites on the surface of the virus particle allows multivalent binding, and the avidity is therefore frequently high. The affinity of influenza hemagglutinin for sialic acid containing glycoconjugates is, for example, in the millimolar range, but virus binding to cells is virtually irreversible.180 That most receptor molecules are laterally mobile in the plasma membrane allows, moreover, the formation of a local microdomain rich in receptors under the bound virus with a composition and properties different from that in the surrounding membrane.52 The consequences can be the inclusion of the virus in lipid rafts, or entrapment in caveolae, coated pits, and other membrane specializations. Receptor clustering can also lead to transmembrane signaling, changes in the actin cytoskeleton, and recruitment of cytosolic factors such as a clathrin coat to the plasma membrane.
Although the interaction between a virus and its receptors is generally direct, there are cases where adaptor proteins are involved. For example, binding of antibodies to dengue virus allows infection of macrophages via Fc receptors on the surface of these cells.143 In this case, the virus particle is internalized as an immune complex. Instead of neutralizing the virus, the antibodies mediate expansion of the host cell repertoire.
In enveloped viruses, the spike glycoproteins are responsible for receptor binding. Typically, these are oligomeric type 1 integral membrane proteins that have the bulk of their mass outside the membrane with the receptor-binding domain exposed. Other external domains in the same protein may be responsible for membrane fusion and receptor destruction. In some spike proteins (e.g., the HIV-1 glycoprotein), the receptor-binding moiety is not covalently connected to the rest, which allows its dissociation
once receptor interaction has occurred. X-ray crystal structures of spike glycoprotein–receptor complexes exist for several enveloped viruses (see Chapter 3).
once receptor interaction has occurred. X-ray crystal structures of spike glycoprotein–receptor complexes exist for several enveloped viruses (see Chapter 3).
Table 4.1 Receptor Proteins for Some Viruses | ||||||||||||||||||||||||||||||||||||||||||||||||||||||||||||||||||||||||||||||||||||||||||||||||||||||||||||||||||||||||||||||||||||||||||||||||||||||||||||||||||||||||||||||||||||||||||||||||||||||||||||||||||||||||||||
---|---|---|---|---|---|---|---|---|---|---|---|---|---|---|---|---|---|---|---|---|---|---|---|---|---|---|---|---|---|---|---|---|---|---|---|---|---|---|---|---|---|---|---|---|---|---|---|---|---|---|---|---|---|---|---|---|---|---|---|---|---|---|---|---|---|---|---|---|---|---|---|---|---|---|---|---|---|---|---|---|---|---|---|---|---|---|---|---|---|---|---|---|---|---|---|---|---|---|---|---|---|---|---|---|---|---|---|---|---|---|---|---|---|---|---|---|---|---|---|---|---|---|---|---|---|---|---|---|---|---|---|---|---|---|---|---|---|---|---|---|---|---|---|---|---|---|---|---|---|---|---|---|---|---|---|---|---|---|---|---|---|---|---|---|---|---|---|---|---|---|---|---|---|---|---|---|---|---|---|---|---|---|---|---|---|---|---|---|---|---|---|---|---|---|---|---|---|---|---|---|---|---|---|---|---|---|---|---|---|---|---|---|---|---|---|---|---|---|---|---|
|
Table 4.2 pH-Dependence of Virus Families | ||||||||||||||||||||||||||||||||||||
---|---|---|---|---|---|---|---|---|---|---|---|---|---|---|---|---|---|---|---|---|---|---|---|---|---|---|---|---|---|---|---|---|---|---|---|---|
|
In nonenveloped viruses, the structures that bind receptors are projections or indentations in the capsid surface. Adenoviruses have trimeric fiber proteins with globular knobs that project from the vertices.15 The penton base protein of many adenovirus subfamilies contains in addition an exposed Arg-Gly-Asp (RGD) sequence that associates with integrins.189 Many enterovirus receptors bind in a cleft in the capsid surface called the canyon, the molecular features of which have been analyzed in great detail.161
Role of Carbohydrates
Glycoconjugates on the cell surface have an important role during entry of many viruses as receptors and attachment factors. Glycoproteins and glycolipids, with terminal sialic-acid residues, serve as specific receptors for a variety of viruses, including orthomyxo-, paramyxo-, and polyoma viruses. The HA1 subunits of influenza A virus hemaglutinin (HA) bind terminal sialic acid residues associated with galactose through either a Neu5Ac α(2,3)-Gal or Neu5Ac α(2,6)-Gal bond.180 Human influenza recognizes the α(2,6) linkage; avian and equine viruses, the α(2,3) linkages, whereas porcine viruses appear to recognize both. These specificities reflect the structure of the glycans expressed in the different species and play a central role in limiting cross-species transmission. The tetrameric hemagglutinin-neuraminidase (HN) proteins of parainfluenza virus 5, has specificity for α(2,3)-sialyllactose,218 and polyomaviruses bind to specific saccharide residues in the glycan moieties of various gangliosides.201 A difference limited to a single atom in sialic acids plays a major role in species specificity of simian virus 40 (SV40), because it binds better to the simian GM1 ganglioside, which has a N-glycolylneuraminic acid, than to the human, which has a N-acetylneuraminic acid.23
The list of viruses recognized as binding to glycosaminoglycan (GAG) chains (e.g., heparan sulfate) is steadily growing.6 It now includes several herpes-, alpha-, flavi-, retro-, parvo-, picorna-, and papillomaviruses. Binding often involves positively charged patches in viral surface proteins. In some cases, viruses adopt GAGs as receptors when grown in tissue culture; their surface proteins mutate and express more basic residues.22,181 In contrast to tissue culture-adapted strains, natural isolates do not necessarily bind to heparan sulfate. Indeed, adaptation of different glycan receptors is likely part of the age-old war against pathogens, including viruses and their hosts, a war in which the diversity of surface carbohydrates plays an important role.
In most cases, it is the viruses that recognize host cell glycans. However, the reverse is true when cell surface lectins bind to glycans present in the envelope proteins of incoming viruses. One such lectin is DC-SIGN, a tetrameric, C-type lectin present on the surface of immature dendritic cells. It binds N-linked glycans of the high-mannose type,57 such as in glycans that have failed to undergo terminal glycosylation in the Golgi complex of the infected cells. Because glycoproteins synthesized in insect cells have exclusively high-mannose glycans, viruses introduced into the skin via insect bites are often recognized by DC-SIGN, resulting in the infection of dendritic cells. Viruses that bind to these lectins include HIV-1, Sindbis, human cytomegalovirus, dengue, and severe acute respiratory syndrome (SARS) viruses.66,91,103,149,178,197 Thus cells that our body uses in the front-line defense against pathogens end up serving the interests of viruses instead by spreading the infection.
Mobility of Cell-Associated Viruses
The encounter between individual viruses and the cell can be visualized live by light microscopy using fluorescent viruses. What happens depends on the virus, the receptor, and the host cell. Parvovirus particles undergo rapid binding and release events that eventually result in permanent attachment and endocytic internalization.171 Polyomavirus particles bind firmly and diffuse laterally in the membrane for 5 to 10 seconds, after which they are arrested in confinement zones defined by the cortical actin network and eventually internalized.54 Reoviruses do not show lateral motion after binding.50 In the case of a bunyavirus, Uukuniemi virus, the rapid clustering of receptor molecules (GFP-tagged DC-SIGN) can be seen to occur at the site of virus binding.102
Filopodia have been shown to play an active role by providing directed transport of surface-associated virus particles toward the cell body.96 They are thin, mobile extensions of the plasma membrane stabilized by an actin filament bundle. Such “virus surfing” occurs at a rate of 1 to 2 μm/min, mirroring the rate of retrograde actin flow from the tip of the filopodia inward.168 It is actin dependent and inhibited by inhibitors of myosin II. Although such motility of viruses is not essential for infection in tissue culture cells, it may play a role in tissues.
After endocytosis, the actin- and microtubule-dependent movement of intracellular vacuoles, viruses, and naked capsids inside the cell can also be visualized.50,77,95,164,207 This is illustrated by fluorescent influenza A viruses, which after a slow period of actin-restricted motion in the cell periphery, undergo rapid microtubule-mediated transport toward the perinuclear space where penetration by membrane fusion occurs.95,164 In
the case of adenovirus 2, the transport of capsids along microtubules is both plus- and minus-end directed, but net transport in the minus-end direction allows the virus to reach the nucleus.193 The entry of parvovirus adeno-associated virus 2 has been traced all the way to the nucleus, inside of which it moves unidirectionally along well-defined pathways.171
the case of adenovirus 2, the transport of capsids along microtubules is both plus- and minus-end directed, but net transport in the minus-end direction allows the virus to reach the nucleus.193 The entry of parvovirus adeno-associated virus 2 has been traced all the way to the nucleus, inside of which it moves unidirectionally along well-defined pathways.171
Virus-Induced Signals
Many viruses use the host cell’s signaling systems to promote entry and optimize infection.68,124,131 Viruses take advantage of the fact that cells are exquisitely sensitive to ligands that bind to the plasma membrane, particularly if they induce clustering of surface components. More specifically, signaling is used to trigger access to coreceptors, to induce endocytic responses, to reprogram endocytic pathways, and to induce favorable intracellular conditions for infection.
Signaling starts at the plasma membrane after binding of the virus to receptors and formation of receptor clusters. Depending on the virus, receptors, and host cells, initial binding can lead to activation of tyrosine or other kinases, which, in turn, trigger cascades of downstream responses at the plasma membrane, in the cytoplasm, and, in some cases, in the nucleus. Virus-induced signaling depends on the usual panel of second messengers (phosphatidylinositides, diacylglycerides, and calcium), and on numerous regulators of membrane trafficking and actin dynamics.
One well-studied case that demonstrates the complexity of virus-induced signaling is provided by adenoviruses 2 and 5, which use CAR and integrin αvβ3 as receptors.68,131 Endocytic internalization occurs via clathrin-coated vesicles, and penetration takes place in endosomes. The interaction with the integrin triggers activation of p85/p110, a PI(3) kinase. The synthesis of PI(3,4)P2 and PI(3,4,5)P3 activates protein kinase C. Small GTPases (e.g., Rab and Rho family members) are also activated. One of the downstream responses is the transient activation of macropinocytosis, an actin-dependent process that results in a rapid increase in internalization of fluid.118 This response seems to promote subsequent penetration of adenovirus from endocytic vacuoles by virus-induced rupture.
Another example is SV40, which is entirely dependent on signaling for entry. After binding to GM1 gangliosides, the virus induces local activation of tyrosine kinases, which results in actin filament reorganization, activation of caveolar dynamics, internalization of the virus in caveolar or lipid raft vesicles, and induction of long-distance transport of the virus-containing vesicles.44,135,145,187 More than 50 different kinases were shown to regulate the entry and early steps in the infection of HeLa cells by this virus.144
A final example involves Kaposi’s sarcoma–associated herpesvirus (human herpesvirus 8).28 The glycoprotein gB of this virus possesses an RGD sequence in the ectodomain that allows it to bind to the integrin α3β1. Binding activates focal adhesion kinase (FAK) and Src kinases, which, in turn, activate PI(3) kinases and Rho GTPases. Furthermore, via the PI(3)K-PKCzeta-mitogen activated or extracellular regulated kinase (MEK) pathway, the virus induces the extracellular signal-regulated kinase 1 and 2 (ERK1/2). Activation of these pathways leads to major alterations in the actin cytoskeleton, and the virus is internalized by macropinocytosis in human fibroblasts.
Endocytic Pathways of Infection
Whether viruses penetrate into the cytosol directly through the plasma membrane or after endocytosis has been a hotly debated issue from the beginning of animal virology. It is now recognized that a majority of animal viruses—whether enveloped or nonenveloped—make use of endocytosis for productive infection. They exploit one or more of several endocytic mechanisms offered by cells (Fig. 4.2), and most of them enter endocytic vacuoles where penetration into the cytosol occurs often triggered by low pH. Enveloped virus families (e.g., paramyxo-, herpes-, and retroviruses) that can penetrate directly through the plasma membrane because they do not require endocytosis for fusion may still depend on endocytosis for productive infection at least in some cell types.92,133 Here, the reason may be that fusion at the plasma membrane remains nonproductive because it does not ensure passage of the capsids through further barriers such as the actomyosin cortex.108
The main reason why endocytosis is a preferred mode of entry is most likely that endocytic vesicles offer viruses a free ride through the cortical cytoskeleton and other barriers that encumber movement of virus-sized particles in the cytoplasm. By delaying their penetration, viruses can in this way get a ride to the perinuclear region of the cell. In endocytic vacuoles, viruses can, moreover, count on receiving specific cues such as a drop in pH and exposure to proteases to trigger penetration
and uncoating. Because no trace of the virus is left exposed on the plasma membrane, immunorecognition of infected cell is delayed. Moreover, for nonenveloped viruses that use membrane lysis or pore formation for penetration, it may be essential to cross membranes of intracellular organelles to minimize damage to the cell.
and uncoating. Because no trace of the virus is left exposed on the plasma membrane, immunorecognition of infected cell is delayed. Moreover, for nonenveloped viruses that use membrane lysis or pore formation for penetration, it may be essential to cross membranes of intracellular organelles to minimize damage to the cell.
In recent years, the landscape of endocytosis research has expanded dramatically beyond phagocytosis and the classic clathrin-mediated endocytosis pathway. New mechanisms include caveolar or lipid raft-mediated endocytosis, macropinocytosis, and several other clathrin- and caveolae-independent pathways (Fig. 4.2).46,116,123,141,203 The situation is often confusing because the mode of uptake of a virus can vary between cell types and strains, and many viruses can make use of multiple receptors and parallel routes of endocytosis in the same cell. In addition to caveolae, SV40 can, for example, utilize a related, noncaveolar pathway.44 Influenza A uses both clathrin-mediated and clathrin-independent pathways,112,164,175 and HIV-1, which can fuse with the plasma membrane, can in some cell lines also make efficient use of an endocytic pathway for entry.42 The use of multiple receptors and redundant endocytic pathways provides viruses with a degree of flexibility and adaptability that make entry a difficult step for host organisms to protect themselves against.
The cell biology of endocytosis and its regulation is complex.35,98 Not surprisingly, the analysis of virus entry by high throughput siRNA silencing screens have led to the identification of hundreds of genes involved as critical factors in early infection of tissue culture cells.31,79,144 Entry studies are often further complicated by the fact that only a small fraction of the cell-associated viruses enter productively. Because most morphological and biochemical methods fail to distinguish between particles that enter productively and those that do not, studies using these methods must be complemented with readouts based on infection (i.e., the biological outcome of successful entry). This involves the use of inhibitors, dominant negative mutants, small interfering RNAs, mutant viruses, and mutant cell lines. Only a combination of methods allows pathways of productive entry to be charted with confidence.
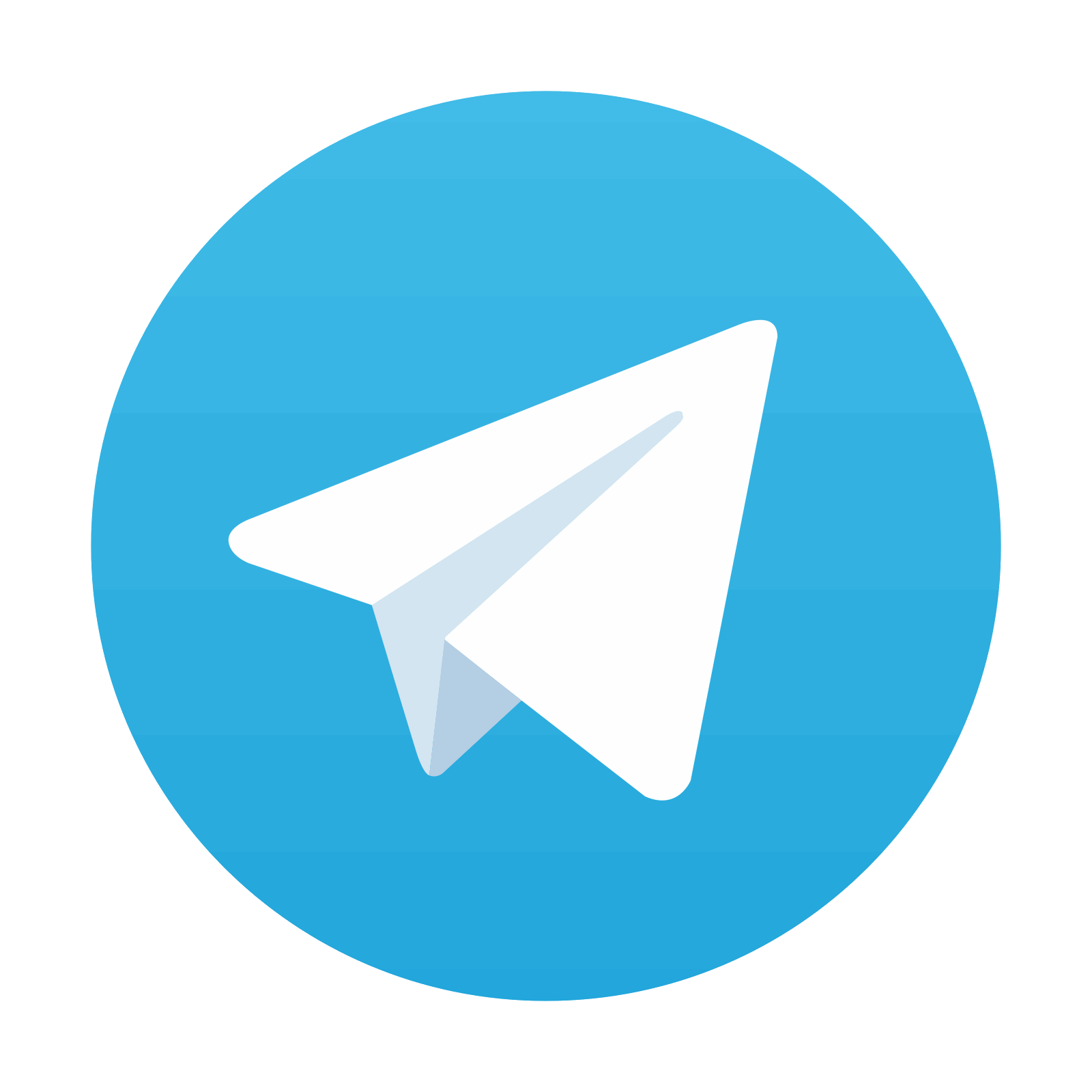
Stay updated, free articles. Join our Telegram channel

Full access? Get Clinical Tree
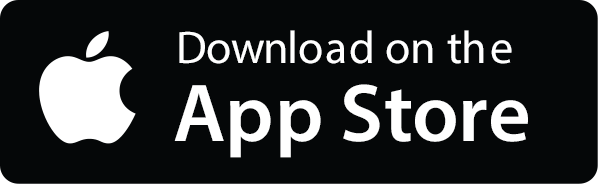
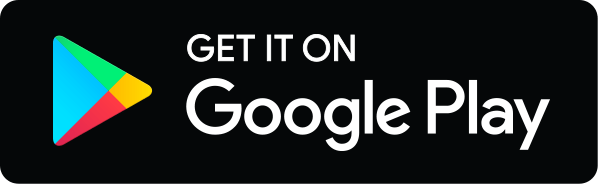