11 The cellular basis of adaptive immune responses
As we saw in a previous chapter, adaptive immune responses are generated by lymphocytes (Fig. 11.1), which are derived from stem cells differentiating within the primary lymphoid organs (bone marrow and thymus). From there, they colonize the secondary lymphoid tissues where they mediate the immune responses to antigens (Fig. 11.2). The lymph nodes are concerned with responses to antigens which are carried into them from the tissues, while the spleen is concerned primarily with antigens which reach it from the bloodstream (Fig. 11.3). Communication between these tissues and the rest of the body is maintained by a pool of recirculating lymphocytes which pass from the blood into the lymph nodes, spleen and other tissues and back to the blood by the major lymphatic channels such as the thoracic duct (Fig. 11.4). This traffic of lymphocytes between the tissues, the bloodstream and the lymph nodes enables antigen-sensitive cells to seek the antigen and to be recruited to sites at which a response is occurring. In addition, unencapsulated aggregates of lymphoid tissue termed ‘mucosa-associated lymphoid tissue’ or MALT, lie in the mucosal surface where they have the job of responding to antigens from the environment, particularly the heavy bacterial load in the intestine, by producing IgA antibodies for mucosal secretions. The lymphocytes which constitute the MALT system recirculate between these mucosal tissues using specialized homing receptors (Fig. 11.5).
B- and T-cell receptors
B and T cells can be distinguished by their surface markers
As they differentiate into populations with differing functions, B and T cells acquire molecules on their surface that reflect these specializations. It is possible to produce homogeneous antibodies of a single specificity, termed ‘monoclonal antibodies’, that can recognize such surface markers. When laboratories from all over the world compared the monoclonal antibodies they had raised, it was found that groups or clusters of monoclonal antibodies were each recognizing a common molecule on the surface of the lymphocyte. Each surface component so defined was referred to as a ‘CD’ molecule (Table 11.1), where CD refers to a ‘cluster of differentiation’.
Each lymphocyte expresses an antigen receptor of unique specificity on its surface
Among the surface markers on the B and T cells referred to above are the receptors on the plasma membrane which are used to identify foreign antigens. B cells possess surface immunoglobulin, whereas the T-cell receptor (TCR) on the surface of the T lymphocyte acts as an antigen recognition unit (see Fig. 10.9). We now know that despite the very large number of different components that could be combined together in multiple ways to give a diversity of surface receptors, each B lymphocyte rearranges its germline genes coding for these receptors so that it selects one and only one of the specificities for each receptor polypeptide chain. It then expresses that receptor molecule on its surface (Fig. 11.6). Once this occurs, the other genes coding for these antigen receptors in the lymphocyte are no longer used. In other words, following this genetic rearrangement process, the lymphocyte becomes committed to the synthesis and expression of a single receptor type. An analogous process occurs in the rearrangement of the αβ and γδ genes coding for the TCR. Just as for B cells, each T cell expresses one and only one specific combination of receptor peptides, and therefore shows a single specificity to which it is committed for the whole of its lifespan.
Clonal expansion of lymphocytes
Antigen selects and clonally expands lymphocytes bearing complementary receptors. As there are such a large number of different possible specificities that lymphocytes can express, perhaps of the order of millions, there must of necessity be only a relatively small number of particular specificities to which lymphocytes are committed. Thus, when a microbe invades the body, the total number of lymphocytes initially committed to recognizing the antigens that go to make up a particular microbe is relatively small, and must be expanded to provide a sufficient number to protect the host. Evolution has provided a masterful solution to this problem. When a microbe enters the body, its component antigens combine with only those B lymphocytes whose surface receptors are complementary to the shape of these antigens. The B cells that bind the antigen become activated and proliferate clonally under the influence of soluble growth factors termed cytokines (see section on Cytokines below) to form a large population of cells derived from the original (Fig. 11.7). The majority of these events occur within the lymphoid structure known as a germinal centre (see Fig. 11.3).
In the case of B cells, a large proportion of the clonally expanded lymphocytes become plasma cells (see Fig. 11.1), dedicated to the synthesis and secretion of antibodies. Since these plasma cells are derived from a parent cell that is already committed to the production of only one specific antibody, the final product is identical to the molecule that was posted on the surface of the original antigen-recognizing cell. Or at least almost so, because somatic mutation of the lymphocytes within the germinal centres which are synthesizing this antibody fine tunes the binding efficiency of the eventual product. The net result is that we have the production of large amounts of antibody which, like that on the surface of the parent cell, can combine with the invading antigen (Fig. 11.7).
A similar process of clonal selection and expansion occurs with T cells, producing a large number of T-cell effectors with the same specificity as the original parent cell; some of these cells release cytokines, whereas others have cytotoxic functions so that they act as effectors of T-cell-mediated immunity. One difference between T and B cells is that the T-cell receptors do not undergo further selection as a result of somatic mutation. Of crucial significance is the fact that in the case of both B and T cells, a fraction of the clonally expanded population differentiates into resting memory cells (Fig. 11.7). Thus, more cells are capable of recognizing the microbial antigen in any subsequent infection than in the initial virgin population that existed before the primary infection occurred. Human memory T cells can be identified by surface markers such as CD45RO, while memory B cells express CD27 and surface IgG, IgA or IgE.
The role of memory cells
Vaccination depends upon secondary immune responses being bigger and brisker than primary responses
In general, memory cells, as compared with naive cells, are more readily stimulated by a given dose of antigen. This occurs because they have greater combining power, in the case of B cells through mutation and selection during the primary response, and for T cells, which do not undergo affinity maturation, through increased expression of accessory adhesion molecules, CD2, LFA-1, LFA-3 and intercellular adhesion molecule-1 (ICAM-1), which enable the lymphocyte to bind more strongly to the specialized cells which present antigen. These factors, combined with the increased number of lymphocytes specific for a given antigen present in the memory pool produced by the primary response, result in a much stronger antibody or T-cell response on second contact with antigen. This provides the principle for vaccination (Fig. 11.8). The microbe or antigen to be used for vaccination is modified in such a way that it no longer produces disease or damage, but still retains the majority of its antigenic shapes. The primary response produced by the vaccination gives rise to a pool of memory cells, which can generate an abundant secondary response on subsequent contact with the antigen during a natural infection. Memory is usually long-lived, often extending over many years. There are many possible reasons for this: memory cells themselves may be innately long-lived or they may be sustained by gentle proliferation through subsequent contact with antigen present in reservoirs within the body or introduced by subclinical infection. An alternative mechanism in the case of T cells may be through stimulation by the cytokine IL-15 and in the case of B cells by anti-idiotypes (anti-antibodies produced in response to the combining region of the first antibody which may stimulate the memory B cells by ‘tweaking’ their surface receptors).
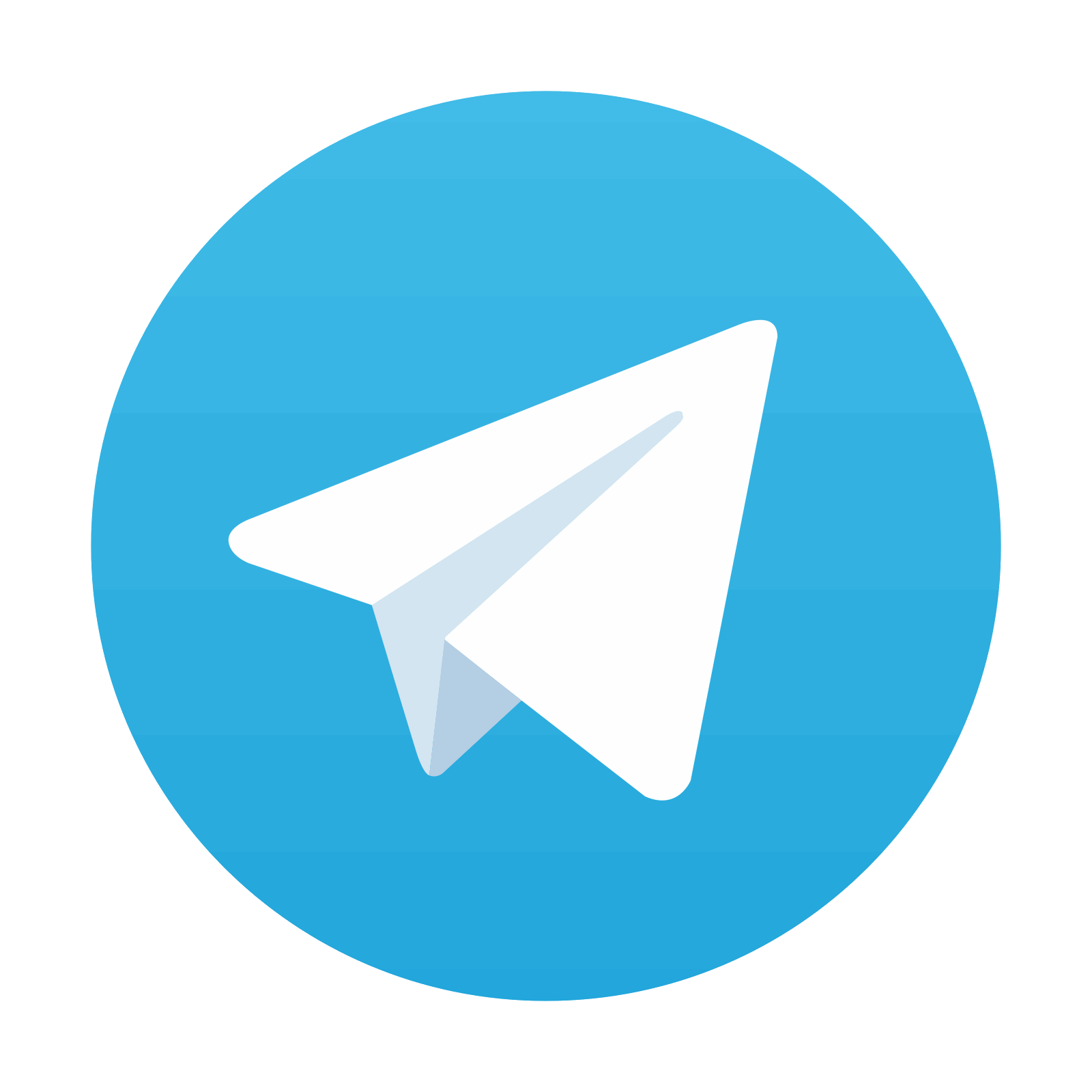
Stay updated, free articles. Join our Telegram channel

Full access? Get Clinical Tree
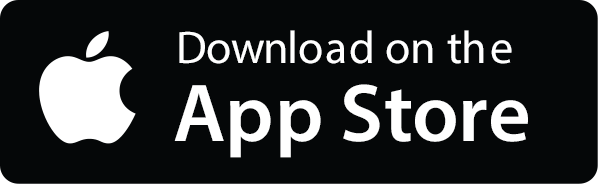
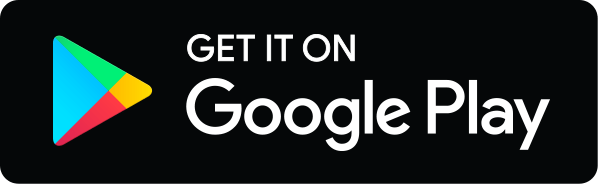