2 The bacteria
Structure
Bacteria are ‘prokaryotes’ and have a characteristic cellular organization
The genetic information of bacteria is carried in a long, double-stranded (ds), circular molecule of DNA (Fig. 2.1). By analogy with eukaryotes (see Ch. 1), this can be termed a ‘chromosome’, but there are no introns; instead, the DNA comprises a continuous coding sequence of genes. The chromosome is not localized within a distinct nucleus; no nuclear membrane is present and the DNA is tightly coiled into a region known as the ‘nucleoid’. Genetic information in the cell may also be extrachromosomal, present as small circular self-replicating DNA molecules termed plasmids. The cytoplasm contains no organelles other than ribosomes for protein synthesis. Although ribosomal function is the same in both pro- and eukaryotic cells, organelle structure is different. Ribosomes are characterized as 70 S in prokaryotes and 80 S in eukaryotes (the ‘S’ unit relates to how a particle behaves when studied under extreme centrifugal force in an ultracentrifuge). The bacterial 70 S ribosome is specifically targeted by antimicrobials such as the aminoglycosides (see Ch. 33). Many of the metabolic functions performed in eukaryote cells by membrane-bound organelles such as mitochondria are carried out by the prokaryotic cell membrane. In all bacteria except mycoplasmas, the cell is surrounded by a complex cell wall. External to this wall may be capsules, flagella and pili. Knowledge of the cell wall and these external structures is important in diagnosis and pathogenicity and for understanding bacterial biology.
Bacteria are classified according to their cell wall as Gram-positive or Gram-negative
Gram staining is a basic microbiologic procedure for detection and identification of bacteria (see Ch. 32). The main structural component of the cell wall is a ‘peptidoglycan’ (mucopeptide or murein), a mixed polymer of hexose sugars (N-acetylglucosamine and N-acetylmuramic acid) and amino acids.
• In Gram-positive bacteria, the peptidoglycan forms a thick (20–80 nm) layer external to the cell membrane, and may contain other macromolecules.
• In Gram-negative species, the peptidoglycan layer is thin (5–10 nm) and is overlaid by an outer membrane, anchored to lipoprotein molecules in the peptidoglycan layer. The principal molecules of the outer membrane are lipopolysaccharides and lipoprotein (Fig. 2.2).
The polysaccharides and charged amino acids in the peptidoglycan layer make it highly polar, providing the bacterium with a thick hydrophilic surface. This property allows Gram-positive organisms to resist the activity of bile in the intestine. Conversely, the layer is digested by lysozyme, an enzyme present in body secretions, which therefore has bactericidal properties. Synthesis of peptidoglycan is disrupted by beta-lactam and glycopeptides antibiotics (see Ch. 33).
In Gram-negative bacteria, the outer membrane is also hydrophilic, but the lipid components of the constituent molecules give hydrophobic properties as well. Entry of hydrophilic molecules such as sugars and amino acids is necessary for nutrition and is achieved through special channels or pores formed by proteins called ‘porins’. The lipopolysaccharide (LPS) in the membrane confers both antigenic properties (the ‘O antigens’ from the carbohydrate chains) and toxic properties (the ‘endotoxin’ from the lipid A component; see Ch. 17).
The cell wall is a major contributor to the ultimate shape of the organism, an important characteristic for bacterial identification. In general, bacterial shapes (Fig. 2.3) are categorized as either spherical (cocci), rods (bacilli) or helical (spirilla), although there are variations on these themes.
Pili are another form of bacterial surface projection
Pili (fimbriae) are more rigid than flagella and function in attachment, either to other bacteria (the ‘sex’ pili) or to host cells (the ‘common’ pili). Adherence to host cells involves specific interactions between component molecules of the pili (adhesins) and molecules in host cell membranes. For example, the adhesins of Escherichia coli interact with fucose/mannose molecules on the surface of intestinal epithelial cells (see Ch. 22). The presence of many pili may help to prevent phagocytosis, reducing host resistance to bacterial infection. Although immunogenic, their antigens can be changed, allowing the bacteria to avoid immune recognition. The mechanism of ‘antigenic variation’ has been elucidated in the gonococci and is known to involve recombination of genes coding for ‘constant’ and ‘variable’ regions of pili molecules.
Nutrition
Bacteria obtain nutrients mainly by taking up small molecules across the cell wall
Some species require only minimal nutrients in their environment, having considerable synthetic powers, whereas others have complex nutritional requirements. E. coli, for example, can be grown in media providing only glucose and inorganic salts; streptococci, on the other hand, will grow only in complex media providing them with many organic compounds. Nevertheless, all bacteria have similar general nutritional requirements for growth which are summarized in Table 2.1.
Table 2.1 Major nutritional requirements for bacterial growth
Element | Cell dry weight (%) | Major cellular role |
---|---|---|
Carbon | 50 | Molecular ‘building block’ obtained from organic compounds or CO2 |
Oxygen | 20 | Molecular ‘building block’ obtained from organic compounds, O2 or H2O; O2 is an electron acceptor in aerobic respiration |
Nitrogen | 14 | Component of amino acids, nucleotides, nucleic acids and coenzymes obtained from organic compounds and inorganic sources such as NH4+ |
Hydrogen | 8 | Molecular ‘building block’ obtained from organic compounds, H2O, or H2; involved in respiration to produce energy |
Phosphorus | 3 | Found in a variety of cellular components including nucleotides, nucleic acids, lipopolysaccharide (lps) and phospholipids; obtained from inorganic phosphates (![]() |
Sulphur | 1–2 | Component of several amino acids and coenzymes; obtained from organic compounds and inorganic sources such as sulfates (![]() |
Potassium | 1–2 | Important inorganic cation, enzyme cofactor, etc., obtained from inorganic sources |
All pathogenic bacteria are heterotrophic
• In aerobic metabolism (i.e. aerobic respiration), complete utilization of an energy source such as glucose produces 38 molecules of ATP.
• Anaerobic metabolism utilizing an inorganic molecule other than oxygen as the final hydrogen acceptor (anaerobic respiration) is incomplete and produces fewer ATP molecules than aerobic respiration.
• Anaerobic metabolism utilizing an organic final hydrogen acceptor (fermentation) is much less efficient and produces only two molecules of ATP.
Anaerobic metabolism, while less efficient, can thus be used in the absence of oxygen when appropriate substrates are available, as they usually are in the host’s body. The requirement for oxygen in respiration may be ‘obligate’ or it may be ‘facultative’, some organisms being able to switch between aerobic and anaerobic metabolism. Those that use fermentation pathways often use the major product pyruvate in secondary fermentations by which additional energy can be generated. The interrelationship between these different metabolic pathways is illustrated in Figure 2.4.
The ability of bacteria to grow in the presence of atmospheric oxygen relates to their ability to enzymatically deal with potentially destructive intracellular reactive oxygen species (e.g. free radicals, anions containing oxygen, etc.) (Table 2.2). The interaction between these harmful compounds and detoxifying enzymes such as superoxide dismutase, peroxidase, and catalase is illustrated in Figure 2.5 (also see Ch. 9 and Box 9.2).
Growth and division
The rate at which bacteria grow and divide depends in large part on the nutritional status of the environment. The growth and division of a single E. coli cell into identical ‘daughter cells’ may occur in as little as 20–30 min in rich laboratory media, whereas the same process is much slower (1–2 h) in a nutritionally depleted environment. Conversely, even in the best environment, other bacteria such as Mycobacterium tuberculosis may grow much more slowly, dividing every 24 h. When introduced into a new environment, bacterial growth follows a characteristic pattern depicted in Figure 2.6. After an initial period of adjustment (lag phase), cell division rapidly occurs, with the population doubling at a constant rate (generation time), for a period termed log or exponential phase. As nutrients are depleted and toxic products accumulate, cell growth slows to a stop (stationary phase) and eventually enters a phase of decline (death).
Cell division is preceded by genome segregation and septum formation
The process of cell division (or septation) involves:
The septum is formed by an invagination of the cytoplasmic membrane and ingrowth of the peptidoglycan cell wall (and outer membrane in Gram-negative bacteria). Septation and DNA replication and genome segregation are not tightly coupled, but are sufficiently well coordinated to ensure that very few daughter cells do not have the correct complement of genomic DNA.
Bacterial growth and division are important targets for antimicrobial agents
Antimicrobials that target the processes involved in bacterial growth and division include:
• quinolones (ciprofloxacin and levofloxacin), which inhibit the unwinding of DNA by DNA gyrase during DNA replication
• the many inhibitors of peptidoglycan cell wall synthesis (e.g. beta-lactams such as the penicillins, cephalosporins and carbapenems, and glycopeptides such as vancomycin).
These are considered in more detail in Chapter 33.
Gene expression
Transcription
Transcription is initiated at promoters
• the exact DNA sequence of the promoter site
• the overall topology (supercoiling) of the DNA
• the presence or absence of regulatory proteins that bind adjacent to and may overlap the promoter site.
Consequently, different promoters have widely different rates of transcriptional initiation (of up to 3000-fold). Their activities can be altered by regulatory proteins. Sigma factor (a component RNA polymerase) plays an important role in promoter recognition. The presence of several different sigma factors in bacteria enables sets of genes to be switched on simply by altering the level of expression of a particular sigma factor. This is particularly important in controlling the expression of genes involved in spore formation in Gram-positive bacteria.
mRNA transcripts often encode more than one protein in bacteria
The bacterial arrangement seen for single genes (promoter- structural-gene-transcriptional-terminator) is described as monocistronic. However, a single promoter and terminator may flank multiple structural genes, a polycistronic arrangement known as an operon. Operon transcription thus results in polycistronic mRNA encoding more than one protein (Fig. 2.7). Operons provide a way of ensuring that protein subunits that make up particular enzyme complexes or are required for a specific biological process are synthesized simultaneously and in the correct stoichiometry. For example, the proteins required for the uptake and metabolism of lactose are encoded by the lac operon. Many of the proteins responsible for the pathogenic properties of medically important microorganisms are likewise encoded by operons, for example:
• cholera toxin from Vibrio cholerae
• fimbriae (pili) of uropathogenic E. coli, which mediate colonization.
Translation
Regulation of gene expression
Bacteria adapt to their environment by controlling gene expression
• Bacteria may encounter a new source of carbon or nitrogen and as a consequence switch on new metabolic pathways that enable them to transport and use such compounds.
• When compounds such as amino acids are depleted from a bacterium’s environment the bacterium may be able to switch on the production of enzymes that enable it to synthesize the particular molecule it requires de novo.
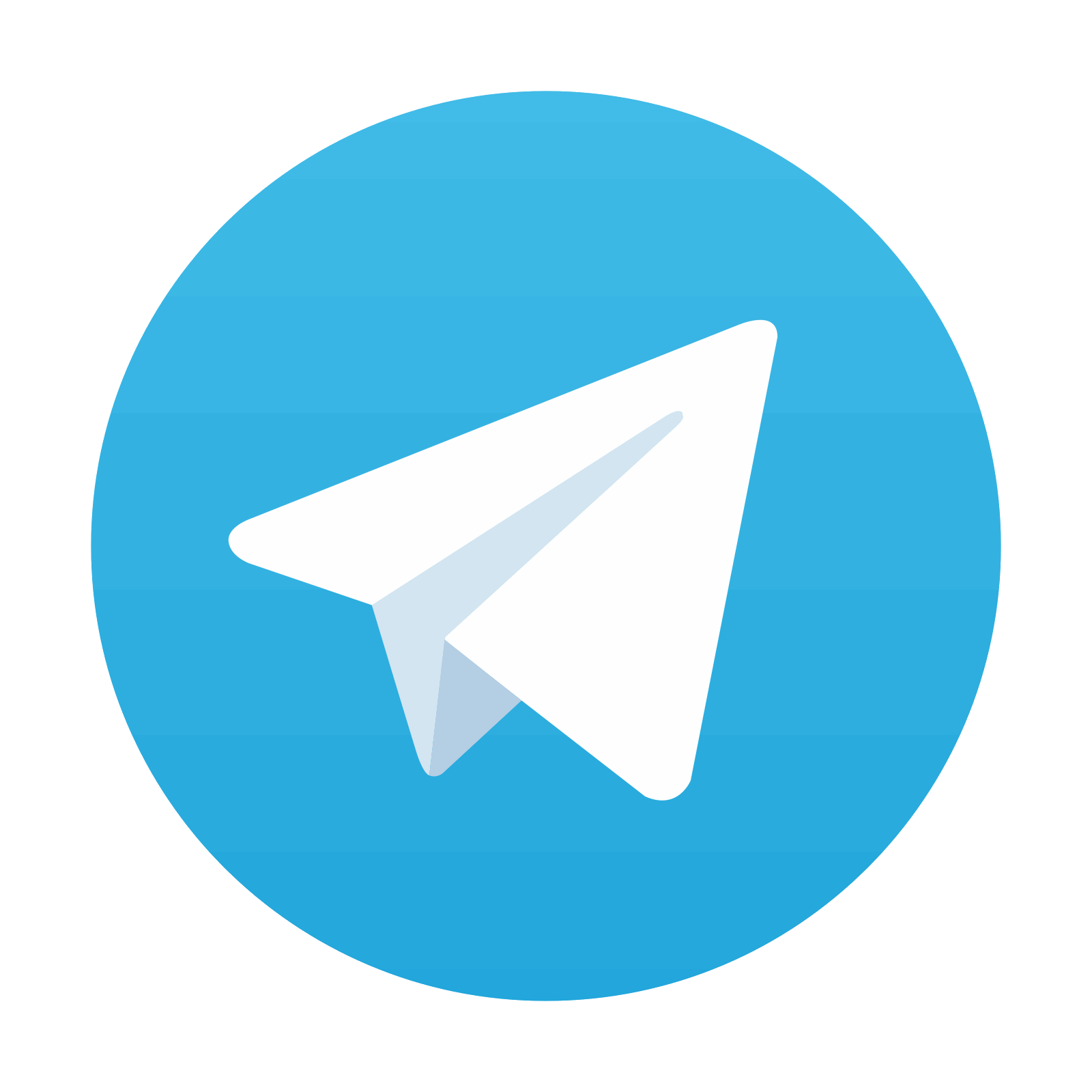
Stay updated, free articles. Join our Telegram channel

Full access? Get Clinical Tree
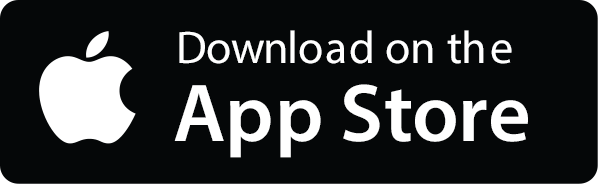
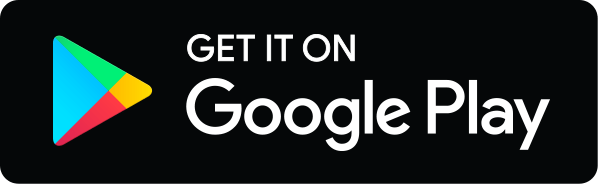