34 Protecting the host
vaccination
Vaccines are one of the most effective public health tools. This chapter will review how vaccines work, and the vaccines in current use. However, although vaccination is very cost-effective as a public health measure, saving many lives (immunization saves an estimated 2.5 million deaths each year from diphtheria, tetanus, pertussis and measles), in 2008 an estimated 1.8 million people died from a vaccine-preventable disease, as a result of poor vaccine uptake. Many others die from infectious diseases such as HIV for which there is as yet no effective vaccine, so new vaccines are also needed (Table 34.1).
Table 34.1 Infectious agents that are major killers
Organism | Disease | Estimated annual deaths (millions) |
---|---|---|
HIV | AIDS | 1.8 |
Mycobacterium tuberculosis | Tuberculosis | 1.7 |
Plasmodium spp. | Malaria | 0.8 |
Total | 4.3 |
We currently lack effective vaccines against these organisms, although bacille Calmette–Guérin (BCG) vaccination can provide protection against disseminated forms of childhood tuberculosis, and pulmonary tuberculosis in some parts of the world. Most of the deaths from HIV are in Africa, and most of the deaths from malaria are in African children. Sources: Figures for 2009 from WHO.
Vaccination exploits the ability of the immune system to develop immunological memory, so that it can rapidly mobilize its forces to fight infection when required. Vaccines can be of different types, including live attenuated organisms, killed organisms, or subunit vaccines. Depending on the vaccine type, more than one dose may be needed to achieve or maintain optimal protection. Adjuvants are often required to increase immunity. A lot has been learnt about how to make a good vaccine, but for some vaccines we lack measurable correlates of protection. It is therefore no surprise that the development of new and more effective vaccines is a major area of research. Successful vaccination also requires an understanding of the epidemiology of disease transmission, for example, to estimate what proportion of a given population needs to be vaccinated to produce herd immunity, as discussed in Chapter 31.
Vaccination – a four hundred year history
‘Never in the history of human progress’, wrote the pathologist Geoffrey Edsall, ‘has a better and cheaper method of preventing illness been developed than immunization at its best’. The greatest success story in medicine, the elimination of smallpox, began before either immunology or microbiology were recognized as disciplines – indeed before the existence of microbes or the immune system was even suspected. As a result of the pioneering work of Jenner with vaccinia (Box 34.1), all forms of specific, actively induced immunity are now referred to as ‘vaccination’.
Box 34.1 Lessons in Microbiology
Edward Jenner (1749–1823)
The English physician Edward Jenner (Fig. 34.1) is regarded as the founder of modern vaccination, but he was by no means the first to try the technique. The ancient practice of ‘variolation’ dates back to tenth-century China, and arrived in Europe in the early eighteenth century via Turkey. The technique involved the inoculation of children with dried material from healed scabs of mild smallpox cases, and was a striking foretaste of the principles of modern attenuated viral vaccines. This practice was, however, both inconsistent and dangerous, and Jenner’s innovation was to show that a much safer and more reliable protection could be obtained by deliberate inoculation with cowpox (vaccinia) virus. Milkmaids exposed to cowpox were traditionally known to be resistant to smallpox and so retained their smooth complexions. In 1796, Jenner tested his theory by inoculating 8-year-old James Phipps with liquid from a cowpox pustule on the hand of Sarah Nelmes. Subsequent inoculation of the boy with smallpox produced no disease. Although greeted with scepticism at first, Jenner’s ideas soon became accepted, and he went on to inoculate thousands of patients in a shed in the garden of his house at Berkeley, Gloucestershire. He ultimately achieved world fame, though his fellowship of the Royal Society was conferred for a quite different piece of work on the nesting habits of the cuckoo!
The principle of vaccination is simple: to prime the adaptive immune system to the antigens of a particular microbe so that on first contact with the live organism a rapid and effective secondary immune response will be induced by memory T and B cells. Vaccination therefore depends upon the ability of lymphocytes, both B and T cells, to respond to specific antigens and develop into memory T and B cells, and represents a form of actively enhanced adaptive immunity. The passive administration of preformed elements, such as antibody, is considered in Chapter 35.
Aims of vaccination
The aims of vaccination can vary from preventing symptoms to eradication of disease
The most ambitious aim of vaccination is eradication of the disease. This has been achieved for smallpox, the eradication of polio is being attempted, and there has clearly been a dramatic downward trend in the incidence of most of the diseases against which vaccines are currently in use (Fig. 34.2). However, as long as any focus of infection remains in the community, the main effect of vaccination will be protection of the vaccinated individual against infection.
The importance of herd immunity
Successful vaccination programmes rely not only on the development and use of vaccines themselves, but also on an understanding of the epidemiologic aspects of disease transmission. If enough individuals in a population are immunized, this will reduce or stop transmission of the infection. This is called herd immunity. By having your own child immunized, you therefore help protect the whole community – but conversely, when too many parents decide that their child will not be immunized, because they think the risk of their child getting the disease is low, this may contribute to the disease becoming more common (Fig. 34.2)
Vaccines can be of different types
Vaccines can be based on whole organisms, either live or inactivated, or components of the infectious agent (Table 34.2).
Types of vaccine | Examples |
---|---|
Live attenuated | |
Viral | Measles, mumps, rubella, vaccinia, varicella, yellow fever, zoster, oral polio, intranasal influenza, rotavirus |
Bacterial | BCG, oral typhoid |
Inactivated | |
Whole virus | Polio, influenza, hepatitis A, rabies |
Whole bacteria | Pertussis, cholera, typhoid |
Fractions | |
Toxoids | Diphtheria, tetanus |
Protein subunits | Hepatitis B, influenza, acellular pertussis, human papillomavirus |
Polysaccharides | Pneumococcal, meningococcal, Salmonella typhi (Vi), Haemophilus influenzae type b |
Conjugates | Haemophilus influenzae type b, pneumococcal, meningococcal |
Note that not all types of vaccine are available in all countries. Vaccines are also available for bioterrorism agents such as anthrax and plague, and for vaccinia.
Live vaccines are designed to induce immunity in a similar way to the actual infection. Most live vaccines use attenuated organisms that were attenuated using culture in eggs, animals or in tissue culture (Fig. 34.3); these attenuated organisms replicate to a limited extent in the vaccinated individual but do not cause disease in healthy people. However, the immunosuppression associated with HIV infection can produce problems with live vaccines. For example, infants with HIV infection given BCG vaccination can develop disseminated BCGosis. HIV-infected individuals with severe immunosuppression should not be given live vaccines such as measles or varicella, but they can be given inactivated vaccines.
Inactivated vaccines are safe to use in the immunocompromised, although they may not be as immunogenic, and a good adjuvant may be needed. Inactivation can use heat or fixation, for example, with formalin. Types of fixatives and preservatives in use in vaccines are given in Table 34.3. Another difference between live and attenuated vaccines is that inactivated vaccines are not affected by circulating antibody, but live attenuated vaccines may be.
Table 34.3 Fixatives and preservatives used in current vaccines
Fixatives | |
Formalin | TdaP, HepA, Hib, influenza, polio |
Glutaraldehyde | DtaP, DtaP-HepB-IPV |
Preservatives | |
EDTA | Rabies, varicella |
Phenol | Pneumococcal, inactivated typhoid |
2-phenoxyethanol | DtaP, HepA, HepA-HepB, inactivated poliovirus |
β-propiolactone | Rabies |
Sodium deoxycholate | Influenza |
Thiomersal | DtaP, DtaP-Hib, HepB, influenza, meningococcal vaccine |
TdaP, combined tetanus, diphtheria and pertussis; DtaP, combined diphtheria, tetanus and pertussis; HepA, hepatitis A; HepB, hepatitis B; Hib, Hameophilus influenzae type b; IPV, inactivated polio vaccine.
Some vaccines are designed to boost immunity using only selected antigens or by using a different delivery route. For example, some new TB vaccines in development would boost the immunity induced by BCG by giving key antigens delivered by a viral vector (Fig. 34.4), or a fusion protein with adjuvant.
Adjuvants
Adjuvants increase the immunity induced by a vaccine in a number of ways. Adjuvants can improve the immune response to the vaccine antigens through inducing activation of Toll-like receptors (TLR) on dendritic cells to improve antigen presentation, or by forming an antigen depot which allows antigen to persist and to leak out slowly over time. The earliest adjuvants consisted of water in oil emulsions, and Freund’s complete adjuvant which included dead mycobacteria in a water in oil emulsion is very effective, although not suitable for use in humans. Other adjuvants increase antigen presentation, or enhance particular types of immunity, such as antibodies or Th1 immunity. The dramatic effect of adding an adjuvant to a vaccine is shown in Figure 34.5. Aluminium salts are powerful adjuvants and used in many vaccines (Box 34.2). Experimentally, cytokines such as IL-1, IL-2, IFNγ, IL-12 and IL-18, as well as some chemokines, have been tested as adjuvants. Compounds such as liposomes, lipid containing vesicles, have also been used, but so far only monophosphoryl lipid A has been used in a licensed vaccine (HPV, Cervarix).
Box 34.2 Adjuvants in Currently Used Vaccines
Aluminium salts* | DTaP, Hib, HepA, HepB, HPV, MMR, rabies, rotavirus, rubella, varicella |
Monophosphoryl lipid A (MPL) | HPV (Cervarix) |
Vaccine safety
As vaccines are given to healthy individuals, it is important that they are safe. In 1926, live M. tuberculosis was inadvertently given to healthy children instead of BCG, leading to the Lubeck disaster, and in 1942, US military personnel were vaccinated with yellow fever virus contaminated with hepatitis B virus (see Ch. 15). Safety testing is now rigorous, requiring extensive quality controls and animal testing, prior to trials or use in humans. Some of the more important issues are summarised in Box 34.3. It is particularly critical that vaccines derived from live organisms are inactivated to ensure they are safe and that vaccines are preserved appropriately to ensure that vaccine immunogenicity is retained. Examples of fixatives and preservatives used in current vaccines are given in Table 34. 3.
Vaccines in current use
Diphtheria, tetanus and pertussis
The first vaccine developed against pertussis was a whole cell vaccine, which was available from the mid 1940s and introduced in the UK in 1957 (Fig. 34.6). However, although four doses of vaccine induced 70–90% protection against serious whooping cough, concerns over the safety of the vaccine in the UK in the 1970s led to resurgence of disease. Whether the vaccine did induce brain damage is unclear, but concerns did lead to the development of a safer acellular pertussis vaccine.
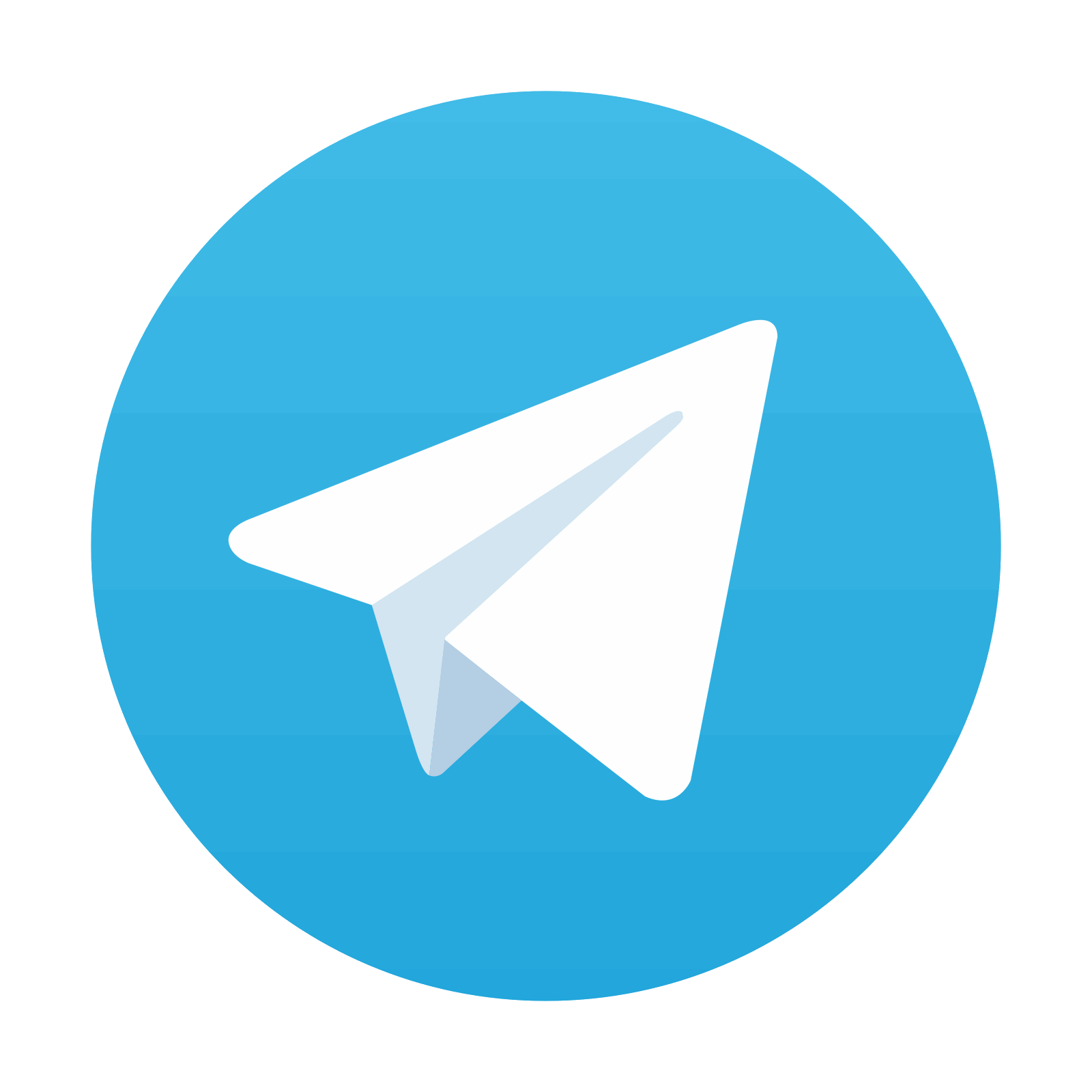
Stay updated, free articles. Join our Telegram channel

Full access? Get Clinical Tree
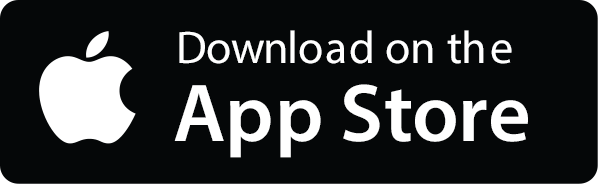
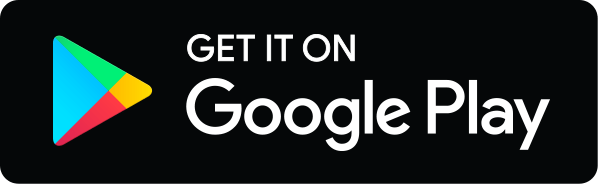