1 Introduction
1.1 Parasitism
Parasitism is a specific type of interaction between two organisms that has many features in common with other infectious processes, but host-parasite interactions often operate over a longer timescale than those seen with other pathogens. This extended process results in significant host-parasite interaction at the cellular and organismal level. It is known, for example, that some parasites alter the behaviour of the host, while others, such as Giardia lamblia, induce biochemical change in the host cells at the site of infection (the duodenal epithelium). Most parasites have a life cycle that often involves several hosts; this means that survival and transmission between different hosts requires the parasite to exhibit more than one physiologically distinct form.
1.2 Habitats
Parasites inhabit a wide range of habitats within their hosts. Some parasites will inhabit only one site throughout their life cycle, but many move to various sites within the body. Such movement may require the formation of motile cellular forms, and it will produce a significant change in the physiology and morphology of the parasite as a result of environmental change. Parasites moving from the gut to other tissues, for example, will encounter higher levels of oxygen, changes in pH and significant exposure to the host immune response. When life cycles involve more than one host organism these changes are greater. The reasons why parasites move to various sites in with the host is driven by evasion of host immune attack and to aid transmission.
1.3 Physiology of parasitic protozoa
Parasitic protozoa, like their free-living counterparts, are single-celled eukaryotic organisms that utilize flagella, cilia or amoeboid movement for motility. The complexity of some parasite life cycles means that some species may exhibit, at different times, more than one form of motility. All pathogenic protozoa are heterotrophs, using carbohydrates or amino acids as their major source of carbon and energy. Some parasitic protozoa utilize oxygen to generate energy through oxidative phosphorylation, but many protozoan parasites lack functional or ‘typical’ mitochondria, or have mitochondria that do not function like those in mammalian cells. As a result of this adaptation many parasites exhibit a fermentative metabolism that functions even in the presence of oxygen. The reason for the utilization of less efficient fermentative pathways is not clear, but it is presumably due in part to the fact that such parasites survive in environments where oxygen is only present occasionally or at low levels. For some parasites oxygen is toxic, and they appear to utilize it possibly in an effort to remove it and thus maintain an anaerobic metabolism.
The metabolism of parasites is highly adapted, with many possessing unique organelles such as kienetoplasts and hydrogenosomes. Many synthetic pathways that are found in other eukaryotes are absent because many metabolic intermediates or precursors such as lipids, amino acids and nucleotides are actively scavenged from their environment. This minimizes energy expenditure, which is finely balanced in parasites and means that the membrane of parasitic protozoa is rich in transporters. Secretion of haemolysins, cytolysins, proteolytic enzymes, toxins, antigenic and immunomodulatory molecules that reduce host immune response also occurs in pathogenic protozoa.
Survival of parasites is partly due to their high rate of reproduction, which may be either sexual or asexual; some organisms such as Plasmodium exhibit both forms of reproduction in their life cycle. Simple fission is characteristic of many amoeba, but some species also undergo nuclear division in the cystic state (cysts are forms required for survival outside the host) with each nucleus giving rise to new trophozoites (the growing, motile and pathogenic form).
This section considers the life cycles, disease and pathology of some blood and tissue parasites; this is not an exhaustive list but covers some of the most important species. These diseases are commonly associated with travel to tropical and subtropical countries, but diseases such as leishmaniasis are frequently seen in southern Spain and France. It should also be noted that climate change is altering the geographical distribution of many parasitic diseases.
2.1 Malaria
Malaria has been a major disease of humankind for thousands of years. Despite the availability of drugs for treatment, malaria is still one of the most important infectious diseases of humans, with approximately 200–500 million new cases and 1–2.5 million deaths each year. Protozoa of the genus Plasmodium cause malaria and four species are responsible for the disease in humans: P. falciparum, P. vivax, P. ovale and P. malariae. P. falciparum and P. vivax account for the vast majority of cases, although P. falciparum causes the most severe disease. Other species of plasmodia infect reptiles, birds and other mammals. Malaria is spread to humans by the bite of female mosquitoes of the genus Anopheles but transmission by inoculation of infected blood and through congenital routes is also seen. These mosquitoes feed at night and their breeding sites are primarily in rural areas.
2.1.1 Disease
The most common symptom of malaria is fever, although chills, headache, myalgia and nausea are frequently seen and other symptoms such as vomiting, diarrhoea, abdominal pain and cough occasionally appear. In all types of malaria, the periodic febrile response (fever) is caused by rupture of mature schizonts (one of the cell forms arising as part of the life cycle). In P. vivax and P. ovale malaria fever occurs every 24–48 hours, whereas in P. malariae, maturation occurs every 72 hours. In falciparum malaria fever may occur every 48 hours, but is usually irregular, showing no distinct periodicity. Apart from anaemia, most physical findings in malaria are often non-specific and offer little aid in diagnosis, although enlargement of some organs may be seen after prolonged infection. If the diagnosis of malaria is missed or delayed, especially with P. falciparum infection, potentially fatal complicated malaria may develop. The most frequent and serious complications of malaria are cerebral malaria and severe anaemia.
2.1.2 Life cycle
Plasmodia have a complex life cycle (Figure 6.1) involving a number of life cycle stages and two hosts. The human infective stage comprises the sporozoites (c.1–7μm), which are produced by sexual reproduction in the midgut of the mosquito (vector) and migrate to its salivary gland. When an infected Anopheles mosquito bites a human, sporozoites are injected into the bloodstream and are thought to enter liver parenchymal cells within 30 minutes of inoculation. In these cells the parasite differentiates into a spherical, multinucleate schizont which may contain 2000–40 000 uninucleate merozoites. This process of growth and development is termed exoerythrocytic schizogony. This exoerythrocytic phase usually takes between 5 and 21 days, depending on the species of Plasmodium; however, in P. vivax and P. ovale the maturation of schizonts may be delayed for up to 1–2 years. These ‘quiescent’ parasites are called hypnozoites. Clinical illness is caused by the erythrocytic stage of the parasite life cycle; no disease is associated with sporozoites, the developing liver stage of the parasite, the merozoites released from the liver, or gametocytes.
Figure 6.1 The malaria parasite life cycle involves two hosts. During a blood meal, a malaria-infected female Anopheles mosquito inoculates sporozoites into the human host (1). Sporozoites infect liver cells (2) and mature into schizonts (3), which rupture and release merozoites (4). (Of note, in P. vivax and P. ovale a dormant stage (hypnozoites) can persist in the liver and cause relapses by invading the bloodstream weeks, or even years later.) After this initial replication in the liver (exo-erythrocytic schizogony, A), the parasites undergo asexual multiplication in the erythrocytes (erythrocytic schizogony, B). Merozoites infect red blood cells (5). The ring stage trophozoites mature into schizonts, which rupture releasing merozoites (6). Some parasites differentiate into sexual erythrocytic stages (gametocytes) (7). Blood-stage parasites are responsible for the clinical manifestations of the disease. The gametocytes, male (microgametocytes) and female (macrogametocytes), are ingested by an Anopheles mosquito during a blood meal (8). The parasite’s multiplication in the mosquito is known as the sporogonic cycle (C). While in the mosquito’s stomach, the microgametes penetrate the macrogametes, generating zygotes (9). The zygotes in turn become motile and elongated (ookinetes) (10), which invade the midgut wall of the mosquito where they develop into oocysts (11). The oocysts grow, rupture and release sporozoites (12), which make their way to the mosquito’s salivary glands. Inoculation of the sporozoites into a new human host perpetuates the malaria life cycle (1).
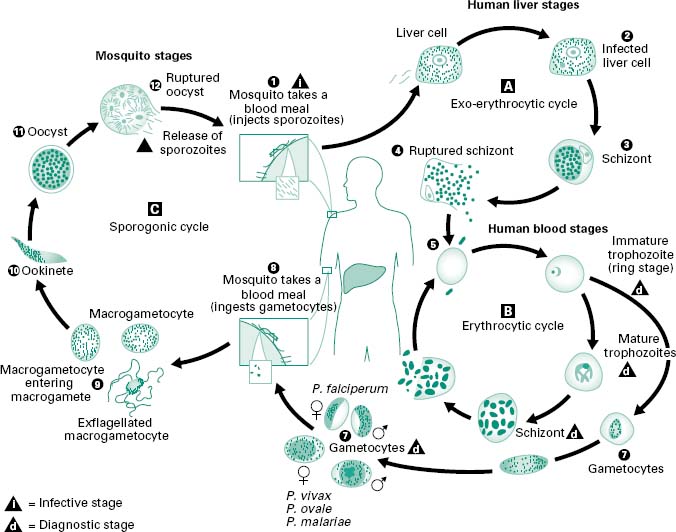
The common symptoms of malaria are due to the rupture of erythrocytes when erythrocytic schizonts mature (Figure 6.2a). This release of parasite material triggers a host immune response, which in turn induces the formation of inflammatory cytokines, reactive oxygen intermediates and other cellular products. These proinflammatory molecules play a prominent role in pathogenesis, and are probably responsible for the fever, chills, sweats, weakness and other systemic symptoms associated with malaria. In P. falciparum malaria, infected erythrocytes adhere to the endothelium of capillaries and postcapillary venules, leading to obstruction of the microcirculation and localized anoxia. The pathogenesis of anaemia appears to involve haemolysis or phagocytosis of parasitized erythrocytes and ineffective erythropoiesis.
Figure 6.2 (a) Mature schizonts of Plasmodium vivax. P. vivax schizonts are large, have 12-24 merozoites, and may fill the red blood cell (RBC). RBCs are enlarged 1.5–2 times and may be distorted. Under optimal conditions Sch üffner’s dots may be seen. (b) Leishmania tropica amastigotes within an intact macrophage. (c) Toxoplasma gondii trophozoites in the bronchial secretions from an HIV-infected patient. (d) Trophozoites of Giardia intestinalis. Each cell has two nuclei and is 10–20 mm in length. (e) Trophozoites of Entamoeba histolytica with ingested erythrocytes, which appear as dark inclusions. (f) Oocysts of Cryptosporidium parvum (upper left) and cysts of Giardia intestinalis (lower right) labelled with immunofluorescent antibodies. (g) Trophozoites of Trichomonas vaginalis.
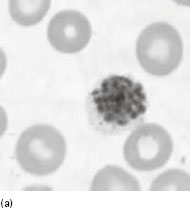
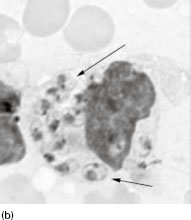
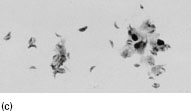
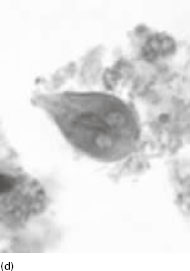
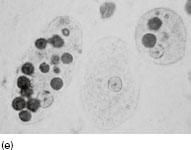
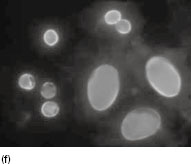
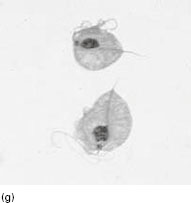
2.2 Trypanosomatids
The family Trypanosomatidae consists of two genera, Trypanosoma and Leishmania. These are important pathogens of humans and domestic animals and the diseases they cause constitute serious medical and economic problems. Because these protozoans have a requirement for haematin obtained from blood, they are called haemoflagellates. The life cycles of both genera involve insect and vertebrate hosts and have up to eight life cycle stages, which differ in the placement and origin of the flagellum. Trypanosomatids have a unique organelle called the kinetoplast. This appears to be a special part of the mitochondrion and is rich in DNA. Two types of DNA have been found in the kinetoplast: maxicircles that encode many mitochondrial enzymes, and minicircles, which serve a function in the process of RNA editing. Replication of trypanosomatids occurs by single or multiple fission, involving first the kinetoplast, then the nucleus, and finally the cytoplasm. There are four major diseases associated with this group: Chagas disease is caused by Trypanosoma cruzi; sleeping sickness (African trypanosomiasis) is associated with T. brucei; cutaneous and mucocutaneous leishmaniasis are caused by a range of species including Leishmania tropica, L. major, L. mexicana, L. amazonensis and L. braziliensis; and visceral leishmaniasis, which is also known as kala-azar, is typically caused by L. donovani.
2.2.1 American trypanosomiasis (Chagas disease)
Chagas disease begins as a localized infection that is followed by parasitaemia and colonization of internal organs and tissues. Infection may first be evidenced by a small tumour (chagoma) on the skin. Symptoms of the disease include fever, oedema and myocarditis (infection of the heart muscle) with or without heart enlargement, and meningoencephalitis in children. The acute disease is frequently subclinical and patients may become asymptomatic carriers; this chronic phase may result, after 10–20 years, in cardiopathy. Chagas disease is transmitted by several genera of triatomine bugs (Triatoma, Rhodnius and Panstrongylus) and in nature the disease exists among wild mammals and their associated triatomines. Human trypanosomiasis is seen in almost all countries of the Americas, including the southern USA, but the main foci are in poor rural areas of Latin America.
T. cruzi exhibits two cell types in vertebrate hosts, a blood form termed a trypomastigote, and in the tissues (mainly heart, skeletal and smooth muscle, and reticuloendothelial cells) the parasite occurs as an amastigote (Figure 6.3). Trypomastigotes ingested when the insect takes a blood meal from an infected host transform into epimastigotes in the intestine. Active reproduction occurs and in 8–10 days metacyclic trypomastigote forms appear which are flushed out of the gut with the faeces of the insect. These organisms are able to penetrate the vertebrate host only through the mucosa or abrasions of the skin; hence, transmission does not necessarily occur at every blood meal. Within the vertebrate the trypomastigotes transform into amastigotes, which, after a period of intracellular multiplication at the portal of entry, are released into the blood as trypanosomes; these invade other cells or tissues, becoming amastigotes again.
Figure 6.3 An infected triatomine insect vector (or ‘kissing’ bug) takes a blood meal and releases trypomastigotes in its faeces near the site of the bite wound. Trypomastigotes enter the host through the wound or through intact mucosal membranes, such as the conjunctiva (1). Common triatomine vector species for trypanosomiasis belong to the genera Triatoma, Rhodinius and Panstrongylus. Inside the host, the trypomastigotes invade cells, where they differentiate into intracellular amastigotes (2). The amastigotes multiply by binary fission (3) and differentiate into trypomastigotes, and then are released into the circulation as bloodstream trypomastigotes (4). Trypomastigotes infect cells from a variety of tissues and transform into intracellular amastigotes in new infection sites. Clinical manifestations can result from this infective cycle. The bloodstream trypomastigotes do not replicate (different from the African trypanosomes). Replication resumes only when the parasites enter another cell or are ingested by another vector. The ‘kissing’ bug becomes infected by feeding on human or animal blood that contains circulating parasites (5). The ingested trypomastigotes transform into epimastigotes in the vector’s midgut (6). The parasites multiply and differentiate in the midgut (7) and differentiate into infective metacyclic trypomastigotes in the hindgut (8). Trypanosoma cruzi can also be transmitted through blood transfusions, organ transplantation, transplacentally, and in laboratory accidents.
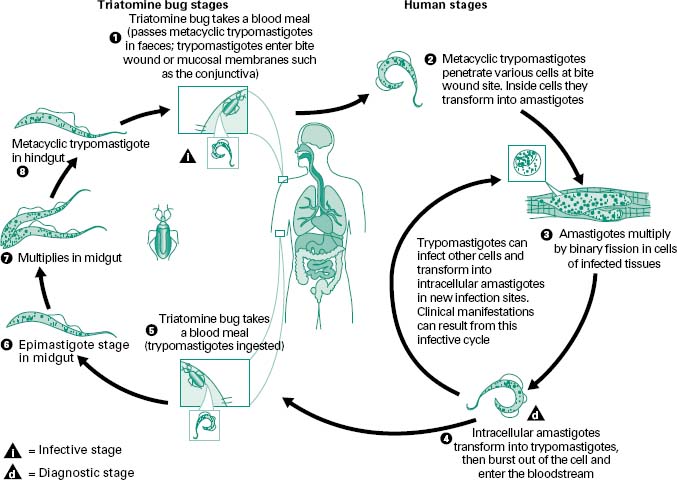
The pathology of the infection is associated with inflammatory reactions in infected tissues. These can lead to destruction of the infected tissue and if it involves heart tissue this can cause acute myocarditis. Parasite enzymes may also cause cell and tissue damage. In the absence of parasites, an autoimmune pathological process seems to be mediated by T lymphocytes (CD4 +) (see Chapter 9) and by the production of certain cytokines; these induce a polyclonal activation of B-lymphocytes and the secretion of large quantities of autoantibodies.
2.2.2 African trypanosomiasis (sleeping sickness)
Sleeping sickness (African trypanosomiasis) is caused by Trypanosoma brucei, of which there are two morphologically indistinguishable subspecies: T. brucei rhodesiense and T. brucei gambiense. After infection the parasite undergoes a period of local multiplication then enters the general circulation via the lymphatics. Recurrent fever, headache, lymphadenopathy and splenomegaly may occur. Later, signs of meningoencephalitis appear, followed by somnolence (sleeping sickness), coma and death.
T. brucei, unlike T. cruzi, multiplies in the blood or cerebrospinal fluid. Trypanosomes ingested by a feeding fly must reach the salivary glands within a few days, where they reproduce actively as epimastigotes attached to the microvilli of the salivary gland where they transform into metacyclic trypomastigotes, which are found free in the lumen. Around 15–35 days after infection the fly becomes infective through its bite.
The pathology of the infection is due to inflammatory changes associated with an induced autoimmune demyelination of nerve cells. Interestingly, the immunosuppressive action of components of the parasite’ s membrane is probably responsible for frequent secondary infections such as pneumonia. Liberation of common surface antigens (the mechanism involved in immune evasion) in every trypanolytic crisis (episode of trypanosome lysis) leads to antibody and cellmediated hypersensitivity reactions. It is believed that some cytotoxic and pathological processes are the result of biochemical and immune mechanisms.
2.2.3 Cutaneous and mucocutaneous leishmaniasis
Leishmaniasis is the term used for diseases caused by species of the genus Leishmania that are transmitted by the bite of infected sand flies. The lesions of cutaneous and mucocutaneous leishmaniasis are localized to the skin and mucous membranes. Visceral leishmaniasis is a much more severe disease, which involves the entire reticuloendothelial system, and is discussed in section 2.2.4 of this chapter. Cutaneous leishmaniasis appears 2–3 weeks after the bite of an infected sand fly as a small cutaneous papule; this slowly develops and often becomes ulcerated and develops secondary infections. Secondary or diffuse lesions may develop. The disease is usually chronic but may occasionally be self-limiting. Leishmaniasis from a primary skin lesion may involve the oral and nasopharyngeal mucosa. Leishmania species that infect humans are all morphologically similar and only exhibit one form, the intracellular amastigotes (3–6 μm long and 1.5–3μ m in diameter). Promastigotes (Figure 6.2b) are found in the sand fly.
In mammalian hosts amastigotes are phagocytosed by macrophages, but resist digestion and divide actively in the phagolysosome (Figure 6.4). The female sand fly ingests parasites in the blood meal from an infected person or animal and these pass into the stomach where they transform into promastigotes, and multiply actively. The parasites attach to the walls of the oesophagus, midgut and hindgut of the fly, and some eventually reach the proboscis and are inoculated into a new host.
Figure 6.4 Leishmaniasis is transmitted by the bite of female phlebotomine sandflies. The sandflies inject the infective stage, promastigotes, during blood meals (1). Promastigotes that reach the puncture wound are phagocytosed by macrophages (2) and transform into amastigotes (3). Amastigotes multiply in infected cells and affect different tissues, depending in part on the Leishmania species (4). This originates the clinical manifestations of leishmaniasis. Sandflies become infected during blood meals on an infected host when they ingest macrophages infected with amastigotes (5, 6). In the sandfly’s midgut, the parasites differentiate into promastigotes (7), which multiply and migrate to the proboscis (8).
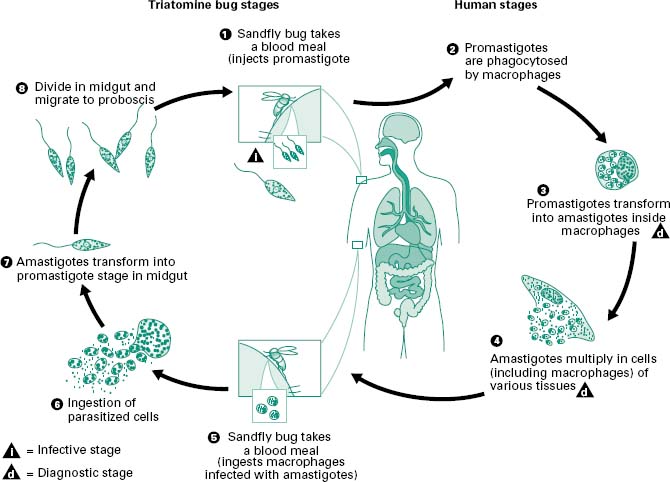
The obvious symptoms of this infection are caused by the uptake of parasites by local macrophages. Host response to infection produces tubercle-like structures designed to limit the spread of infected cells. Some lesions may resolve spontaneously after a few months but other types of lesion may become chronic, sometimes with lymphatic and bloodstream dissemination. In infections due to L. braziliensis there is a highly destructive spread of infected macrophages to the oral or nasal mucosa. In L. mexicana, L. amazonensis and L. aethiopica infections the disease becomes more disseminated. The immunological response of the host plays an important factor in determining the precise pathology of the disease and this is apparent from the more severe type of infection seen in individuals with HIV. In Europe and Africa several rodents may act as reservoirs of the disease, but in countries such as India, transmission can occur in a human–sand fly–human cycle without rodent intervention. In rural semiarid zones of Latin America, both wild and domestic dogs enter the epidemiological chain and the vector is a common sand fly, Lutzomyia longipalpis, abundant in and around houses. The disease is more common in children in both Latin America and the Mediterranean area.
2.2.4 Visceral leishmaniasis (kala-azar)
Like cutaneous leishmaniasis, visceral leishmaniasis begins with the formation of a nodule at the site of inoculation but this lesion rarely ulcerates and usually disappears in a few weeks. However, symptoms and signs of systemic disease such as undulating fever, malaise, diarrhoea, organ enlargement and anaemia subsequently develop. In more serious cases of visceral leishmaniasis the parasites, which can resist the internal body temperature, invade internal organs (liver, spleen, bone marrow and lymph nodes), where they occupy the reticuloendothelial cells. The pathogenic mechanisms of the disease are not fully understood, but enlargement occurs in those organs that exhibit marked cellular alteration such as hyperplasia. Parasitized macrophages replace tissue in the bone marrow. Patients with advanced disease are prone to superinfection with other organisms which are predominantly bacterial.
2.3 Toxoplasma gondii
The term coccidia describes a group of protozoa that contains the genus Cryptosporidium (see intestinal parasites) as well as a number of important veterinary parasites. Toxoplasma gondii is an intestinal coccidian but the major pathology of infection is associated with other tissues and organs. T. gondii infects members of the cat family as definitive hosts and has a wide range of intermediate hosts. Infection is common in many warmblooded animals, including humans. In most cases infection is asymptomatic, but devastating disease can occur congenitally in children as a result of infection during pregnancy. T. gondii infection in humans is a worldwide problem, although the rates of human infection vary from country to country. The reasons for these variations include environmental factors, cultural habits and the presence of domestic and native animal species. The frequency of postnatal toxoplasmosis acquired by eating raw meat and by ingesting food contaminated by oocysts from cat faeces (oocyst formation is greatest in the domestic cat) is not well established but is thought to be significant. Widespread natural infection is possible because infected animals may excrete millions of resistant oocysts, which can survive in the environment for prolonged periods (months-years). Mature oocysts are approximately 12 μm in diameter and contain eight infective sporozoites.
T. gondii infection in most animals including humans is asymptomatic. Severe disease in humans is observed only in congenitally infected children and in immunosuppressed individuals. The most common symptom associated with postnatal infection in humans is lymphadenitis which may be accompanied by fever, malaise, fatigue, muscle pains, sore throat and headache (flu-like symptoms). Typically infection resolves spontaneously in weeks or months, but in immunosuppressed individuals, a fatal encephalitis may occur producing symptoms such as headache, disorientation, drowsiness, hemiparesis, reflex changes and convulsions. Prenatal T. gondii infections often target the brain and retina and can cause a wide spectrum of clinical disease. Mild disease may consist of impaired vision, whereas severely diseased children may exhibit a ‘classic tetrad’ of signs: retinochoroiditis, hydrocephalus, convulsions and intracerebral calcifications. Hydrocephalus is the least common but most dramatic lesion of congenital toxoplasmosis.
The life cycle of T. gondii was only fully described in the early 1970s when felines including domestic cats were identified as the definitive host and various warmblooded animals were identified as intermediate hosts. T. gondii is transmitted by three mechanisms: congenitally, through the consumption of uncooked infected meat and via faecal matter contamination. Figure 6.5 shows the life cycle of T. gondii. Cats acquire Toxoplasma by ingesting any of three infectious stages of the organism: the rapidly multiplying forms, tachyzoites, the dormant bradyzoites (cysts) in infected tissue and the oocysts shed in faeces. The probability of infection and the time between infection and the shedding of oocysts varies with the stage of T. gondii ingested. Fewer than 50% of cats shed oocysts after ingesting tachyzoites or oocysts, whereas nearly all cats shed oocysts after ingesting bradyzoites. When a cat ingests tissue cysts, the cyst wall is dissolved by intestinal and gut proteolytic enzymes, which causes the release of bradyzoites. These enter the epithelial cells of the small intestine and initiate the formation of numerous asexual generations before the sexual cycle begins. At the same time that some bradyzoites invade the surface epithelia, other bradyzoites penetrate the lamina propria and begin to multiply as tachyzoites (trophozoites) (Figure 6.2c). Within a few hours, tachyzoites may disseminate to other tissues through the lymph and blood. Tachyzoites can enter almost any type of host cell and multiply until the cell becomes packed with parasites. The host cell then lyses and releases more tachyzoites to enter new host cells. The host usually controls this phase of infection, and as a result the parasite enters the ‘resting’ stage in which bradyzoites are isolated in tissue cysts. Tissue cysts are formed most commonly in the brain, liver and muscles. These cysts usually cause no host reaction and may remain dormant for the life of the host. In intermediate hosts, such as humans, the extraintestinal cycle of T. gondii is similar to the cycle in cats except that there is no sexual stage.
Figure 6.5 Members of the cat family (Felidae) are the only known definitive hosts for the sexual stages of Toxoplasma gondii and thus are the main reservoirs of infection. Cats become infected with T. gondii by carnivorism (1). After tissue cysts or oocysts are ingested by the cat, viable organisms are released and invade epithelial cells of the small intestine where they undergo an asexual cycle followed by a sexual cycle and then form oocysts, which are then excreted. The unsporulated oocyst takes 1-5 days after excretion to sporulate (become infective). Although cats shed oocysts for only 1-2 weeks, large numbers may be shed. Oocysts can survive in the environment for several months and are remarkably resistant to disinfectants, freezing and drying, but are killed by heating to 70 °C for 10 minutes. Human infection may be acquired in several ways: (A) ingestion of undercooked infected meat containing Toxoplasma cysts (2); (B) ingestion of the oocyst from faecally contaminated hands or food (3); (C) organ transplantation or blood transfusion; (D) transplacental transmission; (E) accidental inoculation of tachyzoites. The parasites form tissue cysts, most commonly in skeletal muscle, myocardium and brain; these cysts may remain throughout the life of the host.
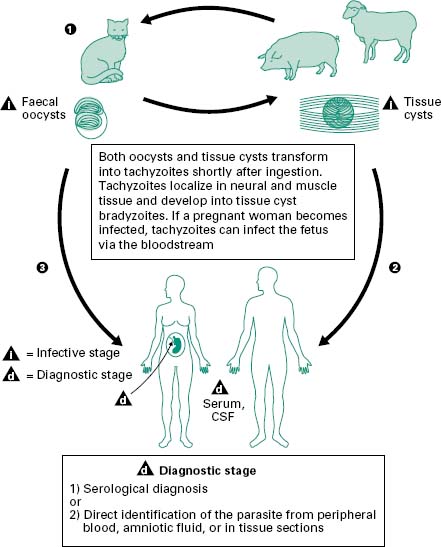
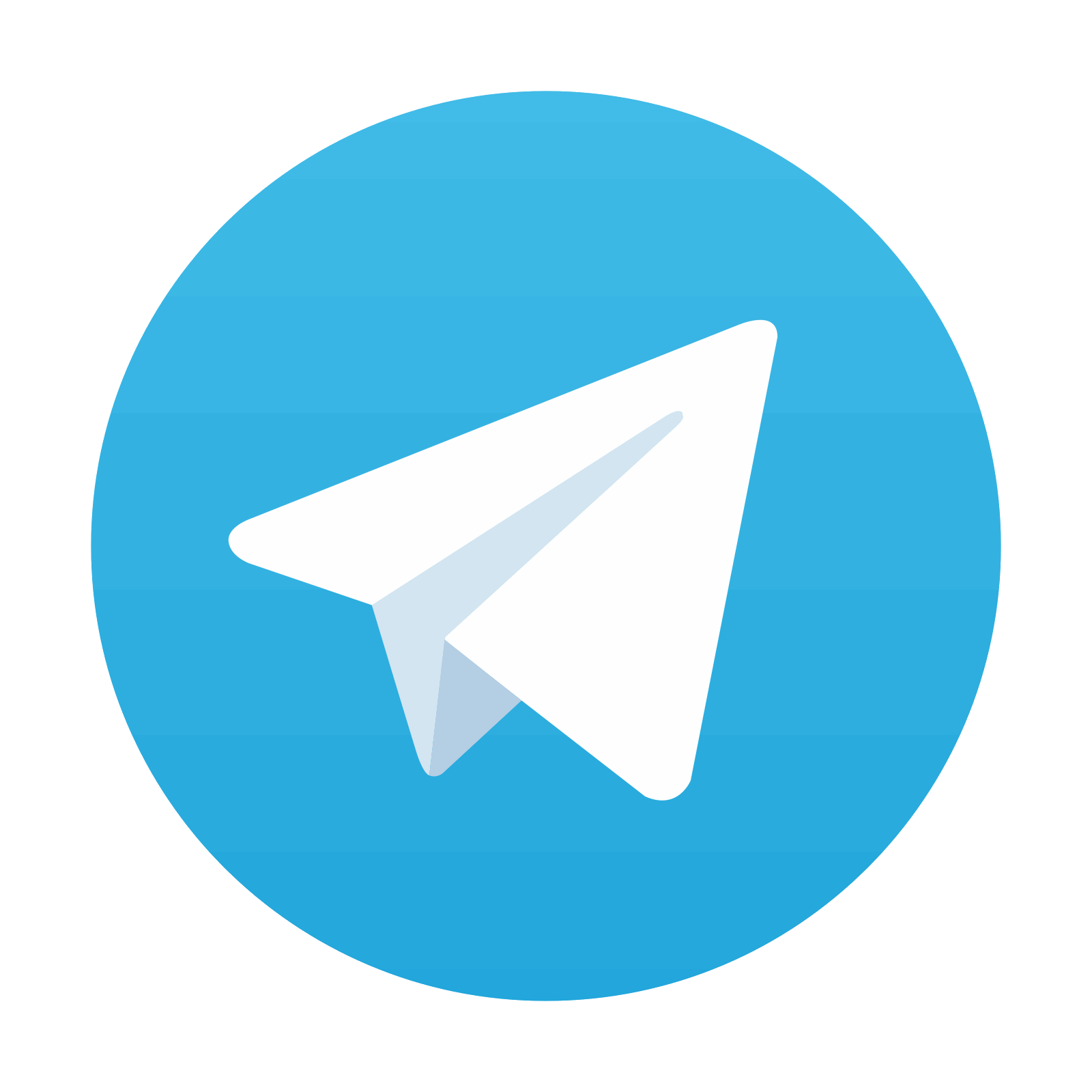