Joshua M. Galanter, Susannah B. Cornes, and Daniel H. Lowenstein
The nervous system contains more than 10 billion neurons. Most neurons form thousands of synaptic connections, giving the nervous system complexity unlike that seen in any other organ system. Interactions among neuronal circuits mediate functions ranging from primitive reflexes to language, mood, and memory. To perform these functions, the individual neurons that comprise the nervous system must be organized into functional networks, which, in turn, are organized into larger anatomical units.
The previous chapter reviewed the physiology of individual neurons by describing electrical transmission within a neuron and chemical transmission from one neuron to another. This chapter discusses neuronal systems by examining two levels of organization. First, the gross anatomical organization of the nervous system is presented to place in context the sites of action of pharmacologic agents that act on this system. Second, the major patterns of neuronal connectivity (so-called neuronal tracts) are presented, because knowledge of the ways in which neuronal cells are organized to transmit, process, and modulate signals facilitates a deeper understanding of the actions of drugs on these tracts. This chapter also discusses the major types of neurotransmitters and the blood–brain barrier; these functional and metabolic concepts have important pharmacologic consequences for drugs that act on the nervous system.
Martha P is a 66-year-old woman with a 4-year history of worsening Parkinson’s disease, a neurological disorder resulting from the progressive degeneration of nigrostriatal neurons that use dopamine as a neurotransmitter. The disease causes a resting tremor, rigidity, difficulty initiating movement, and postural instability. While visiting her physician, Ms. P registers an unusual complaint: “It seems that my Sinemet doesn’t work as well when I take it with meals.” Ms. P explains that she has recently started on a new “low-carb” diet that has increased her protein intake at the expense of high-carbohydrate foods. Concerned, Ms. P asks, “Could my diet have anything to do with this?” Her physician explains that levodopa, a component of her Sinemet, helps replace a chemical in her brain that is produced in insufficient quantities because of the loss of certain neurons in her brain. Although many factors could lead to the decreased effectiveness of her medication, Ms. P’s doctor confirms her suspicion that her high-protein diet could indeed be interfering with the medication’s ability to reach her brain. He recommends that she moderate her protein intake, and, if necessary, take a higher dose of Sinemet after a high-protein meal. At her follow-up visit, Ms. P is happy to report that her medication is more effective now that she is eating less protein.
Questions
1. Where is the nigrostriatal tract located? How does the degeneration of a specific group of neurons result in specific symptoms such as those seen in Parkinson’s disease?
2. Why is levodopa used in the treatment of Parkinson’s disease, and what is the relationship of this compound to dopamine?
3. Why does protein consumption interfere with the action of levodopa?
4. Why does Sinemet contain both levodopa and carbidopa?
The nervous system can be divided structurally and functionally into peripheral and central components. The peripheral nervous system includes all nerves traveling between the central nervous system and somatic and visceral sites. It is divided functionally into the autonomic (involuntary) nervous system and the sensory and somatic (voluntary) nervous system.
The central nervous system (CNS) includes the cerebrum, diencephalon, cerebellum, brainstem, and spinal cord. The CNS relays and processes signals received from the peripheral nervous system; the processing results in responses that are formulated and relayed back to the periphery. The CNS is responsible for important functions such as perception—including sensory, auditory, and visual processing—wakefulness, language, and consciousness.
Anatomy of the Peripheral Nervous System
The autonomic nervous system regulates involuntary responses of smooth muscle and glandular tissue. For example, it controls vascular tone, heart rate and contractility, pupillary constriction, sweating, salivation, piloerection (“goose bumps”), uterine contraction, gastrointestinal (GI) motility, and bladder function. The autonomic nervous system is divided into the sympathetic nervous system, responsible for “fight or flight” responses, and the parasympathetic nervous system, responsible for “rest and digest” responses. The sensory and somatic peripheral nervous system carries sensory signals from the periphery to the CNS and motor signals from the CNS to striated muscle; these signals regulate voluntary movement (Fig. 9-1).
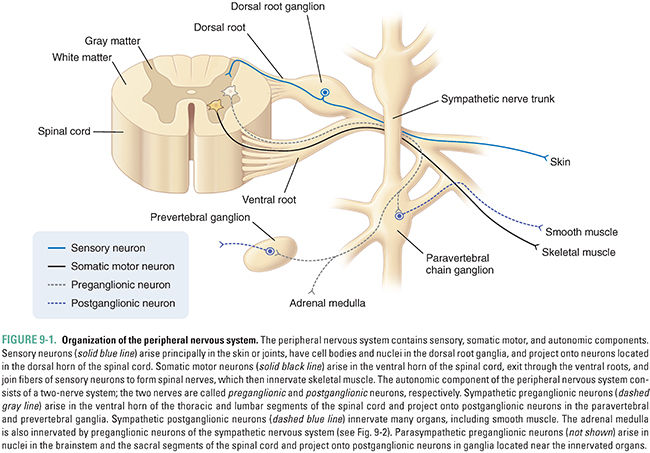
Autonomic nerve fibers interact with their target organs by a two-neuron pathway. The first neuron originates in the brainstem or spinal cord and is termed a preganglionic neuron. The preganglionic neuron synapses outside the spinal cord with a postganglionic neuron that innervates the target organ. As discussed below, the anatomical location of these connections differs for neurons of the sympathetic and parasympathetic divisions of the autonomic nervous system.
Anatomy of the Sympathetic Nervous System
The sympathetic nervous system is also known as the thoracolumbar system, because its preganglionic fibers arise from the first thoracic segment to the second or third lumbar segment of the spinal cord (Fig. 9-2). Specifically, the preganglionic nerve cell bodies arise from the intermediolateral columns in the spinal cord. Preganglionic nerves exit the spinal cord at the ventral roots of each vertebral level and make synaptic connections with postganglionic neurons in sympathetic ganglia. Most sympathetic ganglia are located in the sympathetic chain, which consists of 25 pairs of interconnected ganglia that lie on either side of the vertebral column. The first three ganglia, termed the superior cervical ganglion, middle cervical ganglion, and inferior cervical ganglion, send their postganglionic fibers via the cranial and cervical spinal nerves. The superior cervical ganglion innervates the pupil, salivary glands, and lacrimal glands, as well as blood vessels and sweat glands in the head and face (Fig. 9-2). Postganglionic neurons arising in the middle and inferior cervical ganglia, as well as the thoracic ganglia, innervate the heart and lungs. Fibers arising from the remaining paravertebral ganglia innervate sweat glands, pilomotor muscles, and blood vessels of skeletal muscle and skin throughout the body.
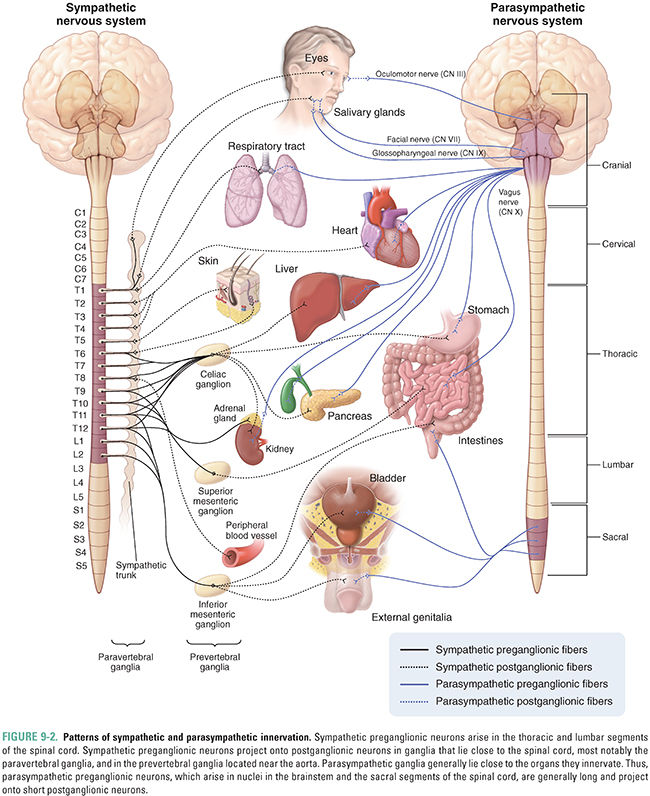
Postganglionic neurons that innervate the GI tract down to the sigmoid colon, including the liver and pancreas, arise from ganglia that are located anterior to the aorta, at the origins of the celiac, superior mesenteric, and inferior mesenteric blood vessels (Fig. 9-2). Hence, these ganglia, collectively known as prevertebral ganglia, are named the celiac ganglion, superior mesenteric ganglion, and inferior mesenteric ganglion, respectively. In contrast to the paravertebral ganglia, the prevertebral ganglia have long preganglionic fibers and short postganglionic fibers.
The adrenal medulla is contained within the adrenal glands that lie on the superior surface of the kidneys. The adrenal medulla contains postsynaptic neuroendocrine cells (Fig. 9-2). Unlike sympathetic postganglionic neurons, which synthesize and release norepinephrine, neuroendocrine cells of the adrenal medulla synthesize primarily epinephrine (85%) and release this neurotransmitter into the bloodstream rather than at synapses on a specific target organ (see Chapter 11, Adrenergic Pharmacology).
Many pharmacologic agents modulate sympathetic nervous system activity. As discussed in Chapter 11, the sympathetic nervous system has an organ-specific distribution of adrenergic receptor types. This organ-specific receptor expression allows drugs to modulate sympathetic activity selectively. For example, certain sympathetic agonists, such as albuterol, can dilate bronchioles selectively, while certain sympathetic antagonists, such as metoprolol, can selectively decrease heart rate and contractility.
Anatomy of the Parasympathetic Nervous System
Nearly all of the parasympathetic ganglia lie in or near the organs they innervate. The preganglionic fibers of the parasympathetic nervous system arise in the brainstem or in sacral segments of the spinal cord; thus, the parasympathetic system is also called the craniosacral system (Fig. 9-2). In some cases, parasympathetic preganglionic neurons can travel almost 1 meter before synapsing with their postganglionic targets. Preganglionic nerve fibers of cranial nerve (CN) III, the oculomotor nerve, arise from a region of the midbrain termed the Edinger-Westphal nucleus and innervate the pupil, stimulating it to constrict. The medulla of the brain contains nuclei for parasympathetic nerve fibers in CNs VII, IX, and X. Parasympathetic fibers in the facial nerve (CN VII) stimulate salivary secretion by the submaxillary and sublingual glands as well as tear production by the lacrimal gland. Parasympathetic fibers in the ninth cranial nerve, the glossopharyngeal nerve, stimulate the parotid gland. The 10th cranial nerve, termed the vagus nerve, provides parasympathetic innervation to the major organs in the chest and abdomen, including the heart, tracheobronchial tree, kidneys, and GI system down to the proximal colon. Parasympathetic nerves originating in the sacral region of the spinal cord innervate the remainder of the colon, urinary bladder, and genitalia.
Many pharmacologic agents modulate parasympathetic nervous system activity. For example, bethanechol is a parasympathomimetic that promotes GI and urinary tract motility.
Antagonists of parasympathetic activity include atropine, a drug used locally to dilate the pupils or systematically to increase heart rate, and ipratropium, a drug used to dilate bronchioles. These agents and others are discussed in Chapter 10, Cholinergic Pharmacology.
Peripheral Motor and Sensory Systems
Fibers of the somatic nervous system innervate their target striated muscles directly (Fig. 9-1). The first-order neurons from the motor cortex send projections that cross in the lower medulla and descend through the spinal cord in the lateral corticospinal tract before synapsing on the second-order neurons in the ventral horns of the spinal cord. Projections from the second-order neurons exit through the ventral roots and join the dorsal roots, carrying sensory nerve fibers, to form the spinal nerves. Spinal nerves exit the vertebral column through the intervertebral foramina, after which they separate into peripheral nerves. Somatic components of the peripheral nerves innervate muscles directly. Muscles are innervated in a myotomal distribution. That is, neurons originating from a particular ventral root level of the spinal cord (e.g., C6) innervate specific muscles (e.g., flexor muscles of the forearm).
Sensory neurons have cell bodies in the dorsal root ganglia. The endings of sensory nerves lie in the skin and joints and enter the spinal cord through the dorsal roots. Neurons for vibration and position sense (proprioception) ascend through the ipsilateral dorsal columns in the spinal cord and synapse with secondary neurons in the contralateral lower medulla. Sensory neurons that carry sensations of pain and temperature synapse with secondary neurons in the posterior horn of the spinal cord and then cross within the spinal cord to ascend in the contralateral spinothalamic tract. Both the spinothalamic tract and the dorsal column tracts connect with third-order neurons in the thalamus, part of the diencephalon (see below), before ultimately reaching the somatosensory cortex. Sensory information is encoded in a dermatomal distribution. That is, neurons originating from a particular dorsal root level of the spinal cord (e.g., C6) carry sensory information corresponding to a particular area of the skin (e.g., the lateral aspects of the forearm and hand).
A number of pharmacologic agents modulate the activity of the somatic nervous system. For example, antagonists of neuromuscular junction activity, such as pancuronium, are used to induce paralysis during surgery. In contrast, drugs that increase neuromuscular junction activity, such as edrophonium and neostigmine, are used in the diagnosis and treatment of myasthenia gravis, an autoimmune disease characterized by decreased skeletal muscle stimulation at the neuromuscular junction. These agents and others are discussed in Chapter 10.
Anatomy of the Central Nervous System
The CNS is divided anatomically into seven major divisions, namely, the cerebral hemispheres, diencephalon, cerebellum, midbrain, pons, medulla, and spinal cord (Fig. 9-3). The midbrain, pons, and medulla are collectively known as the brainstem and together connect the spinal cord with the cerebrum, diencephalon, and cerebellum.
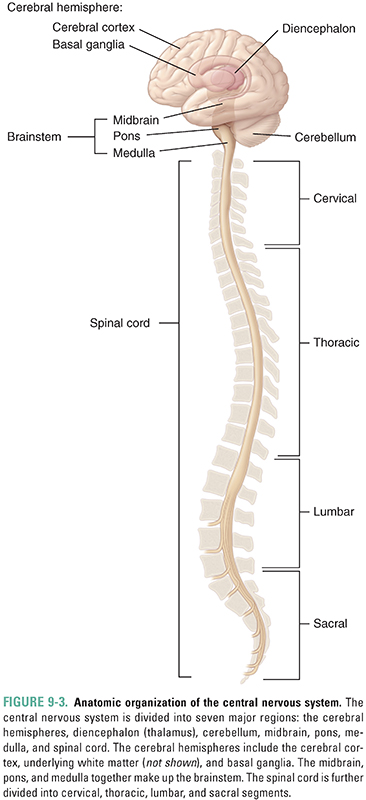
The cerebral hemispheres constitute the largest division of the human brain. These structures contain several subdivisions, including the cerebral cortex, its underlying white matter, and the basal ganglia (Fig. 9-4). The cerebral hemispheres are divided into left and right sides that are connected by the corpus callosum. The cerebral cortex is responsible for high-level functions, including sensory perception, planning and ordering motor functions, cognitive functions, such as abstract reasoning, and language. The cortex is divided anatomically and functionally into the frontal, temporal, parietal, and occipital lobes (Fig. 9-4A). Subregions of the cortex have specific functions. For example, stimulation of part of the precentral gyrus, which lies in the frontal cortex, induces peripheral motor function (movement), and ablation of this structure inhibits movement. From a pharmacologic perspective, the cerebral cortex is a site of action of many drugs, sometimes as part of their intended mechanism of action and sometimes as an adverse effect. Barbiturates and benzodiazepines (see Chapter 13, Pharmacology of GABAergic and Glutamatergic Neurotransmission) are commonly prescribed hypnotics and sedatives that potentiate the action of inhibitory neurotransmitters in the cortex. General anesthetics (see Chapter 17, General Anesthetic Pharmacology) are also thought to have effects on the cerebral cortex.
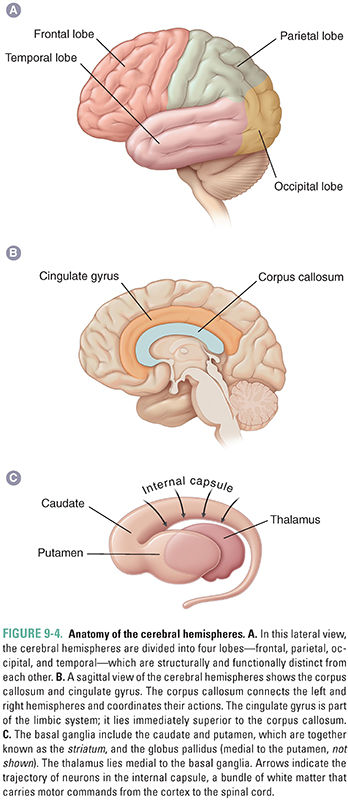
The cerebral white matter, which includes the corpus callosum (Fig. 9-4B), transmits signals between the cortex and other areas of the central nervous system and from one area of the cortex to another. The white matter consists primarily of myelinated axons that, as in other areas of the brain, have an associated vascular network of small arteries, veins, and capillaries. It is around these small vessels that inflammatory cells collect in diseases such as multiple sclerosis, and it is the small arterioles that are especially affected by systemic hypertension.
The basal ganglia consist of three deep nuclei of gray matter (Fig. 9-4C), including the caudate and putamen—together known as the striatum—and the globus pallidus. In a general sense, these nuclei help initiate and control cortical actions. These actions include not only intended movement but also behavior and certain rudimentary aspects of cognition. Regions of the basal ganglia responsible for movement ensure that intended actions are carried out and irrelevant movements are inhibited. As seen in the case of Ms. P, Parkinson’s disease is caused by degeneration of a dopaminergic pathway that arises in the substantia nigra in the midbrain (see below) and terminates in the striatum (hence its name, the nigrostriatal tract or pathway). This degeneration prevents the basal ganglia from properly initiating motor activity—resulting in decreased intended movement and an unintended tremor—and causes the decreased (“flat”) affect characteristic of Parkinson’s disease. Levodopa, a component of Ms. P’s Sinemet medication, acts on the striatum to ameliorate these clinical manifestations of the disease (see Chapter 14, Pharmacology of Dopaminergic Neurotransmission).
A rim or “limbus” around the cortex has “older,” more basic functions and is loosely termed the limbic system. This system consists of the cingulate gyrus (Fig. 9-4B), the hippocampal formation (including the hippocampus and surrounding structures), and the amygdala. These structures are responsible for emotion, social behavior, autonomic control, the perception of pain, and memory. For example, the memory loss associated with Alzheimer’s disease is caused by degeneration of the hippocampal formation. Only a few drugs that specifically affect the limbic system are currently available, although many agents affecting this region of the brain are in development. It should be noted that many drugs of abuse (see Chapter 19, Pharmacology of Drugs of Abuse) stimulate the brain reward pathway, which includes the nucleus accumbens and its projections to the limbic system.
The diencephalon is divided into the thalamus and hypothalamus. The thalamus, which has several distinct nuclei, is located medially in the brain and inferior to the cerebral cortex. Some thalamic nuclei link sensory pathways from the periphery to the cerebral cortex. Other nuclei act as connections between the basal ganglia and the cortex. The thalamus is not a simple signal relay; rather, it filters and modulates sensory information, in part dictating which signals reach conscious awareness.
The hypothalamus lies ventral to the thalamus. It controls the autonomic nervous system, the pituitary gland, and essential behaviors such as hunger and thermoregulation. Descending pathways from the medial hypothalamus regulate autonomic preganglionic neurons in the medulla and spinal cord. It is generally believed that the antihypertensive effect of clonidine is mediated by its action at receptors on brainstem neurons controlled by the hypothalamus (see Chapter 11). Other neurons originating in the medial hypothalamus secrete hormones either directly into the systemic circulation (e.g., vasopressin from axon terminals in the posterior pituitary gland) or into a portal system that, in turn, controls hormone secretion by the anterior pituitary gland (see Chapter 27, Pharmacology of the Hypothalamus and Pituitary Gland). The hypothalamus also initiates complex behaviors in response to hunger, extremes in temperature, thirst, and time of day.
The cerebellum lies inferior to the posterior end of the cerebrum and dorsal to the brainstem. It has three functionally distinct regions: the central cerebellar vermis, the lateral cerebellar hemispheres, and the small flocculonodular lobe (Fig. 9-5). The cerebellum has a relatively well-defined pattern of neural connections, receiving inputs from a wide variety of sources and sending output primarily to the motor areas of the cerebral cortex via the thalamus. The cerebellum coordinates voluntary movement in space and time, maintains balance, controls eye movement, and has roles in motor learning (for example, hand–eye coordination) and certain cognitive functions such as the timing of repetitive events and language. Few drugs are designed primarily to affect the cerebellum. However, several agents, notably alcohol and certain antiepileptic drugs, are toxic to the cerebellum. These agents especially affect the vermis, which controls balance.
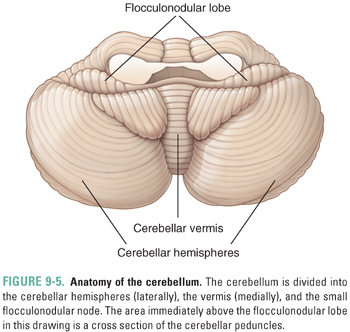
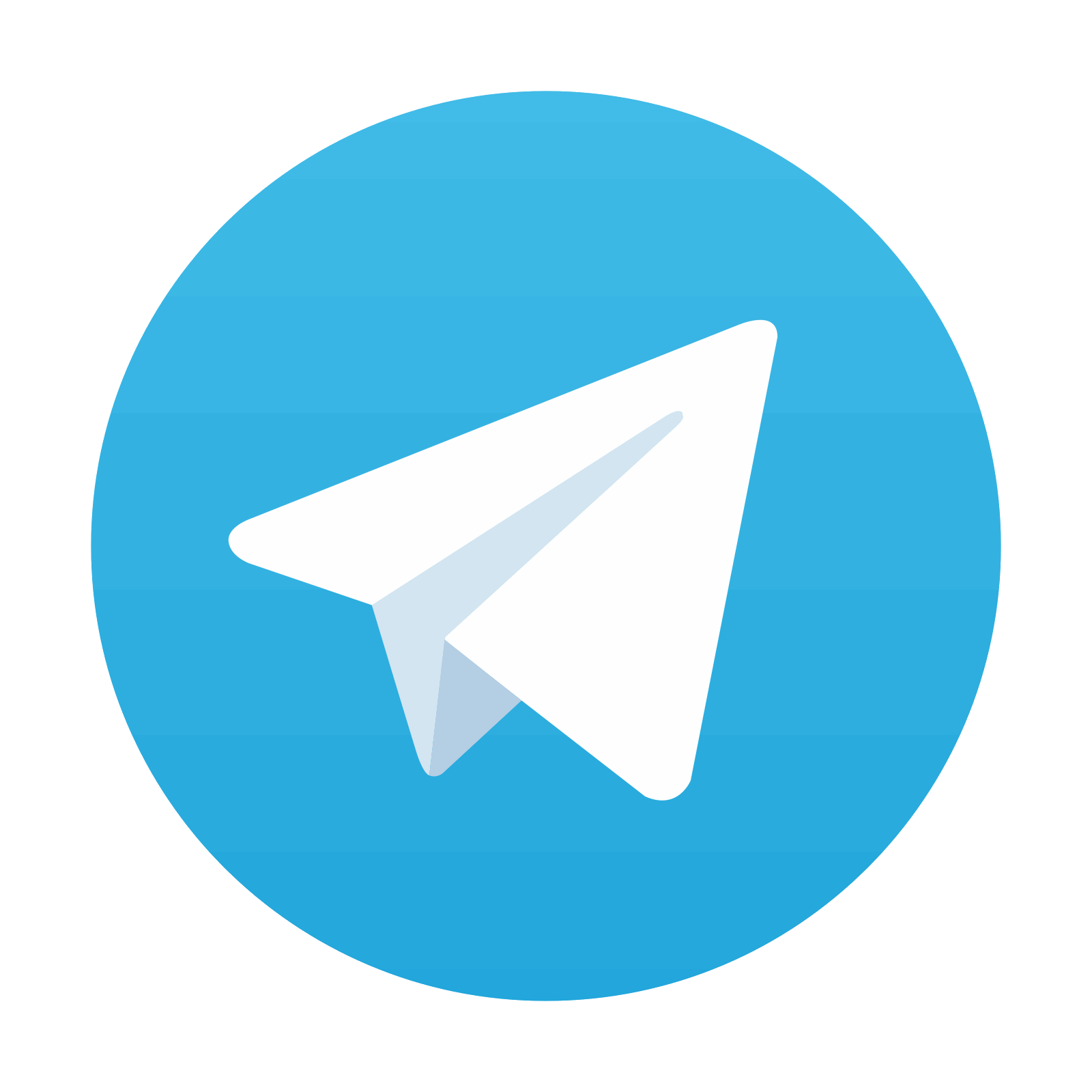
Stay updated, free articles. Join our Telegram channel

Full access? Get Clinical Tree
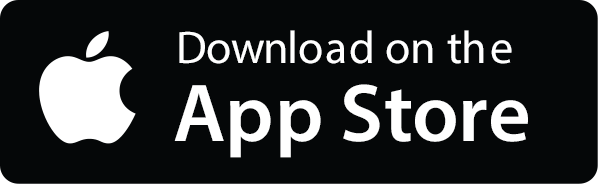
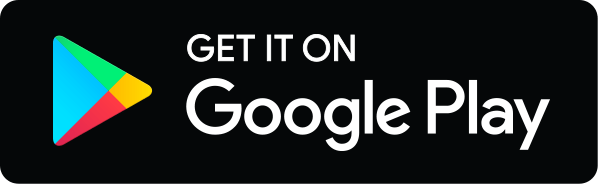