Anand Vaidya and Ursula B. Kaiser
PHYSIOLOGY, PATHOPHYSIOLOGY, AND PHARMACOLOGY OF INDIVIDUAL AXES |
The hypothalamus and pituitary gland function cooperatively as master regulators of the endocrine system. Together, hormones secreted by the hypothalamus and pituitary gland control important homeostatic and metabolic functions, including reproduction, growth, lactation, thyroid and adrenal gland physiology, and water homeostasis. This chapter introduces the physiology and regulation of hypothalamic and pituitary hormones through a discussion of feedback regulation and the various axes of hormonal regulation. The pharmacologic utility of hypothalamic and pituitary factors is then discussed, with emphasis on the regulation of specific endocrine pathways. Three concepts are of special importance in this chapter: (1) hypothalamic control of pituitary hormone release, (2) negative feedback inhibition, and (3) endocrine axes. A thorough understanding of these pathways and their mechanisms provides the foundation for understanding the use of pharmacotherapy to modulate the hypothalamic-pituitary axes.
GR is a 42-year-old sales executive. She travels constantly and prides herself on being energetic and surpassing sales projections each quarter. Three years ago, she developed irregular menses, and she then completely stopped menstruating. Over the past 2 years, she has begun to feel increasingly fatigued, has difficulty rushing the length of airport terminals, and is bothered by frequent headaches. She has always had a firm handshake, but lately, she has also noticed that her wedding ring is excessively tight. GR is also frustrated that she has recently had to replace her entire shoe collection because her shoe size has increased from 7½ to 9, with a need for increased width as well. Additionally, she has noticed increased perspiration, even when she is not exerting herself, and increased spacing between her teeth. Concerned about her progressive cosmetic changes and her lack of menses, GR turns to Internet searches for more information and comes across a condition called acromegaly.
Struck by the uncanny resemblances between her complaints and those she has read about on the Internet, GR arranges to see her doctor for further evaluation. A serum insulin-like growth factor (IGF-1) level is significantly elevated after correction for GR’s age and gender, and her serum growth hormone level is 10 ng/mL (normal, <1 ng/mL) after an oral glucose load of 75 mg. A magnetic resonance imaging (MRI) study of her head reveals a pituitary adenoma with maximal diameter of 1.5 cm. These findings are consistent with a diagnosis of acromegaly due to a growth hormone-secreting adenoma. After referral to an endocrinologist and neurosurgeon, GR elects to undergo transsphenoidal pituitary surgery. GR tolerates the surgery well, but her postoperative growth hormone and IGF-1 levels remain elevated.
Based on the continued elevation in serum growth hormone and IGF-1 levels, GR’s endocrinologist recommends medical treatment with octreotide. GR tolerates the thrice-daily injections well, except for occasional mild nausea. After 2 weeks of frequent injections, GR switches to a long-acting, depot form of octreotide that is injected once a month. GR is much happier with the reduced frequency of drug administration, although she continues to experience mild nausea and bloating as adverse effects of this medication.
After 6 months of depot octreotide injections, GR’s growth hormone and IGF-1 levels remain elevated. GR is frustrated at the lack of improvement of her biochemical assays but does feel that she has more energy than before treatment, and her menses have resumed. GR’s endocrinologist recommends treatment with pegvisomant as an alternative medical approach to treating the effects of her elevated growth hormone levels. GR begins daily injections with pegvisomant. Six months later, GR’s IGF-1 level is in the normal range. GR is again flying around the nation in pursuit of increased sales, and she stops in town just long enough to complete her yearly head MRI and liver function tests.
Questions
1. Why was it necessary for GR to receive injections of octreotide and pegvisomant rather than taking the drugs orally?
2. Why are serum levels of IGF-1 a more appropriate screening test for acromegaly than growth hormone levels?
3. What anatomical and hormonal considerations are raised by the abrupt cessation of normal menstruation?
4. How do octreotide and pegvisomant act to lower IGF-1 levels?
HYPOTHALAMIC AND PITUITARY PHYSIOLOGY
Relationship Between the Hypothalamus and Pituitary Gland
From a developmental perspective, the pituitary gland consists of two closely associated organs. The anterior pituitary (adenohypophysis) is derived from ectodermal tissue. The posterior pituitary (neurohypophysis) is a neural structure derived from the ventral surface of the diencephalon. The prefixes adeno- and neuro- denote the oral ectodermal and neural ectodermal origin of the anterior and posterior pituitary gland components, respectively. An intermediate lobe also exists in most mammals but is vestigial in humans.
Although the anterior and posterior pituitary glands derive from different embryologic origins, the hypothalamus controls the activity of both lobes. The connection between hypothalamus and pituitary gland is one of the most important points of interaction between the nervous and endocrine systems. The hypothalamus acts as a neuroendocrine transducer by integrating neural signals from the brain and converting those signals into chemical messages (largely peptides) that regulate the secretion of pituitary hormones. In turn, the pituitary hormones alter the activities of peripheral endocrine organs.
Hypothalamic control of the anterior pituitary gland occurs via hypothalamic secretion of hormones into the hypothalamic-pituitary portal vascular system (Fig. 27-1). The initial capillary bed of this portal system is formed from branches of the superior hypophyseal artery that fan around the axon terminals of hypothalamic neurons. Endothelial fenestrations in this capillary bed allow hypothalamic factors to be released into the bloodstream. These capillaries then coalesce into short veins that extend to the anterior pituitary gland. Upon arriving at the anterior pituitary, the veins branch into a second capillary bed and bathe the endocrine cells of the anterior pituitary gland with hormones secreted by the hypothalamus.
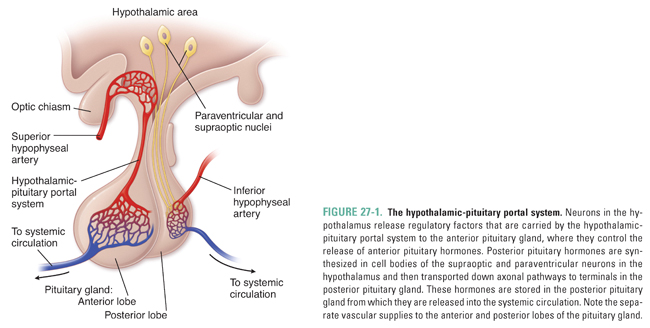
In contrast to the indirect vascular connection between the hypothalamus and the anterior pituitary gland, a direct neural connection exists between the hypothalamus and the posterior pituitary gland. Neurons synthesize hormones, destined for storage in the posterior pituitary gland, in cell bodies of the hypothalamic supraoptic and paraventricular nuclei. These hormones are then transported down axons to the posterior pituitary gland, where they are stored in neuronal terminals until a release stimulus occurs. The posterior pituitary gland can, therefore, be thought of as an extension of the hypothalamus. As with the anterior pituitary gland, the fenestrated endothelial cells in a capillary bed surrounding the posterior pituitary gland, in this case arising from the inferior hypophyseal artery, facilitate release of hormones into the systemic circulation.
During development and proliferation, the fate of anterior pituitary gland cells is determined by a network of transcription factors that shepherd the terminal differentiation of these cells into thyrotrophs, corticotrophs, lactotrophs, somatotrophs, and gonadotrophs. Three examples of transcription factors that are instrumental in anterior pituitary cell development are Pit-1, T-Pit, and Prophet of Pit-1 (Prop-1).
The anterior pituitary gland is a heterogeneous collection of numerous cell types, each of which has the capacity to respond to specific stimuli and to release specific hormones into the systemic circulation. Each of the several hypothalamic releasing or inhibiting factors alters the hormone secretion pattern of one or more anterior pituitary gland cell types (Table 27-1). Releasing factors also modify other cellular processes in the anterior pituitary gland, including hormone synthesis and pituitary cell growth. Interestingly, the relationship between hypothalamic releasing factors and pituitary gland hormones is not always 1:1, nor is the interaction always stimulatory. Somatostatin, for example, primarily inhibits the release of growth hormone (GH), but it can also inhibit release of thyroid-stimulating hormone (TSH) and prolactin. Conversely, thyrotropin-releasing hormone (TRH) primarily stimulates the release of TSH, but it can also cause release of prolactin. The overlapping activities of some releasing factors and release-inhibiting factors, together with the antagonistic actions of some stimulatory and inhibitory hypothalamic factors, provide a mechanism for the precise regulation of secretory pathways.
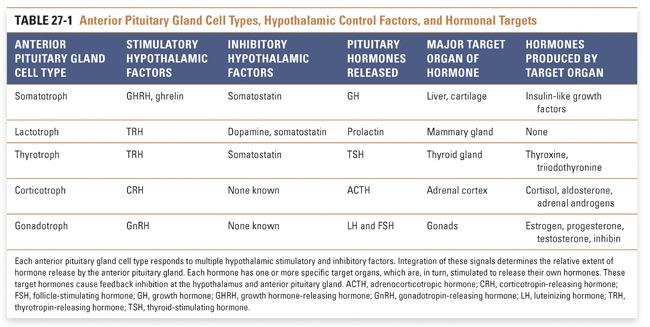
With the exception of dopamine, all known hypothalamic releasing factors are peptides. The anterior pituitary gland hormones are proteins and glycoproteins. Anterior pituitary gland hormones fall into three groups. Somatotropic hormones—growth hormone (GH) and prolactin—are 191 and 198 amino acids long, respectively, and exist as monomeric proteins that share significant structural homology. Glycoprotein hormones—luteinizing hormone (LH), follicle-stimulating hormone (FSH), and thyroid-stimulating hormone (TSH)—are heterodimeric proteins with carbohydrates attached to certain residues. These three hormones share the same homologous α subunit, which is also shared by the human chorionic gonadotropin (hCG) hormone, but each has a unique β subunit that confers biological specificity. Adrenocorticotropic hormone (ACTH) belongs to a separate class, as it is processed by proteolysis from a larger precursor protein. Of importance, intact peptides and proteins are not absorbed across the intestinal lumen; local proteases digest them into their constituent amino acids. For this reason, therapeutic administration of a peptide hormone or hormone antagonist must be accomplished by a non-oral route—this is why, in the introductory case, it was necessary for GR to take octreotide and pegvisomant by injection.
The response of an anterior pituitary gland cell to a hypothalamic factor is initiated when the hypothalamic factor binds to specific G protein-coupled receptors located on the plasma membrane of the appropriate anterior pituitary cell type. Most of these receptors alter the levels of either intracellular cyclic adenosine monophosphate (cAMP), or inositol 1,4,5-trisphosphate (IP3) and calcium (Ca2+) (see Chapter 1, Drug–Receptor Interactions). The molecular details of receptor signaling provide a basis for understanding hypothalamic factor action. For example, growth hormone-releasing hormone (GHRH) binding to its receptors on somatotrophs increases intracellular cAMP and Ca2+ levels, whereas somatostatin binding to its receptors on somatotrophs decreases intracellular cAMP and Ca2+. These signaling pathways provide a biochemical explanation for the opposing activities of GHRH and somatostatin on somatotroph release of GH.
The timing and pattern of hypothalamic factor release are important determinants of anterior pituitary cell response. Most hypothalamic releasing factors are secreted in a cyclical or pulsatile, rather than continuous, manner. For example, the hypothalamus releases pulses of gonadotropin-releasing hormone (GnRH) with a periodicity of a few hours. The frequency and magnitude of GnRH release determine the extent of pituitary gonadotropin release as well as the ratio of LH secretion to FSH secretion. Interestingly, continuous administration of GnRH suppresses rather than stimulates pituitary gonadotroph activity. These different pharmacologic effects of GnRH—depending on the frequency and pattern of administration—have important clinical consequences, as discussed below. Although not studied in as much detail, the majority of the other hypothalamic releasing factors are also thought to be secreted in a pulsatile manner.
End-product inhibition tightly controls hypothalamic and pituitary gland hormone release. For each hypothalamic-pituitary-target organ system, an integrated picture can be constructed of how each set of hormones affects the system. Each pathway, including one or more hypothalamic factors, its pituitary gland target cell type, and the ultimate target gland(s), is referred to as an endocrine axis; the term axis is used to connote one of multiple homeostatic systems that the hypothalamus and pituitary gland control. A simplified model consists of five endocrine axes, with a single type of anterior pituitary gland cell at the center of each axis (see Table 27-1 for anterior pituitary gland cell types).
Each axis regulates an important aspect of endocrine homeostasis and is, therefore, subject to close regulation. Feedback inhibition is usually discussed in terms of loops, because the regulatory connection between a given hormone and its target creates a “loop” that alters the subsequent extent of hormone release. These feedback loops closely regulate the hypothalamic-pituitary axes by providing levels of control at each stage of action (Fig. 27-2). In general, systemic hormones produced by target organs negatively regulate the pituitary and hypothalamus to maintain an equilibrium level of hormone release.
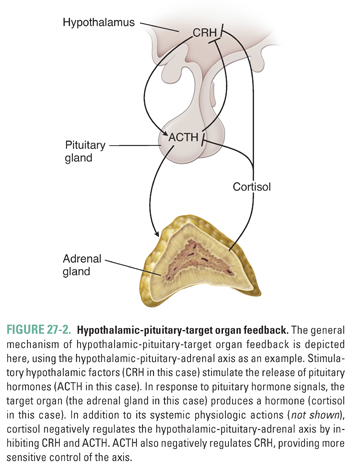
Just as regulatory loops are referred to based on a hormone’s relationship to its target organ, many endocrine diseases are described based on whether the disease etiology is a disorder of the hypothalamus, pituitary gland, or target organ. The disease is referred to as primary, secondary, or tertiary, depending on whether the underlying abnormality is in the target organ, pituitary gland, or hypothalamus, respectively. Therefore, a primary endocrine disorder is caused by target organ pathology, a secondary disorder reflects pituitary disease, and a tertiary endocrine disorder results from hypothalamic pathology. Whether the underlying disease cause is primary, secondary, or tertiary can have important consequences for disease diagnosis and treatment, as discussed below.
PHYSIOLOGY, PATHOPHYSIOLOGY, AND PHARMACOLOGY OF INDIVIDUAL AXES
Hypothalamic-Pituitary-Growth Hormone Axis
The hypothalamic-pituitary-growth hormone axis regulates general processes that promote growth. Somatotrophs of the anterior pituitary gland produce and secrete growth hormone. GH is first expressed at high concentrations during puberty; it is secreted in a striking pulsatile manner, with the largest pulses usually occurring at night during sleep. Most of the anabolic effects of GH are mediated by insulin-like growth factors, especially insulin-like growth factor 1 (IGF-1), a hormone released into the circulation by hepatocytes in response to stimulation by GH. Although several cell types are capable of producing IGF-1, hepatocytes contribute the overwhelming majority of detectable IGF-1 in the circulation. Unlike GH, which has a short circulating half-life and a pulsatile pattern of secretion, IGF-1 is protein-bound and stable in the circulation for longer periods of time at steady concentrations. Thus, IGF-1 measurements represent an integrated surrogate for GH activity that is stable throughout the day, and IGF-1 levels are a more appropriate tool than GH levels in screening for acromegaly (as in the introductory case).
Several environmental and biological stimuli regulate GH secretion. Environmental factors such as hypoglycemia, sleep, exercise, and adequate nutritional status can all increase GH secretion. Endogenous biological inputs that promote GH release include hypothalamic GHRH, sex steroids (most notably during puberty), dopamine, and ghrelin. Ghrelin is an important endogenous growth hormone-releasing peptide that has been identified and well characterized in the last decade. Ghrelin acts synergistically with GHRH to promote GH release, acting on a receptor that is distinct from the GHRH receptor. The majority of ghrelin is secreted by gastric fundal cells during the fasting state, linking growth with nutritional status and energy balance. Nonpeptide, orally active ghrelin mimetics are currently under clinical investigation as GH secretagogues, and antagonists are being studied for appetite control. Several environmental factors inhibit GH release, including hyperglycemia, sleep deprivation, and poor nutritional status. The most significant endogenous biological factors that inhibit GH secretion are somatostatin, IGF-1, and GH.
Pathophysiology and Pharmacology of Growth Hormone Deficiency
Failure to secrete growth hormone or to enhance IGF-1 secretion during puberty results in growth retardation (Fig. 27-3A–D). GH deficiency most commonly results from defective hypothalamic release of GHRH (tertiary deficiency, Fig. 27-3D) or from pituitary insufficiency (secondary deficiency, Fig. 27-3C). Importantly, however, failure of IGF-1 secretion in response to GH (Laron dwarfism or primary deficiency, Fig. 27-3B) is one etiology of short stature that is not amenable to treatment with GH. Sermorelin (synthetic GHRH) can be administered parenterally to help determine the disease etiology. Tesamorelin, a novel GHRH analogue, has also been shown to augment basal and pulsatile GH secretion and has been used in the treatment of HIV-associated lipodystrophy. If a patient possesses defective hypothalamic release of GHRH but has normally functioning anterior pituitary gland somatotrophs, administration of exogenous GHRH results in increased GH release. As of 2008, sermorelin has become unavailable in the United States due to discontinuation of industrial manufacturing. Alternative exogenous agents currently used to stimulate GH release include glucagon, arginine, clonidine, and insulin–induced hypoglycemia.
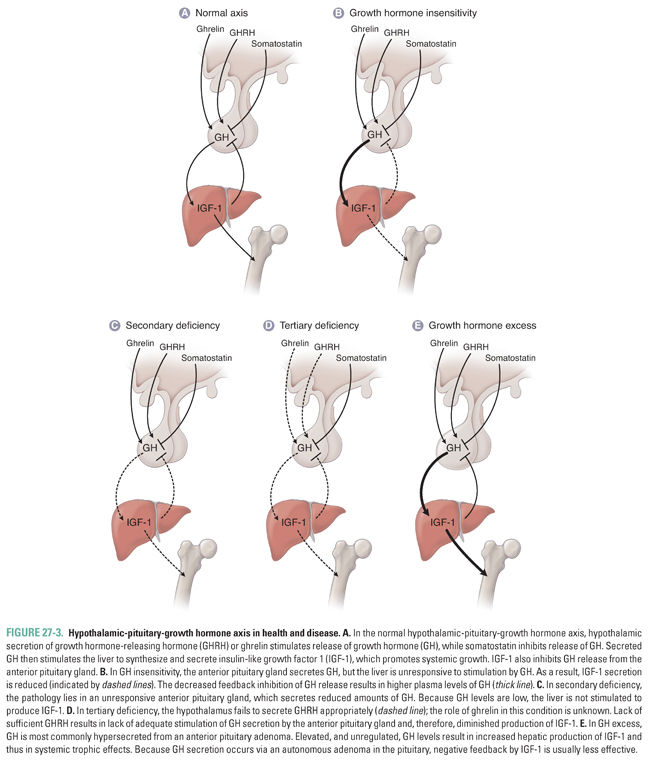
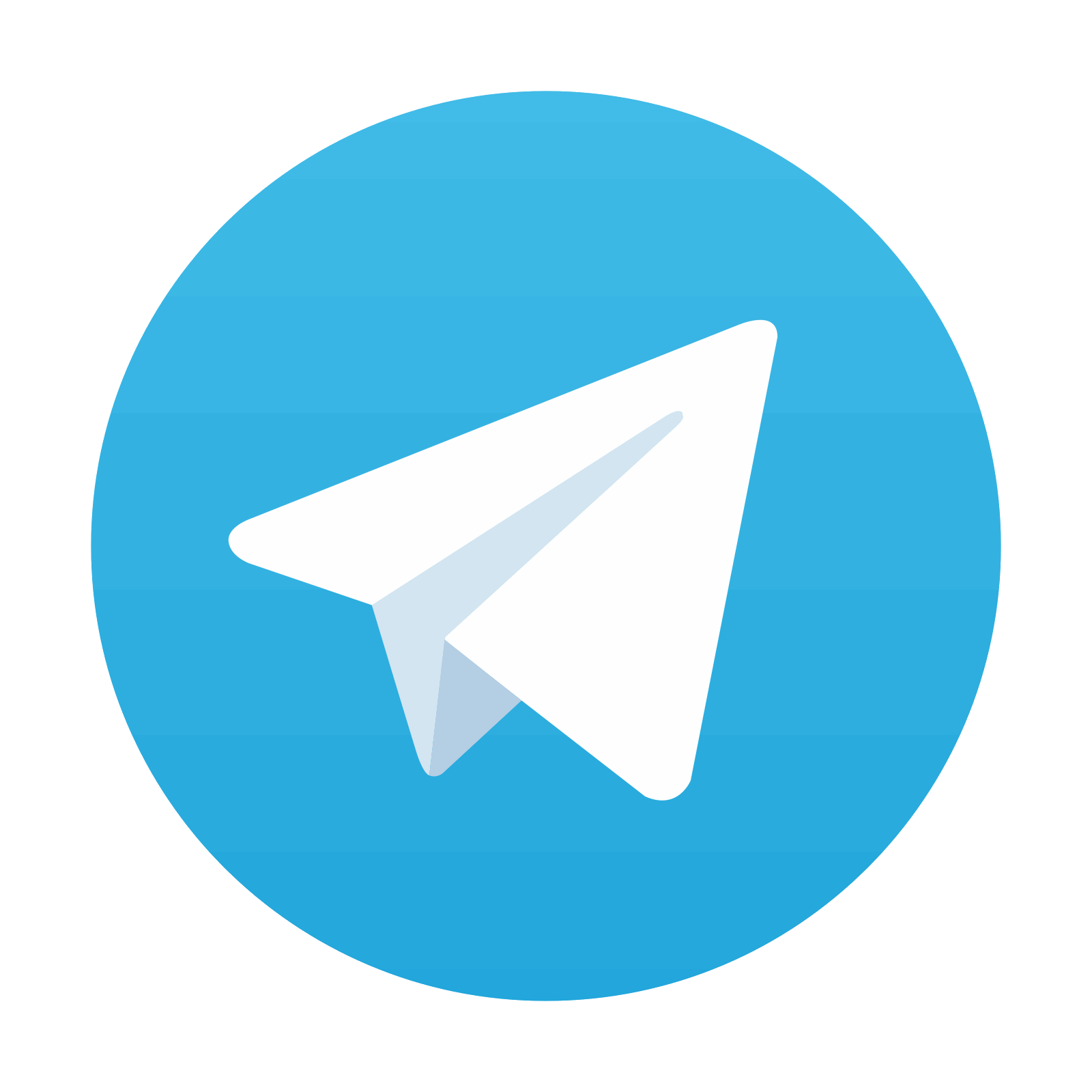
Stay updated, free articles. Join our Telegram channel

Full access? Get Clinical Tree
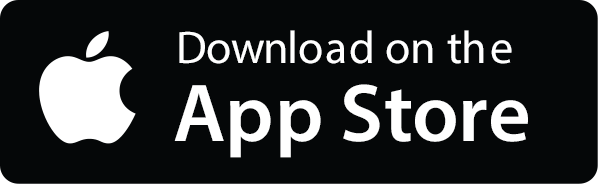
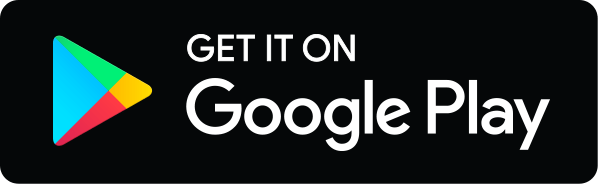