Figure 5.1Sorption isotherm data (absorption and desorption) of gelatin and HPMC (Vcaps® Plus) capsules stored at different RH values. (Courtesy of Capsugel.)
The mechanical resistance and stiffness of two-piece capsules are important for its machinability and robustness in processing and handling. The mechanical resistance is dependent on the moisture content of the capsules (see Figure 5.2) and softening is observed with increasing moisture content in both types of capsules. In general, HPMC capsules tend to be less strong and more elastic compared to gelatin capsules, especially at higher moisture levels (Kuentz et al. 2006; Missaghi and Fassihi 2006). However, under dry conditions, HPMC capsules are more resistant to breakage compared to gelatin capsules and remain sufficiently flexible even at very low humidity (below 30% RH) where a gradual increase of breakage under mechanical stress conditions is observed for gelatin capsules (Ku et al. 2010). Because of this characteristic, HPMC capsules are used for products that are sensitive to moisture or which require low moisture levels during processing or storage.

Figure 5.2Mechanical properties of HPMC capsules (Vcaps® Plus) and gelatin capsules measured by the tube test method after storage at different RH values. (From Ku MS, Lu Q, Li W et al. Performance qualification of a new hypromellose capsule: Part II. Disintegration and dissolution comparison between two types of hypromellose capsules. Int J Pharm 2011; 416: 16–24.)
Despite the slightly higher elasticity and lower strength of HPMC capsules, when compared with gelatin capsules, HPMC capsules have a similar performance on high-speed filling machines. However, tests in which the different capsule types were run on filling machines from laboratory to commercial scale and at different machine speeds revealed that capsules manufactured by thermo-gelation have a superior performance compared to HPMC capsules containing a gelling system (Ku et al. 2010).
Accelerated stability testing has in addition shown that HPMC capsules are more inert in and resistant to residual solvents, higher temperature storage, and higher humidity conditions. In these tests, exposure of HPMC capsules to a 25-ppm (parts per million) aldehyde containing lactose blend for 1 week did not change the dissolution profile of capsules. Short-term storage at a high temperature of up to 90°C had no impact on the mechanical properties of the HPMC capsules or their disintegration and dissolution performance (Ku et al. 2010).
Dissolution and Disintegration
Capsule rupture and disintegration as well as dissolution of the empty capsule are important characteristics of the two-piece capsule dosage form as they determine the release and exposure of the drug formulation into the dissolution media. One of the basic differences between gelatin and HPMC capsules is a temperature-dependent dissolution profile of the gelatin capsule. Gelatin requires a temperature of 30°C to exhibit rapid disintegration and dissolution behavior, while HPMC dissolves independently of the temperature (Chiwele et al. 2000). By using a newly developed “dynamic open flow through test apparatus” that mimics flow rates, intragastric temperature, and gastric motility in fasted state conditions, no difference in the in vitro dissolution is seen between gelatin and tg-HPMC capsules when increasing the temperature slowly from 24°C to 37°C, mimicking the administration of a drug product with a glass of water. However, the application of the dynamic open flow through test apparatus also provided evidence for the importance of the intraluminal pressure caused by physiological gastric motility on capsule rupture and dissolution. Applying a short intragastric motility event after 10 min triggered product release from HPMC capsules (Garbacz et al. 2014).
The disintegration test according to the Pharmacopoeia requires the capsules to disintegrate in less than 15 min. As the disintegration test is quite unspecific, different researchers have investigated rupture time using a dissolution apparatus II with an in-line UV detector as a more precise method to determine the time point when the capsule opens and releases the content. The results from these studies confirm that gelatin capsules have a rapid rupture time of as little as 2 min in gastric or simulated intestinal media. In contrast to gelatin capsules, the HPMC capsule rupturing time is dependent on the HPMC capsule composition. HPMC capsules containing carrageenan as a gelling agent show a slightly faster rupturing time of 3–4 min in the gastric fluid compared to 6–8 min for HPMC capsules manufactured by thermo-gelation (El-Malah et al. 2007; Ku et al. 2011). However, in the study published by El-Malah et al. (2007), longer disintegration times were seen in simulated gastric fluids for the carrageenan containing HPMC capsules (6–10 min), which was not the case in the study performed by Ku et al. The longer rupturing time of HPMC capsules compared to the gelatin capsules is based on the different base polymer properties. HPMC requires more time to hydrate and dissolve, which is caused by the 3–5 min rupturing lag time. The lag time in the rupturing of HPMC capsules compared to gelatin capsules is also seen in the dissolution test (Figure 5.3).

Figure 5.3Dissolution profile of a fast-dissolving caffeine blend in gelatin (HGC) and HPMC (Vcaps® Plus) capsules at pH 1.2. (Courtesy of Capsugel.)
The dissolution of different HPMC capsules has been found to differ significantly from type to type, depending on the manufacturing process, the type of gelling system in the formula, and the pH and the ionic strength of the media. Figure 5.4a shows the dissolution profile of a carrageenan gelling system containing c-HPMC capsule in different media. In a pH 6.8 USP phosphate buffer, the capsule shows a slow dissolution and simulated milk fluid, and the capsule rupture and release were delayed to about 30 min. These results are in accordance with the dissolution results of a previous study where the USP 7.2 phosphate buffer was considered as unsuitable for c-HPMC capsules because of its slow release and was replaced by a pH 7.0 tribasic sodium phosphate buffer at 150 rpm in a USP basket dissolution test system (Honkanen et al. 2001). The delayed dissolution could also be observed in pH 1.2 media containing a high potassium concentration. The delayed dissolution profiles are believed to be caused by the interaction of the potassium cations and the carrageenan gelling polysaccharide and the pH dependence of the gel formation (Doyle et al. 2002). In Figure 5.4b, the dissolution profile of the pure HPMC capsule manufactured by thermo-gelation is shown. The rupture and dissolution time of these capsules are independent from the pH and cationic strength of the media and release occurs after 6–10 min consistently.


Figure 5.4(See color insert.) Dissolution of a fast-dissolving caffeine formulation in different types of HPMC capsules. (a) HPMC capsule containing carrageenan as a gelling system. (b) HPMC capsule manufactured by thermo-gelation without any gelling system (Vcaps® Plus). (Courtesy of Capsugel.)
The dissolution profile of the c-HPMC capsules in a high-pH medium also shows a high variability between capsule types (Figure 5.5). These results are comparable to the results from the study by Ku et al. (2011) for a poorly soluble free acid compound that is ionized beyond the pKa of 4.7 when using the USP pH 6.8 phosphate buffer with the addition of a surfactant (hexadecyl trimethyl ammonium bromide).

Figure 5.5Individual dissolution profiles (V1–V6) of a caffeine formulation in a carrageenan HPMC capsules in pH 6.8 USP phosphate buffer. (Courtesy of Capsugel.)
Similar in vitro dissolution results have been reported for HPMC capsules containing gellan gum as a gelling agent (Cole et al. 2004b). In comparison to gelatin capsules, g-HPMC capsules dissolve rapidly in distilled water, but show a very slow release in pH 1.2 buffer and slightly longer dissolution time in the USP potassium phosphate buffer at pH 7.2. In a pH 7.2 TRIS buffer system, the g-HPMC capsules demonstrate the typical HPMC capsule lag time with a dissolution equivalent to the gelatin capsules starting somewhere in between 5 and 10 min (Cole et al. 2004b).
In Vivo Performance of HPMC Capsules
The first HPMC capsules introduced 20 years ago were manufactured by the traditional hard capsule process and contained either carrageenan (c-HPMC) or gellan gum (g-HPMC) as a gelling system. Since the introduction in 2007 of tg-HPMC capsules manufactured by thermo-gelation, the use of HPMC capsules in pharmaceutical development has gained substantial interest because of its demonstrated in vivo performance.
Investigations centered on the esophageal transit time of HPMC capsules have been performed with HPMC capsules containing carrageenan and gellan gum. A study by Honkanen et al. investigated the detachment forces of c-HPMC capsules and gelatin capsules in vitro using esophageal tissue derived from slaughterhouses (Honkanen et al. 2002). The results suggest that the detachment of gelatin capsules adhered to the isolated mucosa requires 2.5 times higher forces compared to the HPMC capsules. When the same group investigated the esophageal transit of a size 0 c-HPMC capsule in six healthy volunteers after two administrations each, the results showed that for four of the capsule administrations, there were esophageal residence times ranging from 22 to 143 min (Honkanen et al. 2004). However, these results have not been seen in other studies using gamma scintigraphy with either size 0 c-HPMC (Tuleu et al. 2007) or size 1 g-HPMC (Cole et al. 2004b). These studies found esophageal transit times for both types of HPMC capsules in the range of 10–20 s, which is also in agreement with the reported esophageal transit times of gelatin capsules. It should be noted that the esophageal transit of any solid dosage form depends on the size and shape of the dosage form, the position of the volunteer, and the amount of water provided upon administration (Stegemann et al. 2012). Honkanen suggested that the reason for the observation in their study might the incorrect water intake upon capsule administration by the four volunteers on the first day (Honkanen et al. 2004). Moreover, the protocol required capsule intake in a sitting position and then laying down in a supine position 30 s after administration, which could also have contributed to the long capsule residence times on four occasions.
The first in vivo studies performed with HPMC capsules were published in 2001 using c-HPMC capsules. Gelatin capsules and c-HPMC were filled with a 200-mg ibuprofen-containing lactose blend in size 0 capsules and compared in a single-dose, crossover bioequivalence test (Honkanen et al. 2001). The major pharmacokinetic parameters, AUC and Cmax, were comparable for gelatin and c-HPMC capsules (AUC, 109 vs. 111 mg h/L; Cmax, 39.7 vs. 38.6 mg/L) and tmax slightly increased from 1.19 h to 1.5 h, which is not statistically significant. Similar pharmacokinetic results have been observed for g-HPMC capsules when compared with gelatin capsules filled with 200 mg of ibuprofen formulated with a blend of microcrystalline cellulose and Mg-stearate in size 1 capsules administered in fasted and fed conditions (Cole et al. 2004b).
To understand the in vivo disintegration of HPMC capsules, gamma scintigraphy studies have been performed with c-HPMC and g-HPMC capsules. The initial disintegration time of c-HPMC capsules in a fasted state was investigated with powder fills of two different HPMC grades and found to be similar with 49 and 53 min (Honkanen et al. 2004). These times are longer than the times found for g-HPMC capsules with an initial disintegration time of 28 min (Cole et al. 2004b). As expected, the initial disintegration was increased in the fed state (standardized high-fat meal, 1300 kcal) for gelatin, as well as g-HPMC capsules to 23.4 min and 1, respectively. In contrast to these findings, recent studies suggest very short initial disintegration times for gelatin and c-HPMC capsules administered in fasted and fed conditions. In the fasted state, the initial disintegration time for gelatin and c-HPMC capsules was reported to be 7 min compared to 9 min in the fasted state (Tuleu et al. 2007) and 12 min compared to 16 min in the fed state. However, in these studies, the fed state was a breakfast of not more than 500 kcal and no information was provided about the time elapsed between breakfast and when the capsule was administered (Jones et al. 2012).
A comparative in vivo performance investigation between tg-HPMC capsules and gelatin capsules using a fast-releasing tablet (Excedrin Extra Strength caplets) with three different BDDCS class 1 drugs (acetylsalicylic acid, acetaminophen, and caffeine) as a marker has recently been reported. The study was a randomized, crossover study in 24 healthy and fasted subjects receiving either two Excedrin Extra Strength caplets overencapsulated in gelatin or tg-HPMC capsules. Despite the in vitro dissolution lag-time of 5–10 min between the gelatin and tg-HPMC capsules, the statistical analysis confirmed that the 90% confidence intervals for Cmax, AUC0-t, and AUC0-∞ are well within the range of 80–125.00% acetaminophen, aspirin (acetylsalicylic acid, total salicylates), and caffeine for comparison of the gelatin overencapsulated caplet and the HPMC capsule overencapsulated caplet (Stegemann et al. 2015).
Clinical studies have investigated tg-HPMC capsules for dabrafenib, a BCS class 2 compound and a weak base with pH-dependent dissolution characteristics, for its in vitro and in vivo performance in comparison to gelatin capsules. The in vitro dissolution of dabrafenib in FaSSGF at pH 1.6 measure over 24 h shows a three- to fourfold increase in the peak dissolution for the tg-HPMC capsule compared to gelatin and even though precipitation occurred after 2 to 3 h, the steady state above the drug dissolved with gelatin capsules. This higher solubility related to the precipitation inhibition effect of the tg-HPMC was reproduced in vivo under fasted conditions, which led to a 1.8- to 2.0-fold increase in the bioavailability of dabrafenib after a single dose in HPMC capsules compared to gelatin capsules (Ouellet et al. 2013).
A 180-subject crossover bioequivalence study carried out in 2013 compared the administration of a commercial 150-mg immediate-release formulation of dabigatran filled in either c-HPMC capsules containing a gelling system or tg-HPMC capsules manufactured by thermo-gelation (National Institutes of Health 2013). The dabigatran formulation filled in tg-HPMC capsule reached 125% of bioavailability of the c-HPMC capsules and reduced the geometric coefficient of variation from an average of 50% to 32%. As the main difference between the two HPMC capsules was the presence or absence of a gelling system (carrageenan), the results reflect the important in vitro findings of the pH- and ionic strength-dependent dissolution of the gelling system capsules in vivo.
Special Applications of HPMC Capsules
Coating of HPMC Capsules
To achieve targeted intestinal drug delivery, capsule coating is being considered as a suitable option. Aqueous-based coating systems on HPMC capsules have been shown to achieve high performing functional coats because of their adhesion properties and resistance with regard to capsule softening or brittleness during processing. A recent study using scintigraphy to investigate coating performance described the coating of HPMC capsules based on two different types of coatings (Eudragit L 30 D-55 or Eudragit FS 30D at coating thicknesses of 6, 8, 10, and 12 mg/cm2) (Cole et al. 2002).
In vitro dissolution test results confirm that the capsule coated with 6 and 8 mg/cm2 Eudragit L 30 D-55 and 6, 8, and 10 mg/cm2 Eudragit FS 30 D did not release the drug acetaminophen over 2 h at pH 1.2, but released rapidly at pH 6.8. The intestinal targeting of the coatings was demonstrated in a gamma-scintigraphy study in human volunteers whereby Eudragit L 30 D-55-coated capsules disintegrated completely in the small bowel and Eudragit FS 30 D-coated HPMC capsules disintegrated between the mid distal small intestine and the proximal colon while no release was seen in the stomach for either coating. This study establishes the in vitro–in vivo correlation and hence possibility for intestinal targeting through coated HPMC capsules (Cole et al. 2002).
HPMC Capsules for Dry Powder Inhalation
Capsule-based dry powder inhalation (DPI) systems were introduced for the treatment of respiratory diseases approximately 45 years ago. The main elements of these drug delivery systems are the fine drug particles or drug particle–carrier interactive mixtures that are filled as a pre-metered dose into a capsule. For administration, the capsules containing the low therapeutic dose are administered directly to the respiratory system via an inhalation device. Because of the simplicity and cost-effectiveness of these platforms, capsule-based DPI systems remain the pulmonary delivery method of choice for new drugs and are gaining increasing popularity for the delivery of generic versions and new products launched in emerging markets (Stegemann et al. 2013).
In pulmonary DPI systems, the two-piece capsule plays an equally important role in product stability and performance as the drug or drug carrier interactive powder mixture and the device component. In general, the drug formulation is developed in a defined DPI device using a prequalified inhalation grade gelatin or HPMC capsule, which is then further optimized to achieve the desired fine particle drug release profile or product stability. With the introduction of HPMC capsules for DPI, several technological advantages that substantially extended the design space of capsule-based DPI systems were achieved.
Since fine drug particles of less than 5 μm size are normally very cohesive, interactive powder mixtures of the drug particles on coarse carriers of 30–100 μm size are used, whereby the fine drug particles are attached to the surface of the carrier. Upon activation, the fine drug particles have to detach from the coarse particles to be delivered to the deepest parts of the lungs. To develop capsules suitable for this application, the major quality attributes of the capsules to consider are moisture content, powder retention, and opening performance under shear force, piercing, or cutting.
The ability to adjustable moisture content of HPMC capsules at lower humidity levels, while maintaining shell flexibility upon mechanical impact, is one of the main features of HPMC capsules for DPI applications. The lower humidity levels prevent moisture affecting the formulations’ stability and dispersibility over the capsule’s shelf life. To minimize powder retention of the adhesive fine drug particles, the electrostatic charges can be reduced to a certain moisture level with the HPMC capsule. For each drug formulation and device combination, the optimal moisture range of the HPMC capsules can be determined and adjusted on a commercial scale. Further reduction of powder retention can be achieved by modifying manufacturing process parameters such as lubrication to achieve powder retention levels below 0.1% (Stegemann et al. 2014b).
Conclusion
The first HPMC capsules were introduced into the pharmaceutical market 20 years ago; since then, HPMC capsule technology has been applied to both pharmaceutical development and marketed products. The first types of HPMC capsules were manufactured using the traditional capsule manufacturing process, requiring the use of a gelling system within the HPMC shell formulation. The major limitation of gelling systems containing HPMC capsules is the capsule dissolution characteristic that is affected by pH, cations, and the ionic strength of the dissolution media and gastric and intestinal juice in vivo. In 2007, a new HPMC capsule that was manufactured with a modified hard capsule manufacturing technology became commercially available, which allowed the production of HPMC capsules by thermo-gelation. These HPMC capsules are manufactured from a pure aqueous HPMC solution without the addition of other components or gelling systems. HPMC capsules manufactured by thermo-gelation dissolve in all media according to the solubility characteristics of HPMC and dissolve consistently across all dissolution media. Compared to gelatin capsules, the initial rupture time of HPMC capsules has a lag time of approximately 5 min, normally releasing the content after 5–10 min, whereas release from gelatin capsules occurs after 2–3 min.
In vivo studies have confirmed rapid capsule dissolution of HPMC capsules in the gastrointestinal (GI) tract measured by gamma scintigraphy and bioavailability. There is some evidence that HPMC capsules manufactured by thermo-gelatin without the use of gelling systems can inhibit drug precipitation in the GI tract, increasing bioavailability as well as variability for certain drugs (National Institutes of Health 2013; Ouellet et al. 2013).
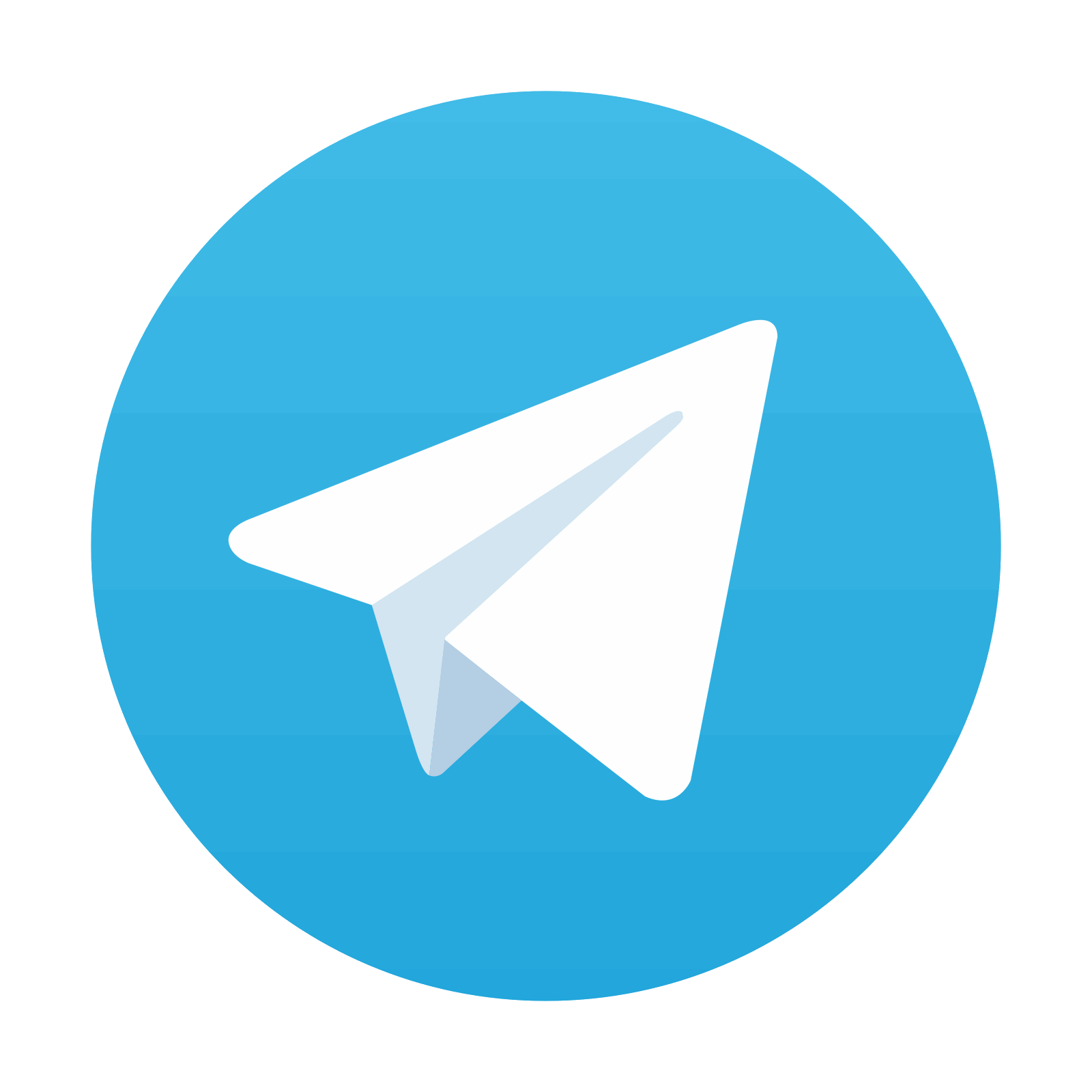
Stay updated, free articles. Join our Telegram channel

Full access? Get Clinical Tree
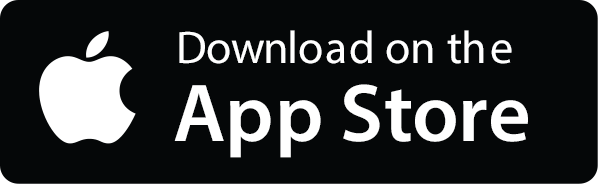
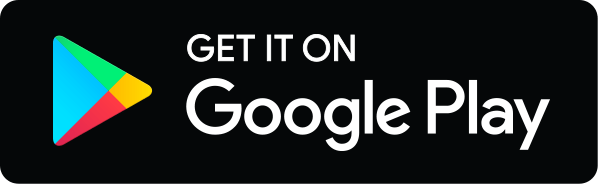