1 Introduction
The group of agents which comprises antiseptics, disinfectants, chemical sterilants and preservatives (often collectively called biocides) have frequently been classified as non-specific protoplasmic poisons. Such a broad generalization is, however, far from the true position.
It is often convenient to consider the modes of action of biocides in terms of their targets within the bacterial cell, in particular the region of the cell in which their activity is deemed to predominate. Thus agents have been described variously as cell wall, membrane and cytoplasm-active. This characterization, whilst having the benefits of simplicity, does not necessarily describe their mechanism of action; this is best classified by effects on functional structures and cellular processes. The range and complexity of the reactions involved will become apparent from this account and Table 20.1, and it is worth emphasizing here that many of these substances exhibit concentrationdependant dual or even multiple effects. More detailed treatments of the subject will be found in the references at the end of this chapter.
Table 20.1 Cellular targets for non-antibiotic antibacterial agents
Crosses, indicating activity, which appear in several rows for a given compound, demonstrate the multiple actions for the compound concerned. This activity is nearly always concentration-dependent, and the number of crosses indicates the order of concentration at which the effect is elicited, i.e. +, elicited at low concentrations, + + +, elicited at high concentrations;
QACs, quaternary ammonium compounds; PHMB, polyhexamethylene biguanides; TCS, tetrachlorosalicylanilide; TCC, trichlorocarbanilide.
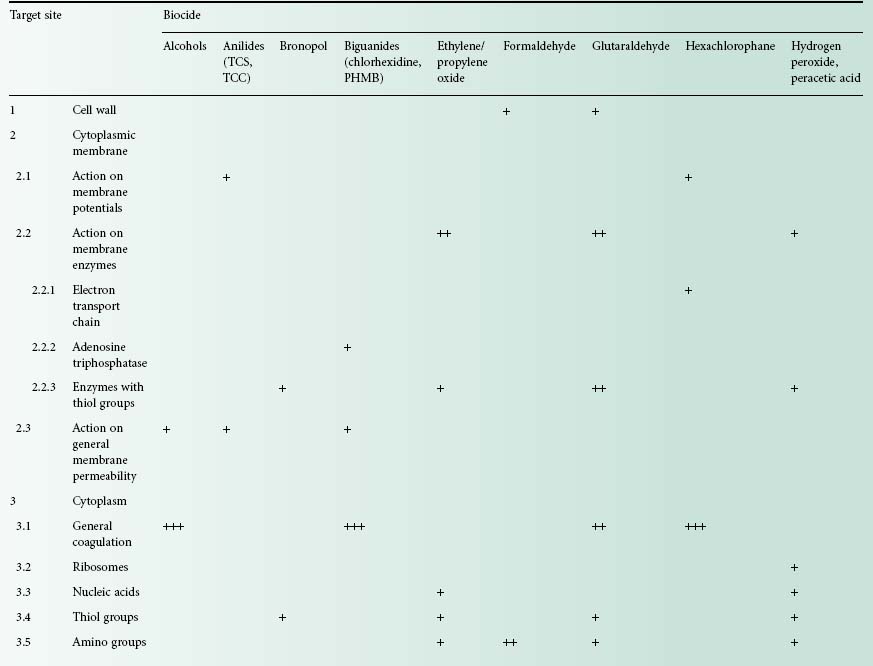
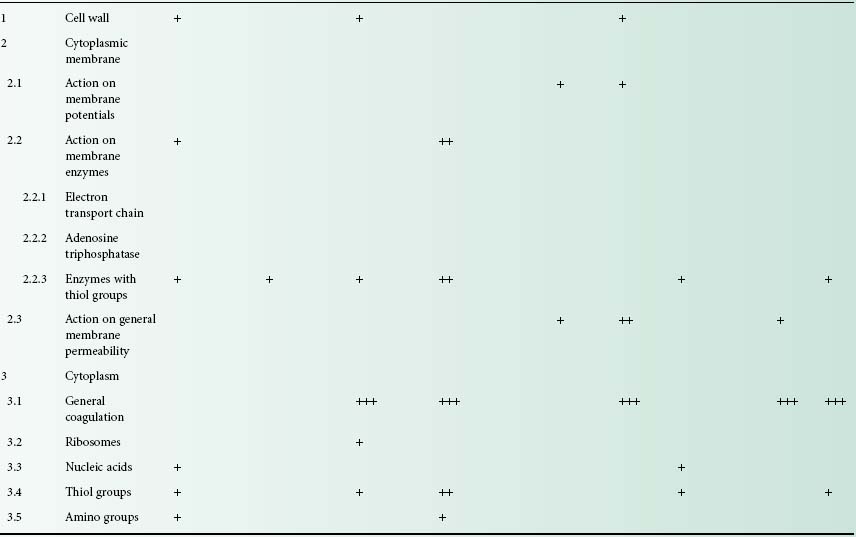
For a chemical to exhibit antimicrobial activity it usually has to undergo a sequence of events that begins with adsorption on to the microbial cell surface. This initial uptake is a physicochemical phenomenon which can be generally characterized into one of several uptake isotherms (Figure 20.1); it bears a relationship to the concentration exponent (Chapter 19) which describes the influence of concentration on activity (Table 20.2). In the many cases where the chemical has an intracellular site of action, adsorption must be followed by passage through porin channels in Gram-negative cells (Chapter 3), diffusion across, or into, the lipid-rich cytoplasmic membrane, and finally, interaction with proteins, enzymes, nucleic acids or other targets within the cyto-plasm. These processes are markedly influenced by the physicochemical characteristics of the biocide, e.g. ioni-zation constant and lipid solubility, so the wide diversity of structures exhibited by biocide molecules (Chapter 19) complicates the prediction of antimicrobial potency and explanation of their mechanisms of action. Despite this, it is important to recognize that there is a basis upon which the mode of action might be deduced, because there are certain molecular features of biocides that are associated with activity against particular cellular targets.
Figure. 20.1 Typical uptake isotherms associated with the initial biocide-bacterium interaction. (a) S-shape, cooperative sorption occurs as applied biocide concentration increases; (b) L-shape, Langmuir uptake with biocide molecules orientated at a fixed number of binding sites; (c) H-shape, special form of the L-shape isotherm indicative of high affinity uptake; (d) C-shape,constant partition of biocide from solution until bacterial surface is fully saturated; (e) Z-shape, enhanced uptake following breakdown in cell structure at a critical applied biocide concentration.
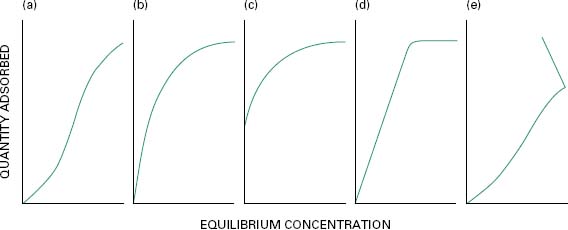
Table 20.2 Relationship between biocide concentration exponent, uptake isotherm and mechanism of interaction
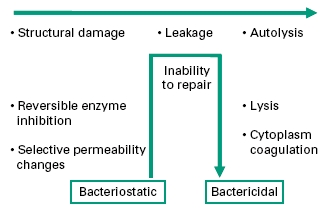
Antimicrobial activity is often strongly influenced by the affinity of the biocide for structural or molecular components of the cell, and this, in turn, may depend upon the attraction of dissimilar charges or on hydrophobic interactions. Antimicrobial drugs whose active species is positively charged, e.g. quaternary ammonium compounds (QACs) and chlorohexidine, display an affinity for the negative charges of sugar residues on the microbial cell surface or phosphate groups on the membrane(s); adsorption of these biocides, and thus their antimicrobial activity, is increased as the pH rises and the cell surface becomes more electronegative. Antimicrobial chemicals possessing a long alkyl chain, on the other hand, may integrate into the hydrophobic region of phospholipid molecules within the membrane and so cause membrane disruption and fatal permeability changes. Further examples of structure-activity relationships are afforded by aldehydes, particularly glutaraldehyde, which is an elec-trophile that is able to react with molecules possess-ing thiol (SH) or amino groups, e.g. proteins. This reaction, too, increases with pH, so aldehydes are more active in alkaline conditions. Biocides containing heavy metal ions, e.g. silver or mercury, also damage or inacti-vate enzymes and structural proteins by virtue of interactions with thiol groups. A number of phenols and bisphenols incorporate a hydroxyl group that is capable of generating a labile proton, i.e. they are weak acids. A weakly acidic nature combined with significant lipid solubility are properties associated with uncoupling agents, i.e. those molecules that can disrupt the proton-motive force that is responsible for oxidative phosphorylation in the cell. It is thought that these molecules dissolve in the lipid bilayer of the membrane and act as proton conductors by virtue of their ionizability (section 4.4.2). This property, possessed by biocides such as phenoxythanol and fentichlor, results in the failure of many important energy-requiring processes in the cell, including the concentration and retention of sugars and amino acids.
In any consideration of mechanism of action, due regard should be given to the initial health of the organism, duration of contact with the biocide, and the concentration of biocide employed. Antibacterial effects may progress from early, sublethal events to multiple lesions of bactericidal consequence. Figure 20.2 identifies events in order of severity, but should not be interpreted as defining the normal progression of cell injury. As disclosed in the following sections, the biocide interaction may induce particular lesions over others; this will most certainly be in a concentration-dependant manner
4.1 Oxidation reactions
Biocides with oxidizing (electron-withdrawing) ability are widely used as disinfectants and chemical sterilants, and include the halogens (chlorine, hypochlorites,bromine, iodophors) and peroxygens (hydrogen peroxide, peracetic acid and chlorine dioxide). They can exert specific effects on essential microbial macromolecules causing, variously: strand breakage and adduct formation on DNA and RNA with disruption of replication, tran-scription and and translocation processes; degradation of, particularly, unsaturated fatty acids leading to loss of membrane fluidity and subsequent reduced functionality of membrane-bound proteins; and specific modifications to amino acid residues, most notably disulphide bonds, leading to changes in protein primary structure and con-formation with consequent disruption of structural enzymic functions. An accumulation of these effects can be particularly devastating to the microbial cell.
4.2 Cross-linking reactions
The aldehydes formaldehyde, glutaraldehyde and orthophthalaldehyde, and the sterilant alkylating agents ethylene oxide and propylene oxide, are both highly reactive chemical classes. The alkylating agents exhibit particularly strong reactions with guanine residues causing cross-linking between DNA strands, inhibiting DNA unwinding and RNA translation. The amino, carboxyl, sulphydryl and hydroxyl groups of structural or enzymic proteins are also susceptible to alkylation, causing cross-links between adjacent amino acid chains and also with other amino acid-containing structures such as peptidoglycan. The aldehydes are generally more specific with greatest effect against the amino groups of surfaceexposed lysine or hydroxylysine residues of proteins, again causing extensive cross-linking.
In all instances, progressive cross-linking leads to macromolecule malfunction causing inhibition or arrest of essential cell functions. It is safe to say that there is no single fatal reaction but that death results from the accumulated effect of many reactions in a manner similar to oxidizing agents (section 4.1).
4.3 Coagulation
The cross-linking reactions identified in section 4.2 give rise to macromolecule denaturation which can be recognized under electron microscopy as intracellular coagulation. Coagulative effects are not unique to aldehydes and alkylating agents, however, and high concentrations of disinfectants such as chlorhexidine, phenol, ethanol and mercuric salts will also coagulate the cytoplasm. This most likely arises from the precipitation of protein caused by a variety of interactions including ionic and hydrophobic bonding and the disruption of hydrogen bonds.
4.4 Disruption of functional structures
The integrity and functions of the bacterial cell are dependant upon critical macromolecular structural arrangements including within the cell wall and cytoplasmic membrane (Chapter 3). A number of biocides can have a profound effect on these organelles.
4.4.1 Cell wall
This structure is the traditional target for a group of antibiotics which includes the penicillins (Chapter 11), but a little-noticed report which appeared in 1948 showed that low concentrations of disinfectant substances caused cell wall lysis such that a normally turbid suspension of bacteria became clear. It is thought that these low concentrations of disinfectant cause enzymes whose normal role is to synthesize the cell wall to reverse their role in some way and effect its disruption or lysis. In the original report, these low concentrations of disinfectants (formalin, 0.12%; phenol, 0.32%; mercuric chloride, 0.0008%; sodium hypochlorite, 0.005% and merthiolate, 0.0004%) caused lysis of Escherichia coli, streptococci, and staphylococci.
Divalent cations, in addition to their role as enzyme cofactors, also stabilize cell wall, membrane and ribosomal structures. In particular, magnesium serves to link the lipopolysaccharide (LPS) of Gramnegative bacteria to the outer membrane. Chelators, particularly ethylenediamine tetraacetic acid (EDTA), have been used to disrupt this link and cause the release of LPS into the medium. The loss of outer membrane integrity and subsequent permeabilization has been exploited in the potentiation of biocides, including combinations of EDTA with chloroxylenol, cetrimide, phenylethanol and the parahydroxy benzoic acid esters (Chapter 19).
4.4.2 Cytoplasmic membrane
The bacterial cytoplasmic membrane consists of an impermeable, negatively-charged, fluid phospholipid bilayer incorporating an organized array of membrane associated proteins. Through the membrane-bound electron transport chain aerobically, or the membrane-bound adenosine triphosphatase (ATPase) anaerobically, the bacterium succeeds in maintaining a transmembrane gradient of electrical potential and pH such that the interior of the cell is negative and alkaline. This proton motive force, as it is called, drives a number of energy-requiring functions which include the synthesis of ATP, the coupling of oxidative processes to phosphorylation, a metabolic sequence called oxidative phosphorylation, and the transport and concentration in the cell of metabolites such as sugars and amino acids. This, put briefly, is the basis of the chemiosmotic theory linking metabolism to energy-requiring processes.
Certain chemical substances have been known for many years to uncouple oxidation from phosphorylation and to inhibit active transport, and for this reason they are named uncoupling agents. They are believed to act by partitioning into the membrane and rendering it permeable to protons, hence short-circuiting the potential gradient or protonmotive force. Some examples of antibacterial agents which owe at least a part of their activity to this ability are tetrachlorosalicylanilide (TCS), tricarbanilide, trichlorocarbanilide (TCC), pentachlorophenol, di-(5-chloro-2-hydroxyphenyl) sulphide (fentichlor),2-phenoxyethanol, and lipophilic acids and esters.
The membrane, as well as providing a dynamic link between metabolim and transport, serves to maintain the pool of metabolites within the cytoplasm. A general increase in membrane permeability brought about by the association and likely insertion of biocide molecules into the lipid bilayer was recognized early as being one effect of many disinfectant substances.
Treatment of bacterial cells with appropriate concentrations of such substances as cetrimide and other QACs, chlorhexidine, polyhexamethylene biguanides, phenol and hexylresorcinol causes a leakage of a group of characteristic chemical species. The potassium ion, being a small entity, is the first substance to appear when the cytoplasmic membrane is damaged. Amino acids, purines, pyrimidines and pentoses are examples of other substances which will leak from treated cells. If the action of the drug is not prolonged or exerted only in low concentration, the damage may be reversible and leakage may only induce bacteriostasis. There is however, evidence that a depletion of intracellular potassium caused by membrane damage can lead to the activation of latent ribonucleases and the consequent breakdown of RNA. Several biocides, including cetrimide and some phenols, are known to cause the release of nucleotides and nucleosides following an autolytic process. This is irreversible and has been proposed as an autocidal (suicide) process, committing the injured cell to death (Denyer & Stewart, 1998).
Surface-associated proteins within the membrane fulfil a number of important roles including wall biosynthesis, nutrient transport and respiration. Usually enzymes, these macromolecules are often topologically organized and uniquely exposed to disruption by biocidal agents. Thus, hexachlorophane inhibits the electron transport chain in bacteria, chlorhexidine has been shown to inhibit ATPase, and thiol-containing membrane dehydrogenases are highly susceptible to mercury-containing antibacterials, silver,2-bromo-2-nitropropan-1,3-diol (bronopol) and isothiazolinones.
Mention has already been made of the use of permeabilizing chelators to enhance the penetration of biocides to their target (section 4.4.1). Much effort has also been expended in the search for synergistic combinations of biocides which, when added together, will greatly amplify the bactericidal effect. While theoretically possible, and potentially predictable from mechanism of action studies, in practice this effect is elusive; combinations of phenylmercuric acetate with benzalkonium chloride, lipophilic weak acids with fatty alcohols, and chlorocresol with phenylethanol have been reported. The most likely route to enhancing activity lies with optimizing biocide formulations to ensure maximum availability of the active moiety—particularly important in situations of pHdependency or poor water solubility. In the area of pharmaceutical and cosmetic preservation, constructive use of formulation ingredients, each with some intrinsic antimicrobial activity, has successfully built on the activity of the original preservative agent to create a cumulative bactericidal effect, an approach called ‘hurdle technology’.
6 Mechanisms of resistance to biocides
Bacterial resistance to biocides has been reported since the 1950s, notably with QACs, biguanides and phenolics. Overall there has been more documented evidence of bacterial resistance to antiseptics than to disinfectants (Table 20.3). It is worth mentioning that some bacteria surviving in biocidal formulations have been associated with outbreaks and pseudo-outbreaks of infection (Weber et al., 2007). Bacterial resistance to all known preservatives has also been reported (Chapman et al., 1998). Recently, much interest has focused on bacteria surviving highlevel disinfection, which is usually employed for the disinfection of medical devices. Thus bacteria surviving exposure to the in-use (high) concentration of highly reactive biocides (e.g. glutaraldehyde, chlorine dioxide, hydrogen peroxide) have been isolated and studied. In 2009, a large outbreak of atypical mycobacteria in at least 38 hospitals in Brazil was reported. These mycobacteria were traced to endoscope contamination and were resistant to 2% w/v glutaraldehyde but also to the clinical concentration of front-line antibiotics against mycobacteria (Duarte et al., 2009). This was the first time that biocide resistance was linked to antibiotic resistance, nosocomial infection and a large infection outbreak.
Table 20.3 Examples of bacterial resistance in biocidal products
Adapted from Weber et al. (2007).
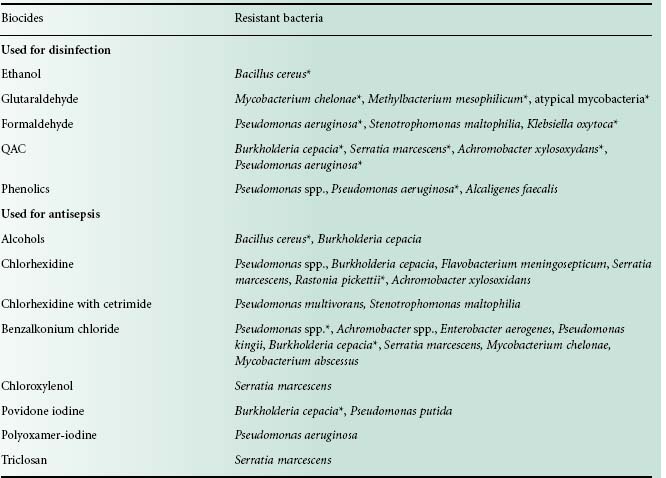
*Bacteria associated with pseudo-outbreak or outbreak.
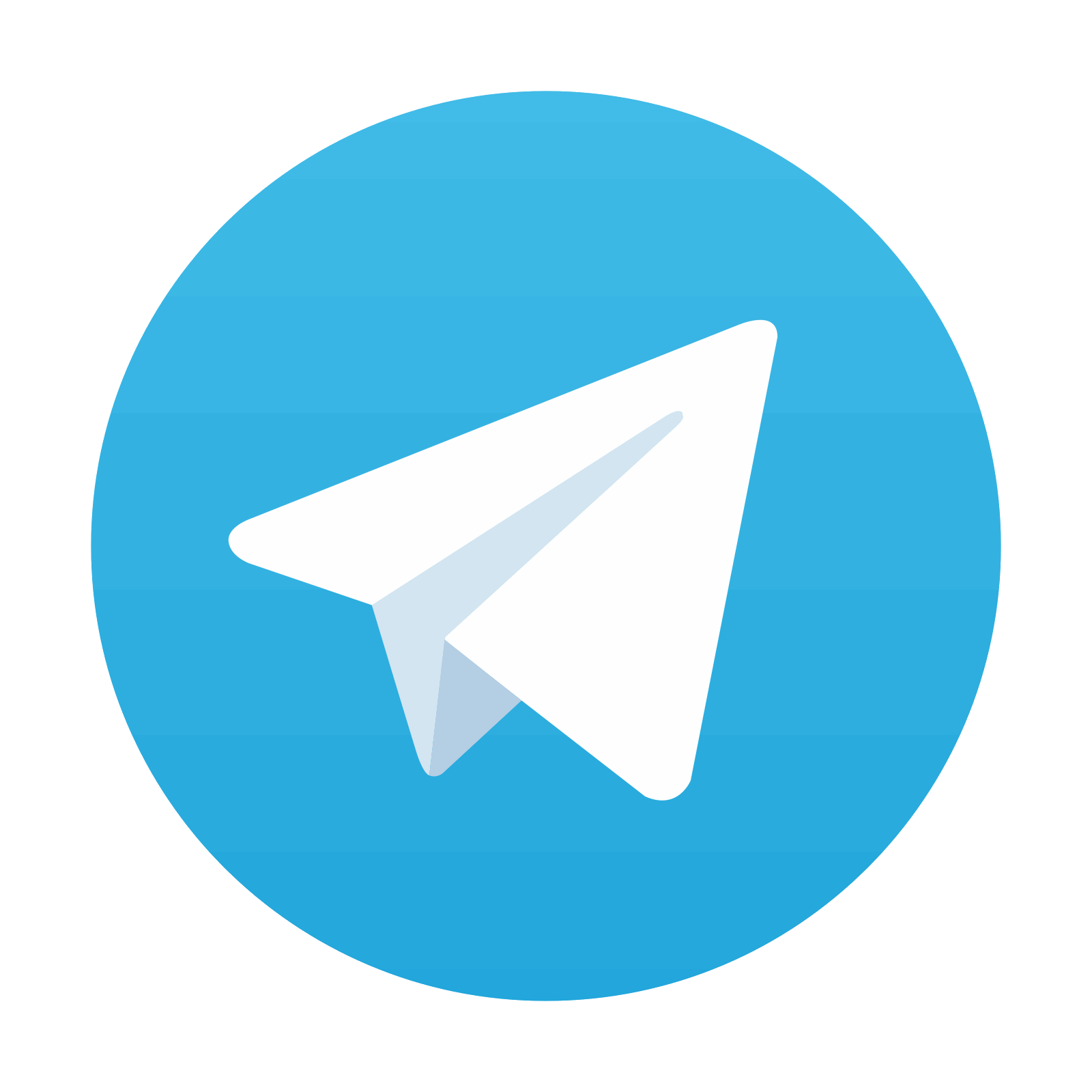