Application
Considerations
Genetics
Ethical issues (presymptomatic and prenatal testing)
Consequences for family members
Informed consent requirements
Requirements for family-related information (ethnicity, pedigree, specimens)
Time sensitivity (prenatal diagnosis)
Complex risk-assessment calculations
Oncology
Many types of samples
Extensive use of paraffin-embedded tissue and fine needle aspirate samples (familiarity with limitations)
Small biopsy specimens
Need for diagnostic samples for optimal interpretation of minimal residual disease test results
Infectious diseases
High-volume testing
Use of automated platforms
High cost of commercial in vitro diagnostic test kits
Increased need for quantitative testing with a wide dynamic range and low detection limit
Identity testing
Chain-of-custody documentation for specimens
Special patient identification requirements
Complex calculations
Special accreditation requirements for paternity and forensic testing
Special qualifications required for laboratory director
HLA testing
Time sensitivity (<24 h turnaround time)
Complex analyses
Special accreditation requirements for the laboratory and laboratory director
Genetic Disorders
All diseases have a genetic contribution, whether it is a specific genetic disease or an increased likelihood for developing a medical condition. Genetic disorders are primarily caused by mutations present in every cell of an individual. Molecular testing for neoplasia or an infectious disease requires a sample of the tumor or the infected tissue, respectively, while molecular testing for a genetic disease can be performed on most accessible tissue types, the simplest being a blood or buccal cell specimen. In addition, because genetic testing examines germline mutations, it has important implications not only for the individual but also for family members that may have inherited the same mutation. Genetic testing also raises ethical concerns, including informed consent, potential for employment or insurance discrimination based on test results, and beginning-of-life issues with prenatal or preimplantation testing. Genetic test results often are not definitive, requiring complex risk-assessment calculations for interpretation. The American College of Medical Genetics (ACMG) and the American Society of Human Genetics (ASHG) have published guidelines and recommendations for many of the more common single-gene disorders (Table 62.2).
Table 62.2
Professional organizations providing standards and guidelines for molecular pathology laboratories
Organization | Standards and guidelines |
---|---|
Centers for Medicare and Medicaid Services (CMS; http://www.hcfa.gov) | Mandatory federal guidelines for regulating laboratory testing via the Clinical Laboratory Improvement Amendments of 1988 (CLIA) and the Laboratory Improvement Amendments of 2003 (CLIA) “Final Rules” |
New York State Department of Health (NYS DOH) | Comprehensive test approval policy and submission guidelines |
The Clinical Laboratory Evaluation Program (CLEP) | Assay approval in genetic testing—molecular |
Assay approval in oncology | |
Approval of microbiology nucleic acid amplification assays | |
Next-generation sequencing (NGS) guidelines for somatic genetic variant detection | |
American College of Medical Genetics and Genomics (ACMG; http://www.acmg.net) | Standards and guidelines for clinical genetic laboratories |
Gene patents and accessibility of genetic testing | |
Policy regarding genetic testing of minors | |
Storage and use of genetic materials | |
Recommended standards for interpretation of sequence variations | |
Recommendations and guidelines regarding genetic testing for certain diseases including fragile X syndrome, apolipoprotein testing for Alzheimer disease, Prader Willi–Angelman syndrome, Canavan disease, cystic fibrosis, Huntington disease, venous thromboembolism (factor V Leiden and prothrombin), congenital hearing loss, colon cancer, and breast and ovarian cancer | |
American Society of Human Genetics (ASHG; http://www.ashg.org) | Educational resources in human genetics |
Legislation for genetic information nondiscrimination | |
American Society for Histocompatibility and Immunogenetics (ASHI; http://www.ashi-hla.org) | Standards for molecular histocompatibility and immunogenetic testing |
Association for Molecular Pathology (AMP; http://www.amp.org/) | Educational resources in molecular pathology |
Reports and guidelines for clinical testing and diagnostic genome sequencing | |
Clinical and Laboratory Standards Institute (http://www.clsi.org) (formerly National Committee for Clinical laboratory Standards, NCCLS) | Molecular diagnostic methods for genetic diseases (MM1-A2); approved guidelines 2nd edition (2006) |
Immunoglobulin and T-cell receptor gene rearrangement assays (MM2-A); approved guideline (2002) | |
Molecular diagnostic methods for infectious diseases (MM3-A2); approved guideline 2nd edition (2006) | |
Nucleic acid-amplification assays for hematopathology (MM5-A); approved guideline (2003) | |
Quantitative molecular diagnostics for infectious diseases (MM6-A) | |
Nucleic acid sequencing methods in diagnostic laboratory medicine; approved guideline (2004) | |
Fluorescence in situ hybridization (FISH) methods for medical genetics; approved guideline (2004) | |
Proficiency testing (external quality assessment) for molecular methods; approved guideline (2005) | |
Collection, transport, preparation, and storage of specimens for molecular methods, approved guideline (2005) | |
Use of external DNA controls in gene expression assays; approved guideline (2006) | |
Diagnostic nucleic acid microarrays (2006) | |
Verification and validation of multiplex nucleic acid assays; proposed guideline (2007) | |
Interpretive criteria for identification of bacteria and fungi by DNA target sequencing (2008) | |
Quality management for molecular genetic testing | |
Establishing molecular testing in clinical laboratory environment (2011) | |
The College of American Pathologists (CAP; http://www.cap.org) | Recommendations for in-house development and performance of molecular tests (2012) |
Molecular pathology checklist for laboratory accreditation | |
Molecular Testing Guideline for Selection of Lung Cancer Patients for EGFR and ALK Tyrosine Kinase Inhibitors (jointly with the Association for the Study of Lung Cancer [IASLC], and the Association for Molecular Pathology [AMP]) (2013) | |
Molecular Markers for Colorectal Cancer (jointly with the American Society for Clinical Pathology [ASCP], and the AMP) (2015) | |
US Food and Drug Administration (FDA; http://www.fda.gov) | Guidelines for industry registration and listing of analyte-specific reagents (ASRs) |
Guidelines for laboratory development and reporting of tests using ASR | |
Premarket review templates for in-house-developed genetic tests | |
Guidelines for industry for manufacturing and validating molecular tests for the detection of HIV-1 and hepatitis C virus (HCV) | |
Draft guidance for industry, clinical laboratories, and US FDA staff for in vitro diagnostic multivariate index assays (IVDMIAs) | |
National Institutes of Health (NIH; http://www.nih.gov) | Promoting safe and effective genetic testing in the Unites States: final report of the task force in genetic testing |
Secretary’s Advisory Committee on Genetics, Health and Society (SACGHS; http://wwwAod.nih.gov/oba/sacghs.htm) (formerly Secretary’s Advisory Committee on Genetic Testing, SACGT) | Recommendations to the secretary of Health and Human Services on all aspects of the development and use of genetic tests |
Clinical Laboratory Improvement | Setting new CLIA regulations for genetic testing |
Advisory Committee (CLIAC; http://www.phppo.cdc.gov/cliac/default.asp) | Guidelines and recommendations for laboratory testing of HCV and HIV |
Centers for Disease Control and Prevention (CDC; http://www.cdc.gov) | |
Data collection of genetic testing and results |
Genetic Testing
Molecular genetic testing is currently used for the following major clinical purposes: diagnostic, carrier, prenatal/preimplantation, presymptomatic, and population screening [2]. Diagnostic testing is performed on affected individuals for establishing or confirming a clinical diagnosis. In general, for diagnostic genetic testing, the patient should have symptoms consistent with the disorder to justify performing the test. Genetic tests may be useful to diagnose an early atypical clinical presentation, or when other diagnostic procedures are more expensive, complex, or will not provide a definite diagnosis. For example, molecular testing for the absence of the survival motor neuron 1 (SMN1) gene for spinal muscular atrophy (SMA) in hypotonic newborns is a simple procedure performed on peripheral blood lymphocytes (PBL), replacing the need for a more painful and complex muscle biopsy. Carrier testing is used to detect recessive mutations in healthy individuals to identify the risk of having an affected child, and can be used for individuals with a family history of a genetic disorder, or for population-based pregnancy screening. Testing of an affected family member can identify the specific mutation present in a family, thus allowing directed testing for other family members and improving the accuracy of the risk assessment for individuals with a negative test result.
Prenatal testing refers to the detection of disease mutations in a fetus, using fetal cells obtained by amniocentesis or chorionic villus sampling (CVS). To overcome some of the problems associated with pregnancy termination for an affected fetus, some laboratories offer preimplantation genetic diagnosis (PGD) in the setting of in vitro fertilization for couples with a family history of a specific genetic disease. PGD is performed on a single blastomere from an early embryo, allowing selection of only unaffected embryos for implantation.
Presymptomatic testing is used primarily for the identification of adult-onset dominant disorders prior to the onset of clinical symptoms, in which the offspring of an affected parent has a 50 % chance of inheriting the disease. This category includes neurological diseases such as Huntington disease and some types of cancer. Presymptomatic testing is the most problematic and challenging in terms of its psychological effect on the individual and hence requires extensive protocols for pre- and post-genetic test counseling. Population screening is also a type of presymptomatic testing and focuses on the most prevalent mutations, often with different sensitivity of mutation detection for different ethnic populations.
Genetic Counseling, Informed Consent, and Ethical Considerations
Genetic testing requires special attention to informed consent issues, the appropriateness of the testing, and the urgency of testing. Many laboratories choose to require documentation of informed consent for the specific genetic test being requested prior to performing the genetic test. In some states, such as New York, documentation by the laboratory of informed consent for genetic testing is required by law (Civil Rights Law §79-l). Non-consensual genetic testing and non-consensual disclosure of genetic test results are only permitted in certain cases, such as the New York Newborn Screening Program. Informed consent can be documented by obtaining a copy of the completed informed consent form signed by the patient and the health care provider who discussed the risks and benefits of testing with the patient, or by confirmation by the physician on the laboratory requisition form that informed consent is on record in his or her medical office. Until informed consent is documented, the laboratory can extract and store the appropriate nucleic acid, but only after confirmation of informed consent can the laboratory perform testing.The clinical molecular laboratory also should review the clinical and test order information provided on the requisition form to determine whether the test is appropriate for the patient and his or her demographics and clinical history. For example, carrier testing of a minor should be deferred until the minor is an adult, and should prompt a discussion with the referring clinician. Similarly, a request for testing of an asymptomatic individual for a dominant disease requires confirmation of adequate presymptomatic genetic counseling. For prenatal test requests, gestational age should be assessed to assure that the test result is available to allow for termination of the pregnancy, if this is the family’s choice. Finally, the laboratory should understand and communicate to health care providers the sensitivities and limitations of the tests performed.
Despite the tremendous advances in understanding of the human genome, the benefit of genetic testing for the patient is not always clear. Testing may be warranted, even if the results are inconclusive or preventive strategies or treatments are not available [3], because of the usefulness of a diagnosis for the patient and their family. The availability of individual genetic information raises critical ethical, legal, and social issues because genetic testing examines the patient’s genetic makeup, rather than acquired (somatic) genome abnormalities or infectious agents, with implications for other family members. After intensive lobbying by many advocacy organizations including health professionals and industry leaders, the federal Genetic Information Nondiscrimination Act of 2008, also referred to as GINA, was passed and signed into law on May 21, 2008 [4]. This federal legislation prevents health insurers and employers from using information to determine eligibility, set premiums, or hire and fire employees, and ensures that genetic information is used for the benefit of the patient. The law also encourages individuals to take advantage of genetic screening, counseling, testing, and new therapies that will result from the scientific advances in the field of genetics. All entities that are subject to GINA must, at a minimum, comply with all applicable GINA requirements, and may also need to comply with more protective state laws.
Molecular genetic testing often requires interpretation using complex risk-assessment calculations [5]. Health care professionals must correctly interpret laboratory test results and be able to accurately convey the test results and interpretation to the patient, and the patient’s family, as appropriate. Accurate communication of results to patients and families, however, can be complex and time-consuming and therefore may be performed by genetic counselors or physicians trained in medical genetics, rather than physicians without genetics expertise. The benefits of this approach are illustrated by a study in which one-third of physicians misinterpreted a negative genetic test result for familial adenomatous polyposis (FAP) and inappropriately suggested that the patients discontinue recommended aggressive surveillance [6]. Moreover, despite tremendous advances in genetic testing technologies, understanding of genetic test results still tends to be poor in the general public and in individuals undergoing genetic testing to assess disease risk [7–9]. For these reasons, most genetic tests should be ordered through health care professionals trained in genetics to ensure that the benefits and risks of testing have been explained to the patient during the informed consent process [10, 11]. This also ensures that genetic testing is voluntary. Although obtaining consent is primarily the responsibility of the referring clinician, the clinical molecular laboratory should consider requiring documentation of informed consent [2]. New York state law allows the health care professional to attest that consent has been received and kept in the patient medical record prior to testing. Proposed changes by the Centers for Disease Control and Prevention (CDC) to CLIA regulations specific for genetic testing include a requirement for laboratories to document informed consent prior to performing a genetic test [12]. However, since the CDC issued its Notice of Intent to add informed consent documentation to CLIA more than 10 years ago, the number of clinical genetic tests has increased substantially, but the proposed changes have not been finalized.
Confidentiality
Although GINA is a strong and essential first step in the fight against genetic discrimination and misuse of medical information, the sensitivity of and need for special protections for genetic test results is unclear. Some consider that genetic test results need the highest protections of health information, even beyond that provided by the Health Insurance Portability and Accountability Act of 1996 (HIPAA) [13]. The protections include only communicating genetic test results to the referring physician or genetic counselor [2], and even potentially not placing genetic test reports into the health care record of patients to avoid discrimination. Others consider the medical significance of genetic test results warrants these results being available in the health care record and feel that “genetic exceptionalism” is wrong and may prevent appropriate medical care. HIPAA requires that release of any patient information, especially to non-health care entities, must be authorized by the patient and documented. To ensure the confidentiality of genetic test results, some patients choose to personally pay for genetic testing rather than use health insurance, and clinical molecular laboratories should develop processes for handling such payments. The US Department of Health and Human Services (DHHS) has issued guidelines for ensuring the privacy of patients’ health information as part of HIPAA [13]. Breach of patients’ confidentiality for any type of health care information, not just for genetic information, can result in litigation against the individual and/or the institution.
Cancer
Hematopoietic Neoplasms
Molecular hematopathology refers to molecular testing for leukemias, lymphomas, multiple myeloma, and myelodysplastic conditions to identify somatic DNA alterations [14]. These DNA changes are present only in the affected population of hematopoietic cells and are not a part of the genetic makeup of the individual. The recent development in polymerase chain reaction (PCR) methods, particularly quantitative real-time PCR (qPCR), has provided greater diagnostic sensitivity and specificity for diagnostic testing, as well as for monitoring of disease during and after therapy. Likewise, the ability to perform molecular tests with very small amounts of nucleic acid has enabled the use of a wide range of sample types, including formalin-fixed, paraffin-embedded (FFPE) tissue specimens. For molecular testing, formalin fixation is optimal. In contrast to genetic tests, which need be performed only once for an individual, molecular oncology tests often are performed repeatedly for initial diagnosis and during and after treatment to monitor for residual disease. If testing is not performed at the time of diagnosis, later testing of the diagnostic specimen may be useful to confirm a molecular marker for minimal residual disease (MRD) testing. Molecular hematopathology results, whenever possible, are interpreted in the context of histopathology, flow cytometry, and clinical findings.
Solid Tumors
As the molecular rearrangements and mutations that cause specific solid tumor types are identified, the detection of these mutations is used for diagnosis, prognosis, therapy selection, and MRD assessment [15]. Many of these molecular rearrangements were identified through molecular characterization of specific chromosomal translocations identified by cytogenetic analysis of solid tumors, including bone and soft tissue sarcomas such as alveolar rhabdomyosarcoma, Ewing sarcoma, and synovial sarcoma. The expression of the fusion transcripts for the more common translocations can be detected by reverse transcription-polymerase chain reaction (RT-PCR) testing. The preferred specimen for sarcoma testing is frozen tissue because the optimal analyte is RNA, but in general, the majority of molecular diagnostic tests are performed on FFPE tissue specimens with DNA as the principal analyte. Specific somatic changes in solid tumors also can provide prognostic information. For example, genetic analysis for loss of heterozygosity on chromosomes 1p and 19q in oligodendroglioma tumors correlates with a better outcome [16]. Recently the development of new drugs targeted to specific genetic changes, such as anti-tyrosine kinase inhibitors (TKIs), has enabled the development of new tests such as EGFR, KRAS, and BRAF mutation testing that predict response to the targeted therapies. The tests, coupled to certain therapies, are called companion diagnostic tests and are used for evaluating the patient’s tumor molecular profile prior to therapy initiation [17, 18]. The major laboratory issues specific to solid tumor testing today include: (1) the use of FFPE tissue specimens for mutation analysis, as formalin fixation greatly reduced the quality and integrity of DNA; (2) the careful selection of a FFPE tissue block with predominantly tumor cells or the use of macrodissection to reduce the nonmalignant cell population used for testing; and (3) working with small tissue specimens such as needle biopsies and fine needle aspiration specimens (FNAs). As next-generation sequencing (NGS) methods are increasingly used for solid tumor testing, TAT becomes an issue with oncologists needing the test results for selection and initiation of treatment, as well as the interpretation of large amount of data and variants of uncertain significance (VUS).
Infectious Diseases
As molecular techniques have become routine, more and more microorganisms are detected or characterized by molecular testing [16]. Molecular tests are especially suitable for infectious agents that are difficult to culture and for drug-resistance testing to provide more rapid diagnostic results. Molecular methods also are useful for viral quantitation as a part of monitoring response to therapy, such as human immunodeficiency virus type 1 (HIV-1), hepatitis C virus (HCV), and cytomegalovirus (CMV) infections. Because some infectious disease tests are high volume and have been clearly shown to improve patient management through therapy, automated instrumentation has been developed, and commercial test kits, including US FDA-cleared or -approved assays for many pathogen types are available. However, these commercial infectious disease test kits are expensive, and although improved patient outcomes due to early diagnosis and treatment may outweigh laboratory expenses, such savings for all infectious diseases have not been demonstrated.
Identity Testing and HLA Typing
Identity tests use polymorphic DNA markers to establish the identity of an individual or to determine an inheritance pattern [19]. Molecular identity testing is used for medical applications including analysis of bone marrow engraftment following bone marrow transplantation, and maternal cell contamination studies for prenatal genetic testing, as well as for paternity testing and forensic identity testing. Paternity and forensic identity testing, in particular, require additional considerations, including special accreditation (reviewed in Table 62.1), director qualifications, verification of the identity of the person being tested, chain-of-custody documentation for the specimen, and expertise in complex probability calculations, reporting of results, and legal proceedings. HLA typing is time-sensitive, requires 24-h laboratory staffing, and involves complex analysis of the test results. Laboratories performing HLA typing require special accreditation by the American Society for Histocompatibility and Immunogenetics (ASHI) (Table 62.2).
Regulatory Agencies
All laboratories performing clinical testing must comply with numerous regulations. With the advent of CLIA [20], all laboratories are required to implement minimum quality and personnel standards. Proof of meeting these standards (accreditation), which may be done through voluntary accreditation programs offered by professional organizations, is critical to providing high-quality laboratory services. Clinical molecular laboratories are faced with special challenges due to the complex technical issues and ethical considerations involved in DNA-based testing. Several regulatory agencies are currently developing guidelines and standards for molecular pathology tests (Table 62.2). Some of these regulatory agencies and regulations are discussed below.
CLIA and CLIA’03
All clinical laboratories are regulated by CLIA. The US Congress passed CLIA to establish quality standards for all clinical laboratory testing to ensure the accuracy, reliability, and timeliness of patient test results [21]. CLIA is “test-site neutral,” meaning that the same regulations apply to any location performing testing. Every laboratory examining “material derived from the human body for the purpose of providing information for the diagnosis, prevention, or treatment of any disease” is subject to CLIA. Final regulations to implement the law were published in 1992 in the Federal Register [22]. In 2003, new, extensively revised CLIA regulations were published and have been called CLIA’03, also termed the “Final Rules.” The changes include major reorganization and consolidation of the regulations by basing requirements on the flow of a patient sample through the laboratory, updating the requirements to accommodate new technologies, and introducing a new quality control concept termed equivocal quality control (EQC), which empowers manufacturers to design instruments with internal quality assessment systems [23]. By this concept, laboratory directors alone, not manufacturers or regulators, must decide to adopt or eschew ECQ in their laboratory.
The CMS, formerly known as the Health Care Financing Administration (HCFA), in conjunction with the CDC, were originally charged with developing and enforcing the CLIA regulations. CMS continues to oversee much of these regulatory activities, including laboratory registration, on-site inspection, training, and accreditation.
CLIA certificates are based on the complexity of the test methods being performed by a clinical laboratory and are divided into three main categories: waived, moderate complexity, and high complexity. The level of complexity is determined by assigning a numerical score for each test or methodology based on numerous criteria including the knowledge, training, and experience required to perform the test, complexity of reagent and material preparation, characteristics of the operational steps, availability of calibrators, controls, and proficiency testing, equipment and test system troubleshooting, and interpretation of results. Under the CLIA’03 regulations, however, all quality control and quality assessment requirements now apply equally to high- and moderate-complexity laboratories, although some of the personnel qualifications remain more stringent for high-complexity laboratories [23]. These criteria are considered to be key elements in performing clinical testing and can be found on the US FDA Web site (http://www.fda.gov/).
Molecular pathology tests are considered high-complexity tests and as such must comply with CLIA requirements. Some of the requirements for high-complexity tests include qualifications of personnel performing and overseeing the testing, procedure manual specifications, method performance verification specifications, proficiency testing, quality assurance, patient test management, and inspection. CLIA, however, does not provide specific guidelines for molecular testing, and, therefore, each clinical molecular laboratory is responsible for the development of a test and quality management program according to CLIA criteria.
Voluntary Accreditation Organizations
CLIA regulations allow CMS to approve nonprofit, professional organizations that have laboratory testing and inspection standards equivalent to or more stringent than the CLIA regulations to inspect clinical laboratories in place of CLIA inspection. The two major organizations providing CLIA inspections are the Joint Commission on Accreditation of Healthcare Organizations (the Joint Commission), which accredits more than 80 % of the US health care organizations, and the Laboratory Accreditation Program of the College of American Pathologists (LAP-CAP). The majority of clinical molecular laboratories are inspected and accredited by LAP-CAP. Other CLIA accrediting organizations include AABB, American Association for Laboratory Accreditation, American Osteopathic Association, ASHI, and COLA. When a test site meets the accrediting agency’s requirements, as assessed by inspection, the laboratory, in essence, is meeting CLIA requirements and receives a CLIA certificate.
The College of American Pathologists
LAP-CAP accredits only clinical laboratories and not entire health care organizations. LAP-CAP does not base its requirements for accreditation on the different CLIA complexity levels of testing; instead it requires that all testing laboratories adhere to the same guidelines. LAP-CAP lists its requirements and guidelines in checklists, each consisting of a series of laboratory guideline questions. All laboratories must follow the Laboratory General checklist as well as the specific laboratory checklists relevant to the testing performed in the clinical laboratory, such as microbiology, blood bank, and other laboratory specialty sections. In addition to general requirements concerning personnel qualifications, quality control, test performance verification, and operation-related guidelines similar to CLIA, the Molecular Pathology checklist also includes test- and application-specific guidelines. The Molecular Pathology checklist also contains specific standards for tests using NGS methods, and addresses both the testing steps to generate the sequence data, the bioinformatics steps used for sequence analysis and variant calling, and the reporting of results. LAP-CAP requires laboratories to have a procedure manual for each test or process that complies with the GP2-3A standards of the Clinical and Laboratory Standards Institute (CLSI; formerly National Committee for Clinical Laboratory Standards, NCCLS) [24]. Clinical laboratories accredited by the CAP, as required by CLIA, also, for each test performed, must participate in a proficiency testing program when available, or perform internal proficiency checks when an external proficiency testing program is not available. Currently CAP inspections are performed every 2 years and are unannounced, with a self-inspection on alternate years.
The New York State Department of Health
The New York State Department of Health (NYSDOH) provides regulatory oversight of all clinical laboratories performing testing of any type on any specimen from a New York resident. The Clinical Laboratory Evaluation Program (CLEP) at Wadsworth Center in Albany, NY, seeks to ensure the accuracy and reliability of results of laboratory tests on specimens obtained within the state through on-site inspections, proficiency testing, and evaluation of the qualifications of personnel of state permit-holding clinical laboratories. NYS Public Health Law Article 5, Title V, requires the licensure of clinical laboratories by establishing minimum qualifications for directors, and by requiring that the performance of all procedures employed by the clinical laboratories meet minimum standards accepted and approved by the NYSDOH. The New York State Clinical Laboratory Technology Practice Act that became effective in 2008 requires that all clinical laboratory technologists and technicians, cytotechnologists, and histological technicians meet specific training requirements and be licensed by the state. However, because of the high complexity of molecular and cytogenetic testing and the lack of adequately trained staff through medical technology training programs, a limited license in molecular pathology or cytogenetics is provided for training under the direction of the laboratory director with qualification for a full license based on training and experience within a molecular laboratory. The clinical laboratory license requirements have been established for laboratories located in or accepting laboratory specimens originating from NYS. CLEP currently issues annual permits to over 1,000 laboratories in NYS. In addition, CLEP provides, on a biennial basis, approximately 3,100 certificates of qualification (CoQs) to individuals to serve as directors and assistant directors of clinical laboratories, which requires either specific training or experience to oversee testing of specific types across the clinical laboratory. A NYSDOH license is issued by testing category, following an on-site inspection. The clinical laboratory must have someone with a CoQ for each category of testing being performed. Finally, NYSDOH requires any test which is not US FDA-approved or -cleared and performed according to the manufacturer’s instructions to be approved by the NYSDOH. This test review and approval is frequently required of clinical molecular laboratories because fewer of the tests are US FDA-approved or -cleared. Specific NGS test validation guidelines and requirements have been developed by the NYSDOH. More information about CLEP, NYSDOH regulatory oversight programs, and test approval policies can be found on NYSDOH Web site (http://www.wadsworth.org/).
The US Food and Drug Administration
The US FDA oversees regulation of medical devices under the 1938 Federal Food, Drug, and Cosmetics Act and subsequent amendments. Unless specifically exempted, medical devices must “be properly labeled and packaged, be cleared for marketing by the US FDA, meet their labeling claims, and be manufactured under Good Manufacturing Practices (GMP), which is a mandated quality assurance system.” These rules concerning the design, manufacturing, marketing, and surveillance of medical devices can be found in the Code for Federal Regulations (CFR). The US FDA also oversees some of the functions related to laboratory operations, including classification of tests as required by CLIA and review of clinical laboratory test kits or systems.
The US FDA and Genetic Testing
The recent growth of genetic testing, mostly developed and performed by individual clinical molecular laboratories without US FDA review, has raised public concerns about the quality and clinical usefulness of these tests. The Secretary’s Advisory Committee on Genetic Testing (SACGT) was established in 1998 to advise the secretary of the DHHS on medical, scientific, ethical, legal, and social issues raised by the development and use of genetic testing. The SACGT, in conjunction with the Clinical Laboratory Improvement Advisory Committee (CLIAC), recommended that the US FDA provide regulatory oversight of laboratory-developed genetic tests. The vast majority of genetic testing is performed using laboratory developed assays rather than by commercial US FDA-approved or -cleared test kits, raising concerns about the quality and clinical usefulness of these tests. As the first step in developing a review process for LDTs, the US FDA developed a “test review template” for gathering data on the use, performance, interpretation, and reporting of LDTs [25]. Although this template was well received by SACGT, the US FDA was concerned that LTDs were part of medical practice, which is not regulated by the US FDA, and did not develop a review mechanism for LDTs at that time. The successor committee to the SACGT, the Secretary’s Advisory Committee on Genetics, Health, and Society (SACGHS), was formed in 2001 and served for 10 years. The SACGHS studied the regulatory oversight of genetic LDTs, and their report provides an overview of the oversight system [26]. Both the Association for Molecular Pathology (AMP) and the CAP have developed proposals for the oversight and strengthening of LDT regulation, after the US FDA announced in 2009 an intention to abandon its regulatory policy of enforcement discretion toward some LDTs. The US FDA has published preliminary guidelines for the oversight of LDTs which will be based on the complexity of the test, with the most high-risk tests requiring US FDA review.
Analyte-Specific Reagents
Although the majority of the US FDA regulations for clinical assays target commercially developed in vitro diagnostic test kits intended for clinical use, the US FDA issued regulations for commercial reagents used in LDTs in 1997 [27, 28]. Although these regulations are not specific for molecular tests, many tests in the clinical molecular laboratory are developed, validated, and performed without the use of US FDA-approved test kits. These tests are distinct from research assays that have unproven clinical utility and cannot be used for patient care. In 1997, the US FDA developed regulations for manufacturers of reagents used in LDTs, as well as the clinical laboratories using such reagents in their LDTs. These reagents, termed “analyte-specific reagents” (ASRs), serve as the key component for LDTs including molecular pathology tests, and include primers or probes that hybridize to specific DNA sequences. The manufacturers of ASRs are prohibited from making statements about the analytic or clinical performance or the recommended use of the reagents, and validation of tests using ASRs is the responsibility of the laboratory. The laboratory must include a disclaimer in the patient report stating, “This test was developed and its performance characteristics determined by (laboratory name). It has not been cleared or approved by the US Food and Drug Administration.” The result of the ASR regulations has been an increase in the availability of commercial reagents for molecular pathology tests; however, in the past few years the US FDA has been moving towards in vitro diagnostics (IVDs) and eliminating ASRs whenever possible [29].
Elements of Laboratory Design
Successful laboratory operation requires optimal utilization of space, appropriate equipment, qualified personnel, adequate laboratory information system (LIS) support, and financial management.
Facilities
The power of molecular testing is achieved by the use of PCR as the main diagnostic procedure. A serious problem in the clinical use of PCR is false-positive amplification due to PCR contamination. Because the products of PCR amplification, called an amplicons, can serve as the substrate for the generation of additional PCR products, and because PCR amplification produces a very large number of amplicons, the potential contamination of subsequent amplifications of the same target sequence with amplicons resulting in inaccurate results is immense without the use of proper controls. This kind of contamination has been termed “carryover” to differentiate it from contamination by genomic DNA. This potential for PCR product contamination requires special precautions. Therefore, the single most important step in designing laboratory space for molecular pathology testing is meticulous attention to the physical separation of the pre-PCR and post-PCR work areas to minimize PCR contamination [30].
The pre-PCR area, also referred to as a “clean room” or “pre-amplification area,” is used for the pre-PCR steps of sample receiving and processing, including extraction of nucleic acids and setting up of amplification reactions. The post-PCR area, also considered a “dirty room” or “post-amplification area,” is used for PCR amplification and analysis of PCR products. The pre-PCR laboratory ideally contains separate work areas for nucleic acid extractions and PCR setup to minimize cross-contamination of patient nucleic acid samples. Additionally, a containment unit, such as a biosafety cabinet, may be used for setting up PCR reactions. These cabinets usually are equipped with ultraviolet light for reducing DNA contamination of the work area at the end of reaction setup. Some clinical molecular laboratories prefer to further separate the various steps of the pre- and post-PCR areas into separate rooms.
If possible, the air system for the pre-PCR and post-PCR areas should be independent. Otherwise, air filters can be installed in the inflow to the pre-PCR room air system, which can be cleaned routinely. An additional measure to limit PCR contamination is to maintain the pre- PCR and post-PCR rooms at different air pressures. The pre-PCR area is maintained at positive pressure, reducing the entrance of airborne contaminants and PCR products, while the post-PCR room is maintained at negative pressure, reducing contaminants that exit the room. If installation of a pressure system is not possible, then the two work areas can be located at a relative distance from each other.
To comply with universal blood-borne pathogen precautions, nucleic acid extraction from patient specimens can be performed in a closed safety cabinet. Specimens known to contain infectious agents, such as HIV-1 and HCV, can be processed in a separate biological safety hood. If organic solvents such as phenol, chloroform, or xylenes are used, a chemical fume hood should be available in the work area. Within the pre-PCR laboratory, RNA extractions should be physically separated from DNA extraction if the DNA extraction procedure uses RNase, which can degrade RNA. All work areas should be cleaned daily with 10 % bleach solution. In addition to pre-PCR and post-PCR areas, the clinical molecular laboratory also may have a neutral reagent preparation area (unless reagents are separately prepared in both rooms) and a data management room for the processing, review, and reporting of test results, which also may be performed in the post-PCR area.
To maximize use of space, equipment, technical expertise, and trained technologists, all types of molecular pathology testing (genetics, infectious disease, cancer, and identity testing) can be consolidated into a single laboratory. Implementing a core molecular pathology laboratory can reduce labor costs by approximately 30 % [31] as well as reduce equipment costs and space requirements due to duplication of these resources in several laboratories. As more infectious disease testing systems have become available, molecular infectious disease testing is more commonly performed in the microbiology laboratory rather than a molecular laboratory, although this is institution-specific.
PCR Contamination Control
In addition to physical separation of the pre-PCR and post-PCR areas, specific procedures are recommended for use of PCR in the clinical laboratory. To ensure that PCR products are not transferred from the post-PCR to the pre-PCR area, each should contain separate supplies and equipment. Pipettes, instruments, and supplies should not be transferred from the post-PCR to the pre-PCR area. This separation of supplies and equipment also dictates the workflow in the laboratory. PCR samples are first set up in the pre-PCR area and then transferred to the post-PCR area for PCR amplification and post-PCR analysis. Worksheets, once moved to the post-PCR area for post-PCR analysis, can no longer be taken back into the pre-PCR area and must be processed and filed in the post-PCR area or a neutral area.
Reagents and solutions for sample preparation and PCR work should be prepared with type I water, which is defined by specific ion, pH, and contaminant limitation requirements. All reagents, except primers, deoxynucleotide triphosphates (dNTPs), and enzymes, should be purchased as sterile reagents or sterilized to prevent bacterial growth. Reagents, including autoclaved type I water and 10 mM Tris–1 mM EDTA buffer (TE) used to dissolve DNA, should be stored as small aliquots to minimize the number of samplings from the same aliquot. Similarly, oligonucleotides used for PCR amplification should be synthesized and purified in a clean environment. To minimize the number of sample transfers and the chance of sporadic contamination and errors, PCR reagents, including primers, dNTPs and buffers, can be combined into a “premixture master mix” (MM), divided into aliquots that are appropriately labeled, and stored at −20 °C [30]. Before using for clinical testing, a new lot of MM must be tested to ensure that it specifically amplifies the target sequence without producing PCR products in the negative “minus DNA” controls, as is done for new lots of any reagents in the clinical laboratory. A “minus DNA” negative control not containing any specimen DNA is tested last in each run to ensure the lack of DNA or amplicon contamination in the PCR reagents.
Although carryover of amplified sequences contributes to the majority of false positives, cross-contamination can occur between samples, especially from specimens containing a high level of the target sequence such as occurs with infectious disease specimens. Consequently, precautions must be taken not only during the setup of PCR amplification reactions but also in all aspects of sample handling, from sample collection to sample extraction. For good laboratory practice, gloves should be changed frequently, at least when entering or reentering the pre-PCR area and whenever the technologist notes the gloves to have been contaminated. Technologists should wear protective clothes to prevent operator-borne spread of contaminating DNA products, with separate protective clothes (i.e., laboratory coats) for the pre-PCR and post-PCR areas.
To minimize aerosolization of PCR products, PCR tubes should be pulse-centrifuged before opening. Microcentrifuge tube caps should be opened using both hands, and not flicked open with the thumb of the same hand, to prevent aerosolization. Because cross-contamination of pipettors can lead to false-positive results, and to minimize aerosolization, aerosol barrier tips are used for all pre-PCR steps. MM and other non-sample components should be added to the reaction tubes before the specimen DNA is added; DNA should be added last and each tube capped before the technologist proceeds to the addition of specimen DNA to the next sample. Positive and negative control DNA or RNA should be the last reaction tubes to be set up, after the patient sample reactions are set up and closed.
Another approach to minimize carryover of PCR products is to synthesize all PCR products with deoxyuridine triphosphate (dUTP) in place of deoxythymidine triphosphate (dTTP). Prior to amplification, the PCR reactions are treated with uracil-N-glycosylase (UNG), which will degrade uracil-containing amplicons, allowing amplification only from thymidine-containing target DNA [32]. Some commercial test kits incorporate this process into the kits.
Finally, automation of DNA extraction as well as PCR setup by the use of automated, robotic nucleic acid extractors and liquid handlers has greatly facilitated standardization of testing, reducing PCR contamination, and increasing overall testing accuracy and reliability of results. Real-time PCR technology, in particular, provided additional tools for PCR contamination control by combining PCR amplification with amplicon analysis and thus eliminating aerosolization of PCR products due to manipulation of PCR products [33].
Equipment
The equipment used for clinical molecular testing is rarely manufactured for clinical use. Most equipment is designed for research purposes and adapted for clinical use [30]. Nucleic acid extractions may be performed manually, although for higher volume testing, automated extraction instruments are available with improved reproducibility and reduced technologist time for the clinical laboratory. PCR can be set up manually in biosafety cabinets; for higher-volume testing, robotic liquid handling systems are available. Robotic systems, however, have reagent dead volumes that increase reagent wastage. Thermal cyclers are standard equipment in the molecular pathology laboratory, and the number required depends on the work volume. Post-PCR analysis is highly variable, and is based on the types of PCR product analysis being performed.
Other specialized equipment for post-PCR analysis includes automated sequencers or capillary electrophoresis instruments, chemiluminescent or colorimetric plate readers, and real-time PCR instruments. However, numerous clinical tests have been approved by the US FDA. These assays use commercial kits and specialized equipment for automated extraction and viral load quantitation, usually supplied by the test kit manufacturer [34]. Recently, with the advent of NGS technology, high-throughput sequencing instruments have been developed for simultaneous analysis of thousands of genes to exomes to genomes [35, 36]. Guidelines and checklists for NGS test validation and performance have been developed by both the CAP and NYSDOH [37]. Because of the high level of testing complexity and the concern of PCR contamination, researchers or personnel not trained in clinical molecular testing requirements and processes should not use the clinical molecular equipment.
Automated platforms for molecular tests are becoming available and are more significant for the clinical laboratory as test volumes increase. Automated systems include extraction systems, as well as real-time thermal cyclers that combine PCR amplification and detection. Automated systems are routinely and widely used for viral load testing, such as for HIV-1 and HCV viral load testing. Automated instrumentation can greatly reduce TAT, human error, and technologist time.
Because nonclinical instruments are used for clinical testing, they require rigorous surveillance and prompt technical support by manufacturers who are not always aware of the critical clinical testing issues. In addition, these instruments require ongoing maintenance. Most maintenance procedures are defined by the manufacturer and include temperature checks of the PCR wells for thermal cyclers and calibration of pipettors. In the absence of manufacturer’s guidelines, the laboratory should set up its own maintenance program in consultation with the manufacturer.
Laboratory Personnel
Personnel Qualifications
Effective laboratory operation requires well-trained staff and a good management team. CLIA regulations specify that for high-complexity testing, individuals for the positions of director, technical supervisor, and testing staff must have specific qualifications. The director, as named on the CLIA certificate, is required to be a licensed doctor of medicine, osteopathy, or podiatry or have a doctoral degree in one of the biological, chemical, physical, or medical sciences, and have 2 years of experience supervising a high-complexity laboratory. In NY, laboratories holding or applying for a NYSDOH clinical laboratory permit must also have a director or assistant director holding a CoQ for each testing category. To qualify for a CoQ, an individual must comply with CLIA requirements, in addition to having board certification in the appropriate specialty and working experience, with at least 2 years in current methodology in each category sought and in general laboratory management documented in the form of recommendation letters. The laboratory director is responsible for the overall operation and administration of the laboratory either personally or through oversight of assistant directors. Overall operation and administration of the laboratory includes the development, validation, and implementation of new tests, current procedure manuals, quality control, and training programs for staff, and teaching residents.
The technical supervisor establishes the quality standards of the laboratory by selecting and monitoring methods and instruments and documenting the competency of laboratory personnel, while the general supervisor provides day-to-day supervision of testing, personnel, and reporting of results. The qualifications for these positions range from a licensed doctor to an individual holding a bachelor’s degree in science plus specific training or experience or both. The laboratory also may have clinical consultants, who must hold a medical or doctoral degree in a relevant discipline with appropriate experience in molecular testing. The Genetic Testing Good Laboratory Practices Workgroup of CLIAC, created in 1998, reviewed personnel qualifications and recommended including specific genetic experience and board certification for supervisors who oversee genetic testing [25].
Testing personnel who perform high-complexity testing must have an associate’s degree in laboratory science or medical laboratory technology, or education and training equivalent to an associate’s degree. Laboratory technologists are responsible for all tasks associated with the daily operation of the laboratory, including specimen receiving and processing, testing, identifying problems and troubleshooting, maintaining equipment, and documenting quality control procedures. In addition, they are required to work according to established procedures in adherence with the quality control standards implemented in the laboratory to comply with CLIA, CAP, or other regulations. Because very few molecular tests are approved by the US FDA, molecular pathology technologists perform much of the development of new tests, as well as validation of commercial molecular kits used for clinical molecular testing.
Because of the high level of desired expertise, technologists also need to be encouraged to attend educational courses to obtain the appropriate background in molecular pathology and the skills required for performing these tests. For the same reason, it is also crucial for the senior management of the laboratory to acknowledge the staff, encourage personal growth, and create new mechanisms for promotion. Although some of these recommendations represent the authors’ personal views and may present a burden in the stringent financial environment of many health care institutions, the cost of training new technologists is higher than that of creating an environment that promotes job satisfaction and employee retention.
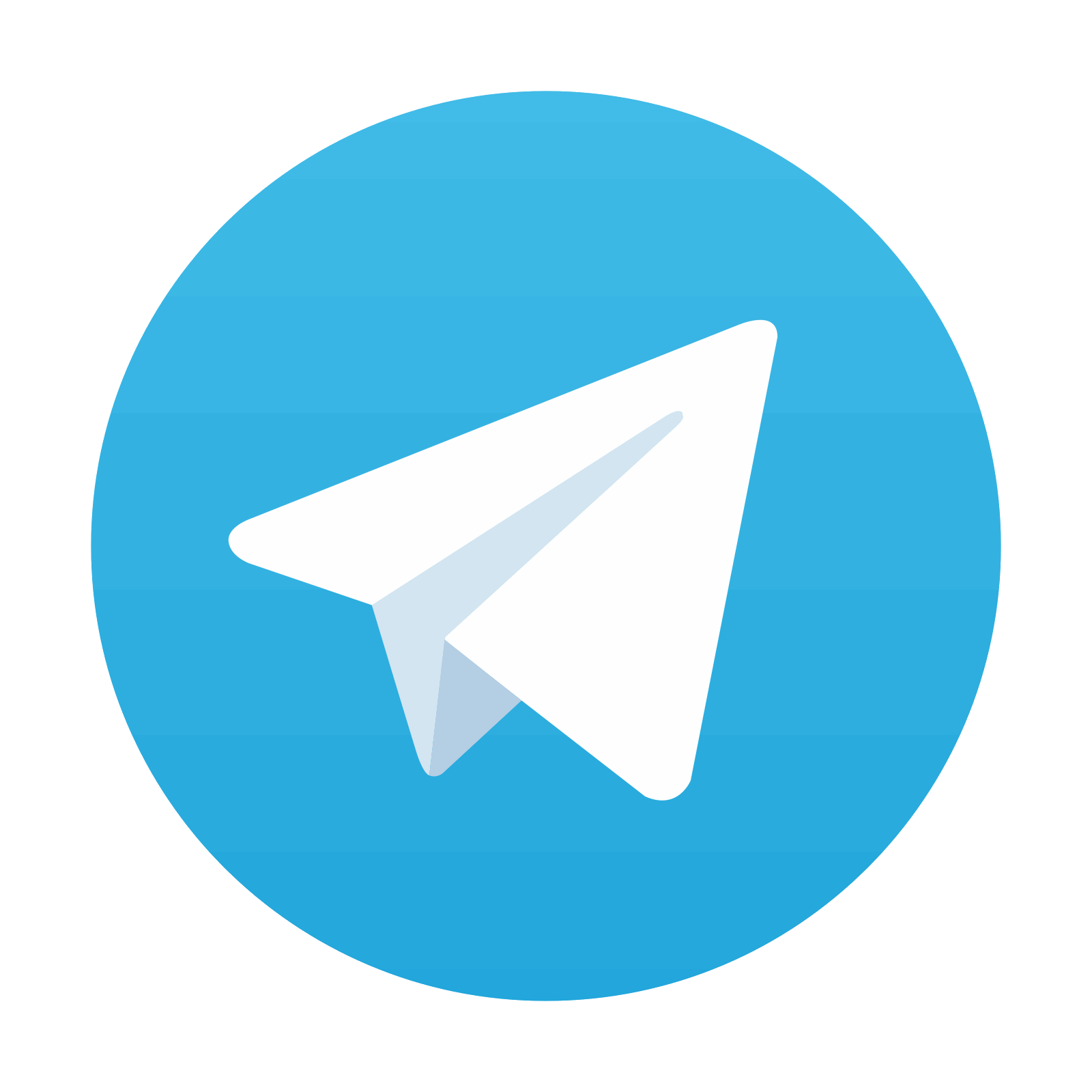
Stay updated, free articles. Join our Telegram channel

Full access? Get Clinical Tree
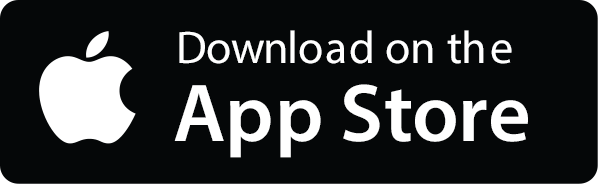
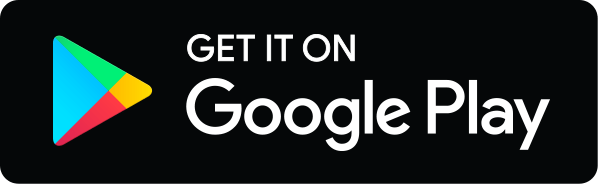