INTRODUCTION
Local anesthetics (LAs) are a class of locally applied chemicals, with similar molecular structures, that can disrupt nerve transmission, inhibit the perception of sensations (importantly, pain), blunt autonomic activity, and prevent movement. They are used for a variety of clinical applications and delivered via many different approaches, ranging from topical application for burns and small cuts, to injections during dental care, to epidural and intrathecal (“spinal”) blocks during obstetric procedures and major surgery.
Cocaine, the first local anesthetic, comes from the leaves of the coca shrub (Erythroxylon coca). It was first isolated in 1860 by Albert Niemann, who noted its numbing powers. In 1886, Carl Koller introduced cocaine into clinical practice as a topical ophthalmic anesthetic. However, its addictive properties and toxicity prompted the search for substitutes. Procaine, the first of these substitutes to prove clinically useful, was synthesized in 1905. Known as Novocain®, it is still used today, although less frequently than some more recently developed LAs.
Local anesthetics exert their effect by blocking voltage-gated sodium channels, thus inhibiting the propagation of action potentials along neurons (see Chapter 8, Principles of Cellular Excitability and Electrochemical Transmission). By inhibiting action potential propagation, LAs prevent transmission of information to and from the central nervous system (CNS). LA actions are not selective for pain fibers; they can also block conduction in other sensory fibers as well as motor and autonomic fibers and action potentials in skeletal and cardiac muscle. This nonselective blockade can serve other useful functions (see Chapter 24, Pharmacology of Cardiac Rhythm) or can be a source of toxicity.
EM is a 24-year-old graduate student in organic chemistry. While he is working in the lab one evening, a bottle of concentrated hydrochloric acid falls from a shelf above him and smashes his left hand against the lab bench before it shatters and cuts his index finger. Although he reflexively jerks his hand away, some of the acid falls on the fingertips of his hand. He immediately feels a localized sharp pain where the bottle cut his hand followed by a diffuse stinging pain where the acid touched his skin. Although he begins to rinse his hand under running water, he still feels a slowly developing, burning, throbbing ache throughout his hand. EM anxiously telephones 911 and is transported to the emergency department.
On exam in the emergency department, he is noted to have a 1.5-cm laceration of his left index finger and isolated areas of red, tender skin with partial-thickness burn on the fingers exposed to acid. He is in severe pain and his laceration will need to be sutured. After continued irrigation of the acid-exposed areas of his hand, topical EMLA cream (eutectic mixture of local anesthetic—a combination of lidocaine and prilocaine) is applied to the burned areas and a digital nerve block of his index finger is performed. After the area is made sterile, 1 cc of 2% lidocaine without epinephrine is injected into the medial and lateral aspects of his finger near the web space to block the digital nerves. The lidocaine injection is directed toward both the palm and the back of his hand in order to block both the dorsal and ventral contributions to the digital nerves that run down the lateral and medial aspects of the finger. He notices that the sharp stinging pain from his cut abates first, followed by the dull throbbing ache in his finger. EM then loses sensation to light touch on his finger and later notes that he cannot feel the needle piercing his skin as the sutures are applied. EM’s wounds heal over the next 2 weeks, and the pain, now primarily a result of posttraumatic inflammation, is well controlled with oral ibuprofen. He is able to return to work.
Questions
1. Why did EM initially experience a stinging pain before the dull aching pain, and why did the stinging pain resolve more quickly than the dull pain after lidocaine administration?
2. Why is epinephrine sometimes administered with lidocaine, and why was it not co-administered in this case?
3. What is lidocaine’s mechanism of action? To which broader class of drugs does it belong?
Nociception occurs following the activation of particular primary sensory nerve fibers (nociceptors) by a noxious stimulus representing potential or actual tissue damage. Noxious stimuli include extreme temperatures, intense mechanical perturbations, harsh chemical environments, and certain molecules released by damaged cells (Fig. 12-1). Nociceptors are activated by noxious stimuli at free nerve endings, which are located in the skin, deeper tissues such as joints and tendons, and viscera. When activated, nociceptors transmit impulses from the periphery to the dorsal horn of the spinal cord, where the information is subsequently processed through synaptic circuitry and transmitted to various parts of the brain. Thus, nociceptors are the first, or primary, cells in a chain of neurons ultimately responsible for pain perception. Because nociceptors, among other sensory fibers, transmit information toward the brain, they are termed afferent neurons.
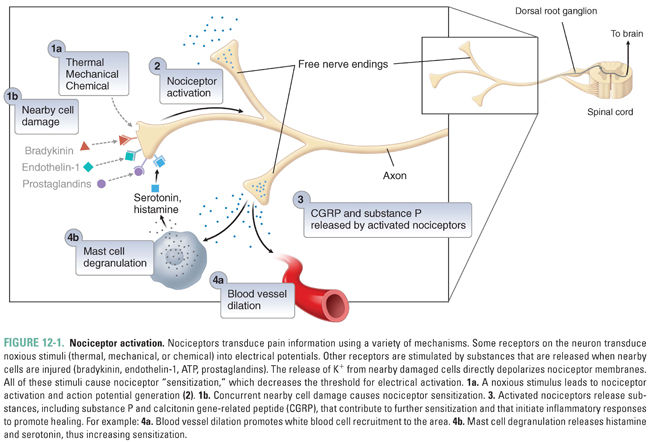
Nociceptors are normally not activated by nonnoxious stimuli such as a breeze against one’s skin or a handshake (the nerves that are activated in these circumstances are termed tactile or low- or medium-threshold mechanoreceptors). When a noxious stimulus is present at a free nerve ending of a nociceptor, it activates specific transducing receptors that generate inward currents and depolarize the primary neuron (Fig. 12-1; also see Chapter 8). If a noxious stimulus has an intensity above the nociceptor’s threshold (e.g., above a certain temperature), an action potential (AP) will be generated. Sensory information is almost always encoded by trains of APs, and the frequency of APs in a train increases as the stimulus intensity increases (e.g., at higher temperatures). If impulses from a nociceptive afferent nerve are sufficiently frequent, or if multiple nociceptors are activated, then the generating stimulus is perceived as “painful.” Although lower stimulus intensities may not be perceived, these “subliminal” sensory inputs are still transmitted to the CNS and can influence future sensory coding. Explicitly painful stimuli are able to induce long-lasting increases in responsiveness of the CNS and thus to effect a “memory” of pain. Such central sensitization is one component of chronic pain (see Chapter 18, Pharmacology of Analgesia).
Transmission of Pain Sensation
In their simplest form, neurons are composed of dendrites, a cell body, and an axon. Axons transmit information along the neuron to dendrites, which synapse with other neurons. Nerve axons are classified as A-fibers, B-fibers, or C-fibers, ranked in the order of decreasing diameter and having respectively decreasing conduction velocities. A-fibers and B-fibers are myelinated; while C-fibers are nonmyelinated, they are still encased in a peripheral glial Schwann cell that collects up to 10 axons in a Remak bundle (Table 12-1). Myelin is composed of the cell membranes of supportive cells in the nervous system (Schwann cells peripherally and oligodendrocytes in the CNS) that wrap tightly many times around neuronal axons to create an electrically insulating sheath that increases the velocity of impulse transmission. Regular, narrow spacings between the myelinated segments, called nodes of Ranvier, contain the voltage-gated Na+ channels and are the locations where inward ionic current drives the APs of myelinated fibers.
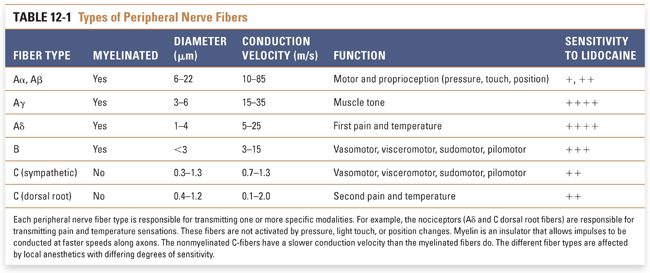
Distinct types of nociceptors are activated by specific noxious stimuli and transmit information along characteristic classes of axons. Nociceptor axons are generally either Aδ–fibers or C-fibers. Thermal nociceptors are activated at temperatures above 45°C (noxious heat, C-fibers) or below 5°C (noxious cold, Aδ-fibers). High-threshold mechanical nociceptors exclusively transmit information indicating injurious force on the skin (Aδ- and some Aβ-fibers). Polymodal nociceptors are activated by thermal, mechanical, and chemical stimuli (C-fibers).
Myelinated Aδ-fibers transmit impulses faster than nonmyelinated C-fibers do (Fig. 12-2). An Aδ-fiber transmits impulses along its axon at a rate of 5–25 meters per second (m/s), while C-fibers transmit impulses at roughly 1 m/s. Impulse transmission is slower in C-fibers primarily because these fibers are nonmyelinated.
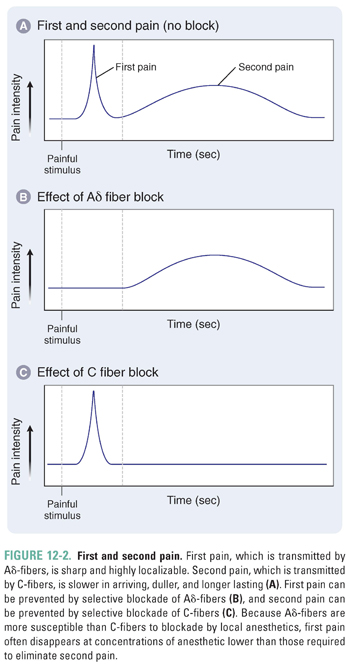
The Aδ-fibers transmit what is called first pain, which is perceived within seconds after an injury, is sharp in quality, and is highly localized on the body. The density of nociceptor Aδ-fibers is high on the fingertips, face, and lips but relatively low on the back. Aδ-fibers require a weaker stimulus for excitation than C-fibers do.
The C-fibers transmit what is called second pain. Second pain is slower to develop, appearing many seconds after an insult, but lasts much longer than first pain; it often feels dull, throbbing, or burning, is poorly localized, and endures long after the stimulus ends. C-fiber nociceptors are often polymodal, which means that a single fiber can be activated by noxious thermal, mechanical, and chemical stimuli. In the case above, EM experienced an initial stinging first pain transmitted by myelinated Aδ-fibers and a later burning and throbbing second pain transmitted by unmyelinated C-fibers. Afferent activity conducted by C-nociceptors has a pronounced potential to create central sensitization, and it also activates regions of the brain, such as the amygdala and prefrontal cortex, that are involved in the affective, subjective aspect of pain perception.
Pain is the physical or emotional distress due to perceived actual or potential tissue injury. Pain perception is a complex process that involves activation of nociceptive neurons by a noxious stimulus and central regulation and interpretation of the nociceptive signals in the CNS. Impulses generated in the skin by nociceptor activation are conducted to the dorsal horn of the spinal cord. In the dorsal horn, the nociceptors form synapses with interneurons and second-order neurons. The second-order neurons travel in the lateral areas of the spinal cord and project mainly to the thalamus, a gray matter structure just superior to the brainstem. The thalamus has cells that project to the somatosensory cortex of the parietal lobe and to other areas of the cortex (Fig. 12-3). Nociceptive signals as well as information from non-nociceptive afferent nerves are integrated centrally and interpreted as pain. The subjective sensation of pain is also altered by less tangible central factors including emotional state and higher executive function. The CNS uses efferent projections within the brain and spinal cord to modulate the incoming nociceptive signals and thus to modify pain perception (see Chapter 18). For example, an athlete focused on an important game might not feel the pain of an injury intensely until after the game is over. Her brain modulates the effect of the input so that the same stimulus is perceived as less painful at certain times than at others.
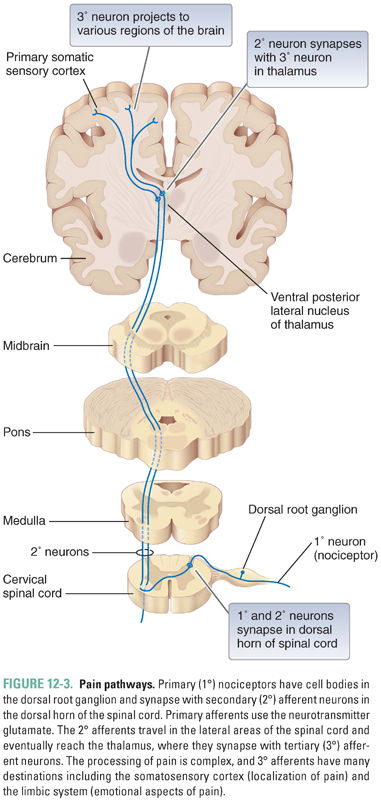
Analgesics are specific inhibitors of pain pathways, whereas local anesthetics are nonspecific inhibitors of peripheral sensory (including pain), motor, and autonomic nerve transmission. Analgesics have actions at specific receptors on primary nociceptors and in the CNS (see Chapter 18). For example, opioid analgesics activate opioid receptors, which signal cells to increase potassium conductance in postsynaptic neurons and to decrease calcium entry into presynaptic neurons. By these mechanisms, postsynaptic excitability and presynaptic transmitter release are reduced, and pain sensations are not transmitted as effectively to the brain (or within it). Importantly, the transmission of other sensations and motor information is not affected.
Local anesthetics act by a different mechanism. These agents inhibit conduction of action potentials in all afferent and efferent nerve fibers, usually in the peripheral nervous system. Thus, pain and other sensory modalities are not transmitted effectively to the brain, and motor and autonomic impulses are not transmitted effectively to muscles and peripheral organs.
PHARMACOLOGIC CLASSES AND AGENTS
Local anesthetics prevent signal transmission by blocking the function of sodium channels in excitable tissues. All LAs share this common mechanism of action and have similar chemical structures. The next section highlights the general principles of LA pharmacology and how the molecular properties of LAs affect their function. Specific LA agents are discussed at the end of the chapter.
Molecular Determinants of Local Anesthetic Action
All local anesthetics have three structural domains: an aromatic group, an amine group, and an ester or amide linkage connecting these two groups (Fig. 12-4). Local anesthetics are structurally classified as ester-linked LAs or amide-linked LAs. As discussed below, the structure of the aromatic group influences the hydrophobicity of the drug, and the nature of the amine group influences the charge of the drug. Both features define the rate of onset, potency, duration of action, and adverse effects of an individual local anesthetic.
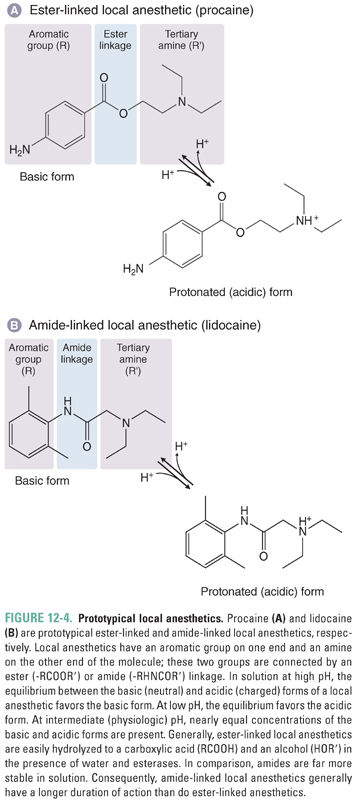
To be effective, a local anesthetic must partition into, diffuse across, and finally dissociate from the membrane into the cytoplasm; the compounds most likely to do so have moderate hydrophobicity. All local anesthetics contain an aromatic group that gives the molecule much of its hydrophobic character. Adding alkyl substituents on the aromatic ring, or on the amino nitrogen, also increases the hydrophobicity of these drugs.
Biological membranes have a hydrophobic interior because of their lipid bilayer structure. The hydrophobicity of an LA molecule affects the ease with which the drug passes through nerve cell membranes to reach its target site, which is the cytoplasmic side of the voltage-gated sodium channel (Fig. 12-5). Molecules with low hydrophobicity partition very poorly into the membrane because their solubility in the lipid bilayer is so low; such molecules are largely restricted to the polar aqueous environment. As the hydrophobicity of a series of drugs increases, the concentration in the membrane and the correlated permeability of the drugs through the cell membrane also increase. However, at a certain hydrophobicity, this relationship reverses, and a further increase in hydrophobicity results in a decrease in permeability. This somewhat paradoxical behavior occurs because molecules that are extremely hydrophobic partition so strongly into the cell membrane that they remain there. The same strong hydrophobic forces that concentrate such molecules in the cell membrane cause them to dissociate very slowly from that compartment.
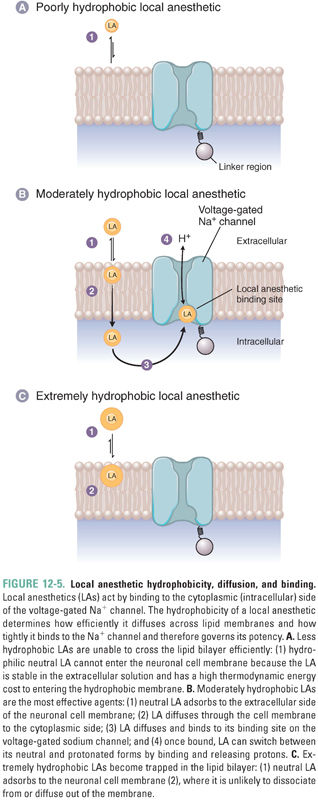
The LA binding site on the sodium channel contains both hydrophilic and hydrophobic residues that are located on transmembrane helices in three of the four domains that form the channel’s pore. The fit is not very tight, however, resulting in relatively low LA potency (IC50 of 10−3 to 10−5 M) and only weak stereoselectivity. In general, more hydrophobic drugs bind more tightly to the target site, increasing the potency of the drug. However, because of the practical need for the drug to diffuse across several membranes in order to reach the target site (see below), LAs with moderate hydrophobicity are the clinically effective molecules. In addition, excessively hydrophobic drugs have limited solubility in the aqueous solutions of their pharmaceutical formulation, and even the molecules that do dissolve remain in the first membrane that is encountered, never reaching the target site in the axon membrane (despite their high affinity for that site). Indeed, access of LAs from the injection site to the axon is so poor, due to permeability barriers and removal by the local circulation, that LA concentrations 20- to 50-fold higher than those effective on an isolated nerve must be injected locally for a successful block.
The amine group of a local anesthetic can exist in either the protonated (positively charged; acid) form or the deprotonated (neutral; base) form.
The pKa is the pH at which the concentrations of a base and its conjugate acid are equal. LAs are weak bases; their pKa values range from about 8 to 10. Thus, at the physiologic pH of 7.4, substantial amounts of both the protonated form and the neutral form coexist in solution. As the pKa of a drug increases, a larger fraction of molecules exists in solution in the protonated form at physiologic pH (see Chapter 1, Drug–Receptor Interactions). Protonation and deprotonation reactions are very rapid in solution (103 sec−1), but drugs in membranes or bound to proteins are protonated and deprotonated more slowly.
The neutral forms of LAs diffuse across membranes much more easily than the positively charged forms do. However, the positively charged forms bind with much higher affinity to the drugs’ target binding site. This site is located in the pore of the voltage-gated sodium channel and is accessible from the intracellular, cytoplasmic entrance of the channel (Fig. 12-5B). Thus, moderately hydrophobic weak bases are effective as local anesthetics because, at physiologic pH, a significant fraction of the weak base molecules are in the neutral form, which, because of its moderate hydrophobicity, can rapidly diffuse across membranes to enter nerve cells. Once the drug is inside the cell, it can then readily gain a proton, become positively charged, and bind to the sodium channel. The neutral species of LAs can also bind to the channel, but the dissociation of this species from the channel is much faster and its affinity is correspondingly lower. Some nonionizable LA drugs, such as benzocaine, are permanently neutral but are still able to block sodium channels. For these drugs, however, the block is weak and rapidly reversible.
Mechanism of Action of Local Anesthetics
Anatomic Factors in Impulse Blockade
The peripheral nerve is composed of a collection of different types of nerve fibers (A-, B-, and C-fibers) surrounded by three protective membranes, or sheaths: the epineurium, perineurium, and endoneurium. Local anesthetic molecules must pass through these sheaths, which present the same permeation-limiting barriers as the nerve cell membranes, considered above, before they can reach the neuronal membranes to block conduction (Fig. 12-6). The sheaths are made up of connective tissue and cell membranes. LAs are injected outside the most external sheath, the epineurium, to avoid mechanical damage to the nerve, but the major barrier to LA penetration into the nerve is the perineurium, an epithelium-like tissue that bundles axons into separate fascicles. Recall that LAs affect not only nociceptors but also other afferent and efferent, somatic, and autonomic nerve fibers. All of these fibers may be contained within a peripheral nerve, and conduction in all fibers can be blocked by local anesthetics. This is why, in the introductory case, EM experienced not only loss of pain sensation but also a more complete block of all sensation in his left index finger.
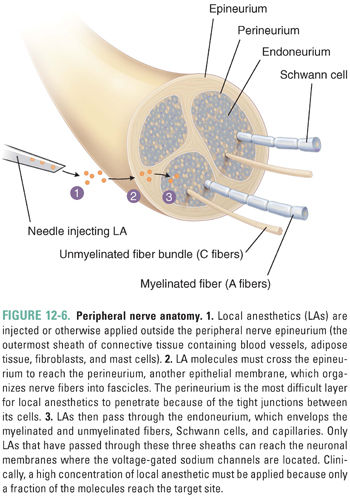
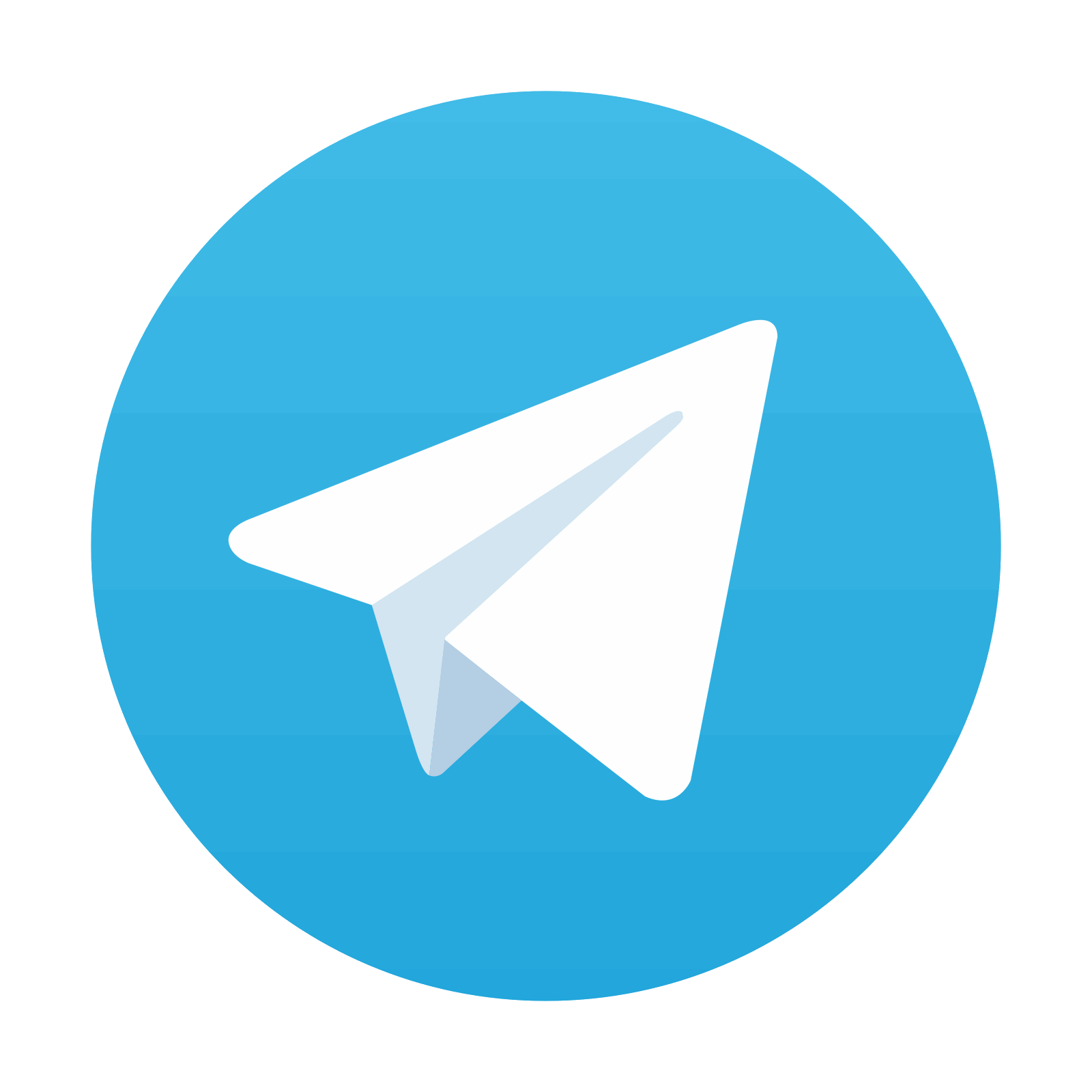
Stay updated, free articles. Join our Telegram channel

Full access? Get Clinical Tree
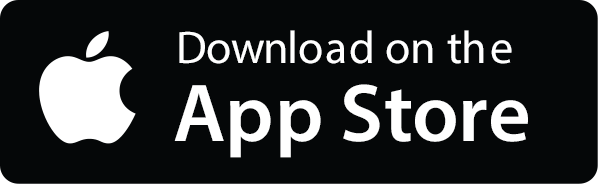
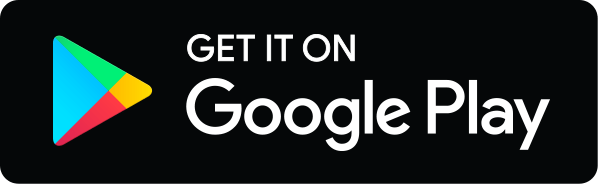