Immunization Against Viral Diseases
Barney S. Graham
James E. Crowe Jr.
Julie E. Ledgerwood
Both innate and adaptive immune responses mediate host resistance to virus infection. Elements of the innate immune system, especially type I interferons (IFNs), strongly influence the clinical outcome of a virus infection. Many viruses have evolved mechanisms to inhibit or evade innate immune responses, indicating the importance of those defenses in limiting virus infection. Innate immune responses also mediate important effects during the induction phase of an immune response and may influence the magnitude and composition of adaptive immune responses. Although memory or “training” of natural killer (NK) cells may occur in response to some antigens (Ags), NK cells do not have mechanisms for somatic diversification of their recognition receptors and are limited to germline sequence.315,343 The capacity for somatic diversification of B-cell receptors (BCRs) and T-cell receptors (TCRs), selection, and long-term memory are central properties of adaptive immunity. Therefore, vaccine-induced immunity that is established in advance of virus infection relies primarily on adaptive immune responses for protective efficacy. Vaccination depends critically on the properties of Ag recognition, activation, expansion, memory, trafficking, and effector functions inherent to lymphocytes. Subtle influences of vaccination on the timing, magnitude, specificity, quality, and location of responding lymphocytes and their effector molecules determine whether exposure to a viral pathogen results in infection, protection, subclinical infection, mild disease, or exaggerated disease expression. The extent to which vaccine-induced immunity is successful in an individual can determine the spread of virus within a population (e-Fig. 14.1). Thus, vaccines have a profound effect on public health through the control of epidemics (e-Fig. 14.2). An antigenic stimulus that elicits a virus-specific adaptive immune response that can be recalled during subsequent virus infection defines it as an immunization. A vaccination is an antigenic stimulus delivered by intention.
Viral Antigens Recognized by the Immune System
Development of an immune response to Ags present on the surface of virions or virus-infected cells is often critical for immunity against virus infection. Antibody (Ab) responses to surface viral protein or glycoprotein Ags often effectively limit infection of new cells and thus spread of the virus. Internal viral Ags can be important targets for T-lymphocyte responses that clear virus-infected cells; however, these responses alone cannot prevent subsequent infections (e-Fig. 14.3). The relative
importance of Abs to surface proteins and of T-cell responses to internal proteins in influenza virus was illustrated by studies of the 1957 Asian influenza pandemic and the 1977 “Russian” influenza epidemic. The 1957 Asian influenza A (H2N2) virus contained surface glycoproteins (the hemagglutinin [HA] and the neuraminidase [NA]) that were novel, such that the human population had not been exposed to strains containing these or related surface Ags. In contrast, the major internal proteins of the new H2N2 virus, the nucleoprotein and the matrix protein, were closely related to corresponding Ags of previous strains of subtype H1N1 to which most individuals had been exposed. Although recent infection with heterotypic viruses may have diminished the level of illness severity in adults,126 the population’s prior immunological experience with related internal Ags did not prevent the 1957 Asian H2N2 influenza A virus from causing a major pandemic.303 In contrast to this experience, observations following the re-emergence of the H1N1 virus in 1977 indicated that homotypic humoral immunity provided a high level of resistance that lasted for decades. Similarly, humans exposed to the 1918 influenza H1N1 virus as infants sustained circulating memory B cells encoding neutralizing Abs to the HA into the 10th decade of life,467 and these Abs cross-reacted with the 2009 H1N1 pandemic strain and provided protection.233,462 Induction of cross-reactive Abs to influenza may sometimes be harmful. For example, immune complexes with complement deposition have been demonstrated in lung tissues of victims of pandemic influenza.287 Additional evidence for the importance of responses to influenza A surface glycoproteins was provided by passive and active immunization studies in mice. Monoclonal antibodies (mAbs) directed against the HA or NA proteins protected mice against challenge with virulent wild-type virus, whereas mAbs specific for the internal nucleoprotein or matrix protein did not alter the course of disease.303 Immunization with recombinant vaccinia viruses expressing each of the 10 known influenza A gene products showed that only recombinants expressing the HA or the NA glycoprotein induced resistance to virus challenge.124 Whereas the vaccinia vector-induced CD8 T-cell–mediated immunity specific for internal viral proteins provided some measure of heterosubtypic cross-reactivity against influenza challenge,125 the aggregate data suggest that a successful strategy of immunoprophylaxis against most virus infections requires the generation of an immune response to the surface Ags displayed on virions and on virus-infected cells. Other viruses (such as poxviruses, herpesviruses, filoviruses, some respiratory viruses, and lentiviruses) that have a more complex pathogenesis may require vaccines that induce more complex immune responses for protection from infection or control of disease. Many of these viruses possess the capacity for persistence, infect immunoprivileged sites, use sophisticated immune evasion strategies, directly infect their target organ without a requirement for viremia, or cause disease by eliciting an aberrant host response. Vaccine development for these viruses probably will require the induction of neutralizing Abs against surface proteins to protect against infection in addition to T-cell responses against structural and regulatory proteins to control virus spread and to modulate the composition of the immune response.167
importance of Abs to surface proteins and of T-cell responses to internal proteins in influenza virus was illustrated by studies of the 1957 Asian influenza pandemic and the 1977 “Russian” influenza epidemic. The 1957 Asian influenza A (H2N2) virus contained surface glycoproteins (the hemagglutinin [HA] and the neuraminidase [NA]) that were novel, such that the human population had not been exposed to strains containing these or related surface Ags. In contrast, the major internal proteins of the new H2N2 virus, the nucleoprotein and the matrix protein, were closely related to corresponding Ags of previous strains of subtype H1N1 to which most individuals had been exposed. Although recent infection with heterotypic viruses may have diminished the level of illness severity in adults,126 the population’s prior immunological experience with related internal Ags did not prevent the 1957 Asian H2N2 influenza A virus from causing a major pandemic.303 In contrast to this experience, observations following the re-emergence of the H1N1 virus in 1977 indicated that homotypic humoral immunity provided a high level of resistance that lasted for decades. Similarly, humans exposed to the 1918 influenza H1N1 virus as infants sustained circulating memory B cells encoding neutralizing Abs to the HA into the 10th decade of life,467 and these Abs cross-reacted with the 2009 H1N1 pandemic strain and provided protection.233,462 Induction of cross-reactive Abs to influenza may sometimes be harmful. For example, immune complexes with complement deposition have been demonstrated in lung tissues of victims of pandemic influenza.287 Additional evidence for the importance of responses to influenza A surface glycoproteins was provided by passive and active immunization studies in mice. Monoclonal antibodies (mAbs) directed against the HA or NA proteins protected mice against challenge with virulent wild-type virus, whereas mAbs specific for the internal nucleoprotein or matrix protein did not alter the course of disease.303 Immunization with recombinant vaccinia viruses expressing each of the 10 known influenza A gene products showed that only recombinants expressing the HA or the NA glycoprotein induced resistance to virus challenge.124 Whereas the vaccinia vector-induced CD8 T-cell–mediated immunity specific for internal viral proteins provided some measure of heterosubtypic cross-reactivity against influenza challenge,125 the aggregate data suggest that a successful strategy of immunoprophylaxis against most virus infections requires the generation of an immune response to the surface Ags displayed on virions and on virus-infected cells. Other viruses (such as poxviruses, herpesviruses, filoviruses, some respiratory viruses, and lentiviruses) that have a more complex pathogenesis may require vaccines that induce more complex immune responses for protection from infection or control of disease. Many of these viruses possess the capacity for persistence, infect immunoprivileged sites, use sophisticated immune evasion strategies, directly infect their target organ without a requirement for viremia, or cause disease by eliciting an aberrant host response. Vaccine development for these viruses probably will require the induction of neutralizing Abs against surface proteins to protect against infection in addition to T-cell responses against structural and regulatory proteins to control virus spread and to modulate the composition of the immune response.167
The surface glycoproteins of enveloped viruses and the capsid proteins of nonenveloped viruses are the primary target Ags for Ab-mediated protection. The following generalizations can be made about the nature of Abs and their interaction with virus surface proteins. First, antiviral Abs against the extracellular domain of surface proteins predominantly recognize conformational epitopes. Such conformational epitopes are difficult to mimic with peptides or proteins that have had the structure of antigenic sites modified by vaccine preparation and formulation. Presentation of the native Ag structure in its authentic conformation is critical for induction of neutralizing Abs. Even when the structure is mimicked with atomic-level precision and elicits Abs that bind the epitope, the property of viral neutralization may not be achieved.274,326 Most viral surface proteins assemble into oligomeric states on virions, typically dimers, trimers, or tetramers; however, the monomeric form of the protein is also present in cell debris or shed proteins. The principal neutralizing determinant may be present only in quaternary structures; thus, Abs binding to monomeric protein do not recognize or neutralize virions. In addition, many surface proteins that are targets of neutralizing Abs are fusion proteins present in a metastable state on virions. During the attachment and fusion process, the fusion proteins transition from prefusion to postfusion forms, in some cases drastically altering the structure of neutralizing epitopes. Second, Abs recognize many major antigenic sites on each viral protein, and within each of these antigenic sites, there may be multiple epitopes. For example, the parainfluenza virus attachment glycoprotein (HN) has at least six antigenic sites, three of which are recognized by neutralizing Abs.85 After infection, individuals often develop Abs only to a subset of the antigenic sites of the infecting virus.86 After one or more reinfections, the Ab response broadens to recognize more antigenic sites. These findings provide a partial explanation for the failure of immunization to induce complete immunity in certain circumstances and also provide a rationale for recommending multiple immunizations to achieve optimal protection. Third, the large size of immunoglobulin (Ig) molecules often precludes direct access to functional sites on viral surface proteins, such as the receptor binding site, sites with enzymatic activity, or fusion peptides. Such domains can be buried within the protein structure of the surface protein or masked by carbohydrate molecules, variable loops, or conformational flexibility.236 As a consequence, the critical sites vulnerable to Ab-mediated neutralization can remain relatively inaccessible. Therefore, Abs usually exert their antiviral activities by mechanisms other than direct inactivation of functional sites on the surface proteins. Instead, Abs most often act directly or indirectly to impede attachment of virus to the cell or to prevent uncoating of virus that has attached to or entered the cell. Unfortunately, effective Ab-mediated protection against virus infection is not always achievable. For example, the Ab response to the envelope glycoprotein of human immunodeficiency virus (HIV) evolves over time similar to the response elicited by repeated infections with parainfluenza virus; however, the elaboration of the envelope-specific Ab repertoire is not able to keep pace with the rapid genetic evolution of the virus. The Abs present at a given point in time may neutralize virus isolated from the subject 6 months earlier, although they have very low neutralization activity against current strains that have mutated extensively.368 Sufficiently high levels of passively administered broadly neutralizing mAbs can prevent simian-human immunodeficiency virus (SHIV) infection of macaques in some settings.265 SHIV is a chimeric lentivirus with the internal and regulatory proteins of simian immunodeficiency virus (SIV) and the surface
glycoprotein (gp160) of HIV-1. However, immunization with currently available HIV-1 envelope Ags does not elicit Abs with these neutralizing properties. There are also examples from Ebola virus,445 vaccinia virus,31,271 and lentivirus393 models that demonstrate settings in which both Ab and T-cell responses contribute to vaccine-induced protection. Ags for eliciting T-cell responses can be surface proteins, internal structural proteins, or regulatory proteins. For example, the major CD8 T-cell responses to HIV-1 are directed toward Gag, Pol, and Nef proteins.36 Often, the nucleocapsid and matrix proteins of viruses are found to encode important cytotoxic T lymphocyte (CTL) epitopes,428 perhaps because they are typically more abundant, more conserved, and produced earlier in the virus life cycle than surface proteins.
glycoprotein (gp160) of HIV-1. However, immunization with currently available HIV-1 envelope Ags does not elicit Abs with these neutralizing properties. There are also examples from Ebola virus,445 vaccinia virus,31,271 and lentivirus393 models that demonstrate settings in which both Ab and T-cell responses contribute to vaccine-induced protection. Ags for eliciting T-cell responses can be surface proteins, internal structural proteins, or regulatory proteins. For example, the major CD8 T-cell responses to HIV-1 are directed toward Gag, Pol, and Nef proteins.36 Often, the nucleocapsid and matrix proteins of viruses are found to encode important cytotoxic T lymphocyte (CTL) epitopes,428 perhaps because they are typically more abundant, more conserved, and produced earlier in the virus life cycle than surface proteins.
Vaccine-Induced Immunity
Immune mechanisms have evolved to protect the host against a diverse assortment of viral pathogens. Viruses and their hosts co-evolve; viruses find ways to evade host defenses, and hosts adapt mechanisms to control virus replication. The host is equipped with mechanical barriers and innate immune mechanisms that can limit the initial encounter with a virus and adaptive immune mechanisms that provide immunity against repeated exposures to the same viral pathogen. Although innate responses are important during the induction phase of vaccine-induced immunity, humoral and cellular adaptive responses are the primary effector mechanisms of protection from future infection (e-Fig. 14.4). Humoral and cellular responses are complementary in that Ab is able to prevent infection by free virus; however, when infection occurs despite innate defenses and the presence of neutralizing Abs, T-cell responses are designed to eliminate virus-infected cells and diminish the subsequent release of infectious virus. The repertoires of BCRs and TCRs have also evolved complementary strategies to deal with genetic variation in viruses. For a given antigenic site on a viral protein, most hosts can expand a B-cell population that can recognize the structure. This leads to population-wide protection against reinfection with a particular virus. In turn, this forces antigenic change in the virus, often resulting in a new serotype, which is not recognized by the original Ab. Therefore, the host has to start over in the generation of Ab-mediated type-specific protection. In contrast, T-cell epitopes are not shared between hosts unless they happen to share the same major histocompatibility complex (MHC)-restricting allele. Consequently, each infected host generates T-cell responses against a limited number of viral epitopes that are largely determined by human leukocyte antigen (HLA) haplotype. Unlike Ab epitopes, T-cell epitopes are often cross-reactive between viral serotypes and thereby provide the Ag-experienced host with partial protection against new virus strains until the Ab responses can adapt (e-Fig. 14.5).
When virus breaches the physical and innate barriers and infects a target cell, several rounds of replication may occur in a primary infection before the adaptive immune responses are established (e-Fig. 14.6A). Depending on the virus, this may result in spread to the target organ of disease and/or establishment of a persistent infection. The timing, magnitude, location, and qualitative features of the host response will determine the clinical outcome of infection. In persistent virus infections (e.g., HIV, hepatitis C virus [HCV], or herpes simplex virus [HSV]), the disease manifestations are often congruent with virus replication. In self-limited infections (e.g., influenza, respiratory syncytial virus [RSV], or dengue), the bulk of disease is often after the peak of viral replication, although in some cases disease can also be temporally associated with viral load (e-Fig. 14.6B). In cases of persistent or latent virus infection, the immune response attempts to limit virus replication or spread. Control of the magnitude and composition of complex immune responses is critical to avoid immunopathology. Fortunately, there is considerable redundancy inherent in immunity to viruses. For example, while CD8 T cells are the primary mediator of cytolytic activity, this function also can be accomplished by complement or by NK cells targeting the Fc portion of Ab labeling virus-infected cells. Another well-studied example of this redundancy involves CD4 and CD8 T cells, either of which are sufficient to achieve clearance of virus, as shown in studies of influenza A virus or poxviruses in mice. Animals lacking CD8 T cells readily clear influenza virus or vaccinia virus from the lungs, indicating that CD4 T cells and Ab are sometimes sufficient for viral clearance.271,390 The therapeutic efficacy of Abs acting in the absence of other immune functions has also been demonstrated in experimental influenza A virus infection of mice. Abs to the influenza A virus surface HA have cleared virus from the lungs of persistently infected severe combined immunodeficiency (SCID) mice.390 Whereas CD8 T cells are specialized in recognition and clearance of virus-infected cells, CD4 T cells (plus the Abs secreted by B cells for which they provide help) and CD8 T cells can mediate viral clearance independently. The relative importance of these two arms of the immune response varies in the resolution of particular virus infection. Even in the case of lymphocytic choriomeningitis virus (LCMV), the prototypic arenavirus, which in the murine model is a paradigm for CD8 T-cell–mediated immunity, Abs and CD4 T cells each make a significant contribution to the resolution of primary virus infection.21 In general, Abs are the major mediator of resistance to reinfection with virus, and CD8 T cells are the major mediator of clearing virus-infected cells. CD4 T cells are critical for the induction of robust Ab responses and important for “helping” CD8 T-cell responses; they also have potential for direct antiviral effector functions. Abs work best when the target organ for disease pathogenesis is distinct from the initial site of infection, particularly in cases where the virus has to travel through the bloodstream to reach its target organ. For example, in diseases such as measles, polio, hepatitis, or the viral encephalitides, where viremia must be established to cause disease, it often takes very small concentrations of pre-existing Abs to achieve clinical protection. Because it is rarely possible to elicit sufficient Ab through vaccination to prevent virus infection completely, other immune mechanisms must contribute to vaccine-induced immunity.
One reason why live virus vaccines are so effective is that many components of both innate and adaptive immunity are engaged. The protein, nucleic acid, carbohydrate, and lipid components of viruses induce innate responses through a variety of toll-like receptor (TLR) and other pathogen-associated molecular pattern (PAMP) receptor interactions. Virus infection induces all components of the adaptive immune response—Ab responses are induced against viral surface proteins in their native conformation, CD4 T cells are stimulated,
and importantly, viral proteins are produced in the cytoplasm, processed, and presented in MHC class I molecules for induction of CD8 CTL responses. All of these responses are needed to achieve the optimal clinical outcome from a virus infection. Abs and innate defenses provide the initial barrier to infection by neutralizing incoming virus. After the virus has infected a cell, however, it is then incumbent on the innate defenses to control the virus until the adaptive cellular immune response can be activated, expand, and traffic to the affected tissues. In the immune host, the pre-existing Ab will prevent or limit the number of infected cells, and rapid mobilization of memory T-cell responses will rapidly clear virus-infected cells (e-Fig. 14.7A). Although cytolytic T cells are potent in their ability to clear virus-infected cells and reduce the spread of virus, it comes at a cost. The T-cell response can injure the host as it clears the virus and is thus tightly regulated. Turning the immune response off is just as important as turning it on for the well-being of the host. The system is biased toward controlling inflammation, and that may be a reason why the T-cell response is regulated to require several days to activate and expand. Influencing the events in this period between virus exposure and the fully activated primary T-cell response is one of the key factors in determining the efficacy of vaccine-induced immunity, for which the goal is to prevent, abort, or control virus infection to avoid clinical disease (e-Fig. 14.7B).
and importantly, viral proteins are produced in the cytoplasm, processed, and presented in MHC class I molecules for induction of CD8 CTL responses. All of these responses are needed to achieve the optimal clinical outcome from a virus infection. Abs and innate defenses provide the initial barrier to infection by neutralizing incoming virus. After the virus has infected a cell, however, it is then incumbent on the innate defenses to control the virus until the adaptive cellular immune response can be activated, expand, and traffic to the affected tissues. In the immune host, the pre-existing Ab will prevent or limit the number of infected cells, and rapid mobilization of memory T-cell responses will rapidly clear virus-infected cells (e-Fig. 14.7A). Although cytolytic T cells are potent in their ability to clear virus-infected cells and reduce the spread of virus, it comes at a cost. The T-cell response can injure the host as it clears the virus and is thus tightly regulated. Turning the immune response off is just as important as turning it on for the well-being of the host. The system is biased toward controlling inflammation, and that may be a reason why the T-cell response is regulated to require several days to activate and expand. Influencing the events in this period between virus exposure and the fully activated primary T-cell response is one of the key factors in determining the efficacy of vaccine-induced immunity, for which the goal is to prevent, abort, or control virus infection to avoid clinical disease (e-Fig. 14.7B).
Vaccine-Induced Cellular Immunity
MHC class I–restricted CD8 cytotoxic CTLs and MHC class II–restricted CD4 helper T cells (Th cells) each function independently as Ag-specific antiviral effector cells (Chapter 9). Intact cellular immunity is critical for protection against some types of viruses. For example, most herpesvirus infections become more severe and destructive when cellular immunity is compromised. Vaccinia can cause progressive tissue invasion in persons with cell-mediated immune deficiencies. RSV and parainfluenza type 3 often become lethal infections in the setting of SCID or in patients who have undergone bone marrow transplantation. In contrast, patients with Ab deficiencies experience more severe disease only from selected viruses, particularly picornaviruses.
CD8 T Lymphocytes
CD8 T cells are the major T-cell effectors of antiviral activity. They are often referred to as CTLs, although they can clear virus-infected cells by both lytic and noncytolytic mechanisms. The CD8 TCR recognizes a short peptide derived from an endogenously produced viral protein in the context of the MHC class I β2-microglobulin heterodimer expressed on the surface of an infected cell. Ag presentation by the MHC class I β2-microglobulin heterodimer is restricted to viral peptides that are produced and processed during infection. Therefore, the major CTL functions are to eliminate infected cells134 or to inhibit virus replication by the elaboration of soluble mediators such as cytokines138,299,425 or chemokines.77,464 The net effect of CTL activity is to prevent further spread of virus and to terminate infection in cells that are already infected. The importance of CTLs in recovery from virus infection is indicated not only by the association of cellular immune deficiencies with severe virus infection but also by the diverse strategies that viruses use to escape from CTL immunity.408 Viruses such as herpesviruses, poxviruses, and lentiviruses in particular have evolved strategies for interfering with Ag presentation pathways or CD8 T-cell effector molecules that compromise CTL activity. Lentiviruses are prototypes for escaping CTL killing by genetic variation and selecting mutations of key epitopes. CD8 T-cell activity is critical for controlling SIV replication.393 When that control is mediated by a dominant CTL response to a single epitope, immune control of viremia is lost when that epitope is altered by mutation.24
Although CTL antiviral activity generally is associated with the clearance of virus and the reduction of virus-associated pathology, disease enhancement can be observed under experimental conditions in which CTLs have been transferred passively to virus-infected recipients.58,121,293 Therefore, one can view the immunopathology caused by the T-cell response to virus infection as the cost for virus clearance. A major goal of vaccination is to induce CD8 T cells with properties to clear virus-infected cells efficiently and rapidly to minimize the immunopathology associated with virus infections.
The time course of CTL activation during virus infection in the lungs is consistent with its important role in clearance of primary virus infection. Primary CD8 CTL activity in lung peaks at about day 7 during acute virus infection, such as that produced by influenza A virus, LCMV, or RSV.14,134,209 The naive CD8 T cell in the LCMV model undergoes a process of activation and expansion during that time of 10,000- to 50,000-fold.40 After about 40 days, the level of detectable virus-specific memory CD8 CTL is about 10% of that during acute infection and is then maintained at constant levels for years even in the absence of Ag.209 Each successive virus infection adds an expanded set of specificities to the pool of memory CD8 T cells over time and shapes the capacity of the host to respond to selected viruses.398,399 The memory CD8 T-cell pool not only has an increased precursor frequency against virus-specific epitopes; those cells also have a faster response time. The influence of CD8 CTL memory on the outcome of a subsequent virus exposure can be subtle and may depend on the absence or presence of other components of the immune response. For viruses in which a significant component of the clinical syndrome is related to the immune response clearing the virus, having memory CD8 T-cell responses will result in reduced virus replication a day or two earlier than in primary infection, an earlier peak illness time point, and a lower peak magnitude of illness.138,168 In a setting where there is a large viral load (e.g., high inoculum challenge) and an absence of pre-existing neutralizing Abs to diminish the number of virus-infected cells, a large CTL response or infusion of excessive CD8 T cells can result in more severe disease.59
To date, T-cell response has not been used successfully as a correlate of immunity that is the basis for licensure of a vaccine. However, the incorporation of CTL epitopes into viral vaccines has been considered valuable because of the important role that CTLs play in clearance of established virus infections. The following characteristics of the CTL response in vivo should be kept in mind when designing a vaccine for viruses that may require a CTL component of immunity. First, the ability of a CTL epitope to induce an immune response is MHC dependent. Therefore, the incorporation of large antigenic content is important so that multiple CD8 T-cell specificities can be elicited by the vaccine to allow selection of epitopes by the diverse repertoire of human MHC class I alleles and to reduce the possibility of immune escape through genetic mutation. Second,
when considering vaccine-induced immune protection, CTLs represent a second line of defense against virus infection because they cannot prevent infection and are only effective against infected cells. The localization and phenotype of CD8 T cells can influence the timing and efficiency of viral clearance. For example, immunization of macaques with a recombinant CMV vector that induces a sufficiently high frequency of intraepithelial effector memory CD8 T cells can rapidly clear virus-infected cells following SIV challenge, leading to abortive infection.185 Technologies such as polychromatic flow cytometry,348 MHC class I tetramer reagents that provide the means to sort epitope-specific T cells,108 the ability to define functional subsets of T cells with more precision,105 sequencing technology that allows single-cell clonotyping of epitope-specific cells, and correlation of the TCR-peptide-MHC biophysical interaction and structure with T-cell functions37 may provide the necessary information to harness CD8 T cells more effectively in the future.
when considering vaccine-induced immune protection, CTLs represent a second line of defense against virus infection because they cannot prevent infection and are only effective against infected cells. The localization and phenotype of CD8 T cells can influence the timing and efficiency of viral clearance. For example, immunization of macaques with a recombinant CMV vector that induces a sufficiently high frequency of intraepithelial effector memory CD8 T cells can rapidly clear virus-infected cells following SIV challenge, leading to abortive infection.185 Technologies such as polychromatic flow cytometry,348 MHC class I tetramer reagents that provide the means to sort epitope-specific T cells,108 the ability to define functional subsets of T cells with more precision,105 sequencing technology that allows single-cell clonotyping of epitope-specific cells, and correlation of the TCR-peptide-MHC biophysical interaction and structure with T-cell functions37 may provide the necessary information to harness CD8 T cells more effectively in the future.
CD4 T Cells
CD4 T cells provide a variety of functions, including help to B cells and CD8 T cells, thereby augmenting the two major effector mechanisms of the adaptive immune response. CD4 T cells can also mediate direct antiviral activity in vivo188,262,296,426; however, this is not their major function as it is for CD8 T cells. The MHC class II, αβ heterodimer—the restricting element for CD4 T cells—is present predominantly on antigen-presenting cells (APCs) such as dendritic cells, macrophages, monocytes, and B cells. However, during inflammation, even epithelial cells can express MHC class II molecules, and CD4 T cells have been shown to have compensatory viral clearance capacity in the absence of CD8 T cells. In addition to having antiviral and helper activity, CD4 T cells can mediate immunopathology12,279,422 and have been shown to have regulatory and other specialized functions476 (e-Fig. 14.8).
The CD4 T-cell response to certain internal viral proteins may play a cooperative role in the development of effective resistance by augmenting the Ab response to a major surface protein.380 For example, immunization with the membrane (M) protein of influenza A virus can prime for a subsequent augmented Ab response to the HA glycoprotein on virus particles.380 In this manner, the entire CD4 T-cell repertoire developed against the viral surface and internal proteins can be called into play to amplify a B-cell response to a surface protein. Because MHC class II–restricted T-cell epitopes tend to be more numerous on viral proteins than MHC class I–restricted epitopes,234 the repertoire of CD4 T cells capable of augmenting the Ab response is large. Although CD4 T cells are typically thought to provide indirect helper functions to improve CD8 T-cell and Ab responses, there is evidence that they may also contribute direct effector functions to virus clearance. CD4 T cells have been shown to have cytolytic function against HIV.329 Viral Ags can induce a protective immune response mediated by CD4 T cells in the absence of B cells or CTLs,262 indicating that this arm of the immune response can make an independent contribution to resistance against both acute and chronic virus infections.188 CD4 T cells also secrete IFN-γ, tumor necrosis factor (TNF)-α, and other soluble factors that may directly inhibit virus replication.322,323 For these reasons, it is advisable to include virus Ags capable of inducing CD4 T-cell responses in viral vaccines to achieve maximal immunogenicity. The processes involved in CD4 T-cell memory induction are distinct from the processes involved in establishing CD8 T-cell memory responses397 and more complex in some ways. Ag processing and presentation in MHC class II molecules can occur through the endocytic pathway; therefore, unlike CD8 T cells, CD4 T cells can be induced by killed virus vaccines or even purified proteins and do not require live virus or gene delivery approaches to allow Ag to reach the cytoplasm. CD4 T cells do not proliferate to the same extent as CD8 T cells during primary infection, and those producing IFN-γ do not survive as long-lived memory cells.472
CD4 T cells have traditionally been considered “helper” T cells (Th), and indeed their activities can improve the potency of B-cell and CD8 T-cell responses. In addition to the original subsets Th1 and Th2, there are now many subpopulations of T cells defined with distinct functions, some of which can alter or diminish effector function. For example, CD4+FoxP3+ T cells have been found to have inhibitory effects on other immune effector functions, hence the name T-regulatory cells, or Tregs.30 The extent to which vaccines induce CD4+FoxP3+ T cells with T-regulatory activity is not well defined. In addition, there are other subsets of CD4 T cells that produce interleukin (IL)-17 and other proinflammatory cytokines that have been associated with immune-mediated pathology but are also important for immunity against some pathogens. More recently, a CD4+CXCR5+ subset of CD4 T cells designated T-follicular helper (Tfh) cells has been identified that resides primarily in lymph node germinal centers and promotes B-cell memory and plasma cell differentiation.95 The role of each CD4 T-cell subset in vaccine-induced protection and optimal balance is an area that needs more investigation.
Vaccine-Induced Humoral Immunity
The correlates of immunity that have been established to date for licensed viral vaccines have been associated with Ab responses. In fact, the rationale for development of many of the vaccines in use today was the evidence derived from clinical trials of Ab treatments showing that Abs could mediate protection against disease. Passive transfer studies established the efficacy of Abs in preventing or treating virus infections and disease caused by a wide variety of viruses belonging to diverse RNA or DNA virus families that include the orthomyxoviruses, paramyxoviruses, alphaviruses, flaviviruses, arenaviruses, lentiviruses, picornaviruses, hepadnaviruses, and herpesviruses. Examples of the licensed clinical use of Abs include preparations for hepatitis A and B viruses, measles virus, poliovirus, VZV, rabies virus, RSV, and cytomegalovirus (CMV). A large number of new human mAbs have been discovered in the past decade, and some are under development as prophylactic agents.
A long history of Ab treatments laid the groundwork for current vaccine strategies. Commercial human gamma globulin, which usually contains 16% to 18% immunoglobulin G (IgG) is highly effective in preventing hepatitis A disease and was used widely for that purpose during the past 50 years, even though only a small proportion of the Abs in the preparations are specific for that virus.89 Currently, inactivated or recombinant protein vaccines for hepatitis A are licensed and thought to work by inducing serum Abs to the virus. A historic double-blind, prospective clinical trial performed in 1951–1952 showed that human IgG also was effective in preventing
paralytic disease caused by poliovirus.181 Intramuscular inoculation of 0.3 mL of IgG/kg conferred significant resistance that lasted for at least 5 weeks. This seminal observation showed that Abs alone can confer resistance to poliomyelitis and suggested that vaccines inducing such Abs might protect against the disease. Subsequent field trials of inactivated vaccines by Salk and live attenuated vaccines by Sabin validated this view, leading to implementation of universal immunization against poliovirus and ultimately the current worldwide campaign for elimination of polio disease (Fig. 14.1). Before the live measles virus vaccine was licensed for routine use in immunization of young children, human IgG was used for passive prophylaxis and was the mainstay for prevention of disease. IgG given at a dose of 0.5 mL/kg prevented measles, even when administered to exposed individuals as late as 3 days after inoculation with virus.202 Human IgG prepared from pooled plasma selected for a high titer of varicella-zoster virus (VZV) Abs (varicella-zoster immune globulin [VZIG]) is licensed in the United States for use in prevention of severe varicella in immunosuppressed children.471 Most of the exposed immunosuppressed children receiving treatment still become infected; however, most exhibit attenuated disease or subclinical infection. Live attenuated varicella virus vaccine was licensed and recommended for universal immunization in 1995. Human IgG was effective in preventing chronic hepatitis B virus (HBV) infection in high-risk infants following maternal exposure.28 Hepatitis B immune globulin and subunit vaccine are now used in concert to protect infants in this setting.
paralytic disease caused by poliovirus.181 Intramuscular inoculation of 0.3 mL of IgG/kg conferred significant resistance that lasted for at least 5 weeks. This seminal observation showed that Abs alone can confer resistance to poliomyelitis and suggested that vaccines inducing such Abs might protect against the disease. Subsequent field trials of inactivated vaccines by Salk and live attenuated vaccines by Sabin validated this view, leading to implementation of universal immunization against poliovirus and ultimately the current worldwide campaign for elimination of polio disease (Fig. 14.1). Before the live measles virus vaccine was licensed for routine use in immunization of young children, human IgG was used for passive prophylaxis and was the mainstay for prevention of disease. IgG given at a dose of 0.5 mL/kg prevented measles, even when administered to exposed individuals as late as 3 days after inoculation with virus.202 Human IgG prepared from pooled plasma selected for a high titer of varicella-zoster virus (VZV) Abs (varicella-zoster immune globulin [VZIG]) is licensed in the United States for use in prevention of severe varicella in immunosuppressed children.471 Most of the exposed immunosuppressed children receiving treatment still become infected; however, most exhibit attenuated disease or subclinical infection. Live attenuated varicella virus vaccine was licensed and recommended for universal immunization in 1995. Human IgG was effective in preventing chronic hepatitis B virus (HBV) infection in high-risk infants following maternal exposure.28 Hepatitis B immune globulin and subunit vaccine are now used in concert to protect infants in this setting.
Preclinical studies in animals suggested that serum RSV-neutralizing Abs above a threshold titer were protective against lower respiratory tract infection, and this observation was validated during a clinical trial of human IgG containing a high titer of RSV-neutralizing Abs that led to licensure in 1995.1,359 Of interest, the level of serum RSV-neutralizing Abs required for reduction of hospitalization was similar to that needed to prevent infection in cotton rats. A second-generation approach—the use of a humanized murine-neutralizing mAb to RSV—was licensed in 1998.2 To date, this is the only mAb licensed for prevention of an infectious disease. These clinical applications strongly suggest that neutralizing Abs are an important correlate of protection for RSV vaccines, which are under development. Immunosuppressed patients who have not been infected previously with CMV, a herpesvirus, are at high risk of developing severe life-threatening disease following exposure to the virus, especially when they receive an organ transplant from a CMV-infected donor. Administration of CMV IgG, prepared from pooled units of plasma selected for a high Ab titer, prevents approximately half of the serious illnesses attributable to CMV but does not prevent infection.405 This finding is interesting because the mode of action suggests that Abs suppress disease by their action on CMV-infected cells and that Abs with these properties should be a target for CMV vaccines under development.
Mechanisms of Virus Neutralization
Abs may render free virus noninfectious, principally through neutralization of infectivity. Virus-neutralizing activity typically is measured by in vitro assays in which Ab and infectious virus are incubated and then inoculated onto cell culture. Residual infectivity is quantified by plaque count, infectious foci, signal from recombinant reporter virus, percent of infected cells detected by flow cytometry or other methods and compared to untreated suspensions. Viruses are neutralized in vitro by Abs by a wide variety of mechanisms. Important Ab
characteristics include affinity/avidity, isotype, concentration, Ab-to-virus ratio, valency, state of polymerization, ability to bind the polyimmunoglobulin receptor (pIgR), ability to fix complement, and specificity for the virus protein and epitope of interest. Virus-related factors important to neutralization include general aspects of the replication cycle of the virus under consideration (receptor use, mode of entry into the cell, pH dependence of fusion, site of replication) and individual characteristics of the specific virus strain (antigenic subgroup and amino acid sequence of viral surface proteins). Host factors influencing the mechanism of neutralization include the type and origin of cells and the level of expression of membrane receptors. The most potent mechanisms of neutralization act prior to infection of cells. First, free infectious virions in solution can be aggregated by multivalent Abs directed against surface glycoproteins.17,331 The consolidation of multiple infectious virions into an aggregate reduces the number of available infectious units. Second, Abs can inhibit virus attachment to cells, usually by binding directly to virus attachment proteins. Abs to attachment proteins often are poorly neutralizing in vitro, effecting only partial neutralization even at high concentration.211 This effect may be caused by virus heterogeneity owing to a variable extent of glycosylation that occurs during posttranslational processing at the numerous glycosylation sites on these proteins, or because of multiple routes of entry or mechanisms of attachment. Third, viruses can be neutralized following attachment, at the cell surface prior to cell entry, suggesting that immune serum contains Abs capable of inhibiting entry into the cell. For example, Abs to human papillomavirus (HPV) can interrupt infection at two stages of attachment and entry: the first an initial association with the acellular basement membrane that induces conformational changes in the virion and second the association of virus with the keratinocyte cell surface.104 Some neutralizing Abs decrease infectivity by inhibition of virus–cell fusion at the plasma membrane. Fusion inhibition (FI) activity for some Abs has been inferred from in vitro assays in which Ab is incorporated into overlay medium after virus absorption and penetration, and activity measured by inhibition of postinfection cell-to-cell fusion. Additionally, Abs can inhibit the release of progeny viruses from infected cells. There is even evidence that some influenza virus–specific Abs inhibit virus replication by preventing the primary uncoating of virus that is associated with low-pH membrane fusion in the endosome or at the step of the secondary uncoating of the virion core structure—a step required for primary transcription of the virus genome.330,332 Our current understanding of the induction of such Ab functions is not sufficient to allow us to design specific vaccine strategies to induce them.
characteristics include affinity/avidity, isotype, concentration, Ab-to-virus ratio, valency, state of polymerization, ability to bind the polyimmunoglobulin receptor (pIgR), ability to fix complement, and specificity for the virus protein and epitope of interest. Virus-related factors important to neutralization include general aspects of the replication cycle of the virus under consideration (receptor use, mode of entry into the cell, pH dependence of fusion, site of replication) and individual characteristics of the specific virus strain (antigenic subgroup and amino acid sequence of viral surface proteins). Host factors influencing the mechanism of neutralization include the type and origin of cells and the level of expression of membrane receptors. The most potent mechanisms of neutralization act prior to infection of cells. First, free infectious virions in solution can be aggregated by multivalent Abs directed against surface glycoproteins.17,331 The consolidation of multiple infectious virions into an aggregate reduces the number of available infectious units. Second, Abs can inhibit virus attachment to cells, usually by binding directly to virus attachment proteins. Abs to attachment proteins often are poorly neutralizing in vitro, effecting only partial neutralization even at high concentration.211 This effect may be caused by virus heterogeneity owing to a variable extent of glycosylation that occurs during posttranslational processing at the numerous glycosylation sites on these proteins, or because of multiple routes of entry or mechanisms of attachment. Third, viruses can be neutralized following attachment, at the cell surface prior to cell entry, suggesting that immune serum contains Abs capable of inhibiting entry into the cell. For example, Abs to human papillomavirus (HPV) can interrupt infection at two stages of attachment and entry: the first an initial association with the acellular basement membrane that induces conformational changes in the virion and second the association of virus with the keratinocyte cell surface.104 Some neutralizing Abs decrease infectivity by inhibition of virus–cell fusion at the plasma membrane. Fusion inhibition (FI) activity for some Abs has been inferred from in vitro assays in which Ab is incorporated into overlay medium after virus absorption and penetration, and activity measured by inhibition of postinfection cell-to-cell fusion. Additionally, Abs can inhibit the release of progeny viruses from infected cells. There is even evidence that some influenza virus–specific Abs inhibit virus replication by preventing the primary uncoating of virus that is associated with low-pH membrane fusion in the endosome or at the step of the secondary uncoating of the virion core structure—a step required for primary transcription of the virus genome.330,332 Our current understanding of the induction of such Ab functions is not sufficient to allow us to design specific vaccine strategies to induce them.
Structural and Biochemical Features of Antibodies Important to Affinity
Abs induced by primary infection or immunization are generally of low affinity for the target Ag. Affinity maturation of Abs is mediated by somatic mutation of the genes encoding B-cell receptors, which occurs in germinal centers, most prominently following second infections or booster doses of vaccine. Somatic hypermutation (SHM) coupled with B-cell selection results in the promulgation of high-affinity Abs in the antiviral repertoire. Increases in affinity likely increase neutralizing potency for most antiviral Abs directed to surface proteins. Although the molecular basis for affinity maturation during secondary responses was not understood during the development of early vaccines, the enhanced efficacy of multiple-dose vaccine regimens in clinical trials pointed the way to a practical use of this principle. Most licensed viral vaccines are administered using multiple-dose regimens to enhance the quality, magnitude, and durability of the immune response.
Increased affinity is widely believed to confer enhanced functional competence on Abs. Work using HIV-1–specific Abs derived from combinatorial phage display Ab libraries demonstrated improvements in neutralization associated with affinity maturation caused by SHM.427 The fusion (F) protein—the major protective Ag of RSV—has at least four antigenic sites, each with a differing capacity as a target for virus neutralization.29 Recombinant derivatives of a humanized RSV mAb containing random mutations in the combining site have shown that mutations enhancing the association rate improved neutralization and efficacy, whereas mutations improving the dissociation rate did not, despite their contribution to improved steady-state affinity.458 Somatic mutations in human rotavirus Abs have been shown to enhance affinity and inhibitory function, with individual mutations in Ab combining sites conferring several hundred-fold increases in affinity and function.211 The broadest and most potent human Abs to HIV and influenza virus isolated to date are distinguished by exceptional numbers of somatic mutations in the Ab sequences (on the order of about one-third of nucleotides altered).467,474 Hypermutation introduces not only point mutations that enhance function, but in some cases nontemplated insertions of sequences encoding new amino acids that mediate neutralization.233 In the vesicular stomatitis virus (VSV) mouse model, investigators have shown only modest increases in the affinities of hypermutated Abs compared with those in germline configuration over the course of infection and after repeated booster injections.210 The VSV G glycoprotein is unusual in that it contains only a single immunodominant antigenic site that reliably induces neutralizing Abs. Neutralization activity also is determined by the number of times the neutralizing determinant is displayed on the surface of the virion, and some studies suggest that virion particles may be dynamic in their display of epitopes.112 Understanding the relationship of affinity and neutralization is needed so that we can better appreciate the B-cell response to exposure with foreign Ags in a physiologic context. Furthermore, elucidation of the role of affinity in antiviral Ab function is critical for designing new active and passive immunization strategies against pathogenic human viruses. The absence of somatic mutations in the virus-specific Ab sequences of infants probably accounts for the difficulty in inducing high-affinity, high-potency Abs in this population after a single vaccination.448
CDR3 Loops of the Antibody Variable Region in Antigen Binding
A combining site formed by the variable heavy (VH) and variable light (VL) domains of the Ab fragment (Fab) mediates Ag recognition. Both the VH and the VL chains have three noncontiguous linear sequences of greatest variability—CDRs—that result in flexible loops in the Ig protein and form the classical Ag binding site. HCDR3 shows the greatest variability in terms of length and sequence, particularly in human Abs. Several crystal structures of Abs have demonstrated that human CDRs tend to adopt a limited number of canonical conformations, with the exception of HCDR3.75 In each of the crystal
structures of human Abs complexed with Ags, HCDR3 has been shown to make important contacts in every case.23,401 Diverse CDR length and gene family usage have been shown to be essential features of immunological health and functional antiviral immune responses.153,228,389 Vaccinations that induce a broad Ab repertoire that is rich in CDR diversity are more likely to succeed than highly focused vaccinations inducing limited Ab repertoires. Although not universal, long HCDR3 regions are common in highly active Abs to HIV, and they may facilitate high-affinity binding to complex viral antigenic sites. For example, the crystal structure of the 17b mAb complexed with CD4-triggered HIV gp120 reveals a complex interaction that is accomplished only by the unique long HCDR3 region of this Ab.235 High-throughput screening for broadly reactive high-potency HIV-neutralizing Abs has isolated two unusual Abs with the longest CDRs ever identified,440 and the HCDR3s of these Abs possess fascinating secondary structural elements.346 In contrast, identification of another broadly neutralizing mAb specific for the CD4 binding site has a CDR3 loop of only 13 amino acids, although the variable region of the heavy chains is more than 30% somatically mutated from germ line.459
structures of human Abs complexed with Ags, HCDR3 has been shown to make important contacts in every case.23,401 Diverse CDR length and gene family usage have been shown to be essential features of immunological health and functional antiviral immune responses.153,228,389 Vaccinations that induce a broad Ab repertoire that is rich in CDR diversity are more likely to succeed than highly focused vaccinations inducing limited Ab repertoires. Although not universal, long HCDR3 regions are common in highly active Abs to HIV, and they may facilitate high-affinity binding to complex viral antigenic sites. For example, the crystal structure of the 17b mAb complexed with CD4-triggered HIV gp120 reveals a complex interaction that is accomplished only by the unique long HCDR3 region of this Ab.235 High-throughput screening for broadly reactive high-potency HIV-neutralizing Abs has isolated two unusual Abs with the longest CDRs ever identified,440 and the HCDR3s of these Abs possess fascinating secondary structural elements.346 In contrast, identification of another broadly neutralizing mAb specific for the CD4 binding site has a CDR3 loop of only 13 amino acids, although the variable region of the heavy chains is more than 30% somatically mutated from germ line.459
The Role of Isotype in Additional Mechanisms of Antibody-Mediated Virus Neutralization
The complement system is activated by the classical (Ab-dependent), alternative (Ab-independent), and lectin-binding pathways. Viral neutralization by specific Abs is concentration dependent and is often enhanced by complement activation. It has been reported that even apparently “nonneutralizing” Abs can acquire neutralizing activity with the addition of complement—for example, a nonneutralizing RSV murine mAb was shown to exhibit complement-enhanced neutralization in vivo in the presence of complement.92 Complement fixation is mediated by the CH2 region of the Ab Fc domain of immunoglobulin M (IgM), IgG1, and IgG3 subclasses in humans and the IgM, IgG2a, and IgG2b subclasses in mice.328 Understanding the role of complement in enhancing the functional capacity of Abs to neutralize viruses has broad implications for vaccine design, because the cytokine milieu induced by infection or immunization affects the isotype and subclass of the Ab response to the viral Ags by activating selective promoters in the appropriate Ig heavy chain locus. B cells triggered in an environment with high IFN-γ concentrations tend to produce IgG1 and IgG3 Abs. In contrast, in the presence of a dominant IL4 response, B cells produce immunoglobulin E (IgE) and IgG4 Abs. IgE participates in allergic diseases, and it is generally considered desirable to avoid strong IgE responses following vaccination. The Fc region of Abs also mediates some cellular activities, termed Ab-dependent cell-mediated cytotoxicity (ADCC), which are discussed below.
Structural Features of Antigens Critical for Inducing Efficient Antibody Interactions
The conformation of epitopes on viral surface proteins often is critical to the ability of vaccine Ags to induce or bind neutralizing Abs. Most neutralizing Abs bind to conformationally dependent epitopes on surface proteins. Linear peptide vaccine candidates derived from the sequence of the natural Ag rarely recapitulate the conformation of the protective Ag, which is why most peptide-based vaccines are poor immunogens for humoral immunity. Many subunit Ags comprising purified or recombinant viral proteins that have been developed as vaccine candidates contain the full polypeptide sequence of the Ag; however, the protein emerges from the purification process in a conformationally relaxed state that resembles the natural Ag only in part. Such Ags may induce high levels of Abs that bind the vaccine Ag but low levels of Abs that bind or neutralize virus.385 Some viruses appear to present highly antigenic surface loops to the immune system that are structurally nonessential, causing immune focusing on hypervariable domains of viral proteins, such as the influenza HA variable loops surrounding the sialic acid receptor–binding domain or the third variable (V3) region of HIV gp120. Recent studies, however, reveal that some subjects make broadly neutralizing Abs that recognize these hypervariable domains.156 Many structurally critical domains of viral surface proteins are hidden from B cells because they are buried in the interior of viral molecules or oligomers of molecules, or they are exposed only briefly during rapid conformational changes required for virus attachment or virus–cell membrane fusion. For example, viral hydrophobic fusion peptides, receptor-binding domains, heptad repeats, and other critical regions of fusion proteins usually are not accessible to Abs. Most fusion proteins require cleavage by proteases before they are competent to fuse, and Abs to the precursor protein may not bind the cleaved protein in the mature virus particle.336 Posttranslational modifications of surface proteins, especially glycosylation, can present a challenge for Ab recognition of protein because of the highly varied nature of the glycoprotein population. Deglycosylated protein Ags may enhance induction of protein-specific Ags; however, these Abs may not efficiently recognize glycosylated proteins in vivo. Some virus-neutralizing Abs are directed specifically to carbohydrates on virus glycoproteins.56 Some broadly neutralizing Abs to HIV, such as PG9 and PG16, recognize a conformational epitope that depends on glycosylation at specific variable loop N-linked sites.111 Posttranslational modification of Abs also can affect the interaction of Ab and virus. For instance, several human Abs to HIV gp120 are sulfated in the combining site, which allows the Ag-combining sites to mimic the structure of the virus coreceptor CCR5, which is sulfated.74 These Abs inhibit infectivity by binding to the co-receptor-binding domain on HIV gp120.
Antibody Escape Mechanisms
Viruses, especially RNA viruses with error-prone RNA-dependent RNA polymerases, exhibit facility in generating genetic diversity during multiple replicative cycles. During chronic infections, such as those with HBV, HCV, or retroviruses such as HIV, viruses that accumulate amino acid changes in epitopes that are targets of Abs owing to point missense mutations in the corresponding genes can escape neutralization by reduction or loss of binding. Some viruses easily shed surface glycoproteins (e.g., HIV gp120 or Ebola GP)187 or encode soluble forms of surface glycoproteins using alternative start codons (e.g., RSV G protein),369 in part as a decoy mechanism, targeting Abs away from infectious virions. The immunity induced by experimental vaccine candidates that are narrowly targeted to single epitopes or single proteins are susceptible to rapid viral escape. To avoid such Ab-resistant mutants, optimal vaccines should induce broad humoral responses to a diversity of antigenic sites.
Antibodies Active Against Virus-Infected Cells
Viruses that mature at the cell surface by budding or that insert viral glycoprotein(s) into the cell surface membrane render the infected cell susceptible to lysis by Ab-dependent complement-mediated lysis or ADCC mechanisms. This lysis is mediated by cytolytic cells, including NK cells, neutrophils, and eosinophils, in an Fc region–dependent manner. Ab-dependent cell-mediated virus inhibition (ADCVI) activity is similar but is typically measured with a readout of reduction of virus replication instead of lysis of target cells. Cytophilic Abs can arm leukocytes in the ADCC reaction, which has been shown to be important in the resistance of infant mice to herpes infection and for clearance of a retrovirus.87,226 Fc receptor–mediated mechanisms appear important to neutralization of HIV.191 Some herpesviruses encode an IgG Fc receptor homolog, which enables the virus to evade ADCC by binding Abs, especially virus-specific Abs if they bind virus proteins on infected cells, suggesting the importance of the ADCC effector or other Fc-mediated mechanisms in vivo.115 RSV ADCC activity mediated by NK cells has been detected in monkey or human peripheral blood using Abs in serum.216,277,395 Because infection of cells must occur for these immune mechanisms to act, these mechanisms represent a second line of defense against infection or disease.
Inadvertent Induction of Antibodies to Host Antigens
Immunization of monkeys with formalin-inactivated SIV produced in human cells induced Abs directed at Ags originating from the cells used to produce the virus.407 These anticellular Abs can neutralize the infectivity of SIV produced in human cells, although not virus produced in monkey cells, by interacting with human Ags incorporated into the virion outer membrane. This form of neutralization, which is mediated by complement-mediated virolysis, can yield an overestimate of vaccine immunogenicity. Hence, an effort should be made to avoid immunization with virions into which foreign host cell Ags have become incorporated.407 In some cases, even the native virion proteins may mimic structures associated with host cell proteins. For example, many of the original broadly neutralizing mAbs specific for the HIV-1 gp160 envelope glycoprotein have been shown to cross-react with host cell proteins.190
Inhibitory Antibodies that Lack Classical In Vitro Neutralizing Activity
Some Abs that do not exhibit neutralizing activity in vitro can mediate resistance to virus infections, although typically they are less effective than neutralizing Abs.297 Such Abs can be directed against Ags on the surface of virus particles or against virion or nonstructural proteins displayed on the surface of infected cells. In vivo, these Abs mediate antiviral activities by several different mechanisms. First, virus particles coated with nonneutralizing Abs can undergo accelerated clearance from the bloodstream. Second, Abs directed at surface glycoproteins such as the NA of influenza A virus or the HA-esterase protein of coronavirus (CoV) can decrease the level of virus replication in the target organ, presumably by inhibiting the release of virus from infected cells.46,466 Third, Abs can lyse virus-infected cells via IgG Fc-dependent mechanisms.92,391
Antiviral Activity of Antibodies In Vivo
Resolution of infection generally depends on CTLs, which reduce infection by lysing virus-infected cells. However, virus-specific Abs also can exert a therapeutic effect. Dramatic clinical therapeutic effect of viral Abs has been observed in patients with Argentine hemorrhagic fever, which is caused by Junin virus (an arenavirus). This disease has a high mortality; however, death can be prevented with a preparation of pooled human serum with a high titer of Junin virus–neutralizing Abs administered within 8 days of onset of symptoms.123,261 Mucosal virus infections that are limited to the cells that line the lumen of the respiratory tract also can be cleared by specific Abs that are delivered by parenteral inoculation or by direct instillation into the lungs. RSV immune globulin or RSV-neutralizing Abs protect against severe lower respiratory tract disease.1,2 These Abs also reduce established infection in animal models; however, therapeutic trials in humans have not revealed a clinical benefit of treatment of active infection. Influenza A virus HA-specific IgG Abs cleared the lungs of influenza-infected SCID mice in the absence of other recognized immune functions.334 Abs can exert a therapeutic effect in privileged sites where CD8 CTLs are not active. Central nervous system (CNS) neurons are not good targets for CD8 CTLs, because they have highly regulated expression of class I MHC glycoproteins. Nevertheless, in SCID mice, persistent infection of brain neurons by the alphavirus Sindbis virus can be cleared rapidly by parenteral inoculation of envelope-glycoprotein–specific Abs without causing obvious cell damage.248 Some patients with underlying X-linked agammaglobulinemia complicated by chronic enteroviral meningoencephalitis have been treated successfully with human IgG that contains Abs specific for the infecting virus. A therapeutic effect was achieved with IgG administered by the intravenous or intraventricular routes.118,275 Failure of human IgG to alter chronic enteroviral meningoencephalitis in some patients suggests that the concentration of enterovirus-specific Abs in human IgG is often inadequate.
Mucosal Antibodies
Abs have been shown to function within mucosal epithelium.268,269 Dimeric immunoglobulin A (IgA) secreted at the basolateral surface of cells or from plasma is transcytosed to the apical surface in association with polymeric Ig receptor to which dimeric IgA with J chain binds. During transcytosis through cells infected with the Sendai virus, virus-specific IgA can reduce intracellular virus and infectivity. The mechanism responsible for this effect is not clear, although it is possible that the endocytic pathway used for IgA transport and the exocytic pathways used for transport of viral glycoproteins intersect at some location within the cell, possibly the apical recycling endosome.142 Similar studies with IgA Abs to rotavirus and measles virus suggest that IgA molecules may inhibit viruses inside epithelial cells or after transcytosis.50,463 Induced IgA molecules to HIV gp41 appear to block transcytosis across genital epithelial surfaces.42 The FcRn receptor for IgG also is present in some epithelial cells and could participate in transport or retention of IgG at mucosal surfaces.
Abs present in the systemic circulation efficiently protect internal organs against viruses that are introduced directly into the bloodstream (e.g., HBV and HIV) or that spread via the bloodstream from primary sites of replication such as the respiratory or gastrointestinal mucosa. As mentioned previously,
diseases produced by viruses that fall into the latter category (i.e., measles, polio, hepatitis A, rubella, smallpox, and varicella) can be prevented or modified by Ig prophylaxis, often with small amounts of Ab that are difficult to detect in the blood of recipients. Such Abs are relatively easy to induce by parenteral immunization with live or inactivated virus vaccines. In contrast, Abs present in the systemic circulation do not provide efficient protection against viral diseases that are limited to mucosal surfaces unless these Abs are present in high titer. This is because only a small proportion of such Ig molecules traffic from the plasma to the lumenal surface of the mucosal epithelium by transudation. Therefore, to prevent viral diseases that are limited to mucosal tissue, two different strategies can be employed. The first is to induce Abs such as secretory IgA at the mucosal site by virus replication or immunization at that site. The second, and less efficient, strategy is to stimulate a high titer of serum IgG Abs that can protect mucosal surfaces following transudation.
diseases produced by viruses that fall into the latter category (i.e., measles, polio, hepatitis A, rubella, smallpox, and varicella) can be prevented or modified by Ig prophylaxis, often with small amounts of Ab that are difficult to detect in the blood of recipients. Such Abs are relatively easy to induce by parenteral immunization with live or inactivated virus vaccines. In contrast, Abs present in the systemic circulation do not provide efficient protection against viral diseases that are limited to mucosal surfaces unless these Abs are present in high titer. This is because only a small proportion of such Ig molecules traffic from the plasma to the lumenal surface of the mucosal epithelium by transudation. Therefore, to prevent viral diseases that are limited to mucosal tissue, two different strategies can be employed. The first is to induce Abs such as secretory IgA at the mucosal site by virus replication or immunization at that site. The second, and less efficient, strategy is to stimulate a high titer of serum IgG Abs that can protect mucosal surfaces following transudation.
When IgG Abs present in the blood are passively transferred to mucosal surfaces by transudation where they can exert antiviral activity, there is a gradient regarding the ability of serum IgG-derived Abs to restrict virus replication on mucosal surfaces. This gradient is less in lung than it is in the nasopharynx. IgG Abs, if present in the serum in high enough titer, can provide almost complete resistance to pulmonary replication of RSV; however, resistance in the upper respiratory tract is more difficult to achieve. Transudation of IgG also probably occurs in the intestine, especially in inflammatory states, although IgG is more easily subjected to proteolytic degradation in the intestinal lumen than in the respiratory tract. Immunization of the female genital tract with a live virus vaccine can induce the local synthesis of IgG antiviral Abs such that the specific activity of mucosal IgG Abs significantly exceeds that of serum, indicating that IgG Abs present on a mucosal surface can come either from serum via transudation or from IgG Ab-secreting cells present in the submucosal tissues.340 Although passively transferred IgG Abs can provide mucosal immunity in the lower respiratory tract, the major mediators of resistance to virus infection of the upper respiratory tract, the larger airways of the lungs, and the gastrointestinal tract are mucosal Abs, many of which are IgAs selectively transported across mucosal surfaces to exert antiviral effects on the lumenal surface. Both nonneutralizing50 and neutralizing379 IgA Abs have antiviral effects on mucosal surfaces, with the former active intracellularly during transcytosis and the latter active both intracellularly and on the lumenal side of the epithelium. Antiviral IgA Abs function to clear virus infections, to modify the severity of disease on reinfection, and to prevent infection on re-exposure to virus. The level of antiviral IgA Abs, as well as the number of virus-specific IgA-secreting cells, often correlates with resistance to virus infection.83,469 The advantages of IgAs in the mucosal fluids are that they are transported more efficiently, they resist proteolytic degradation better than IgG, and the dimeric nature of secretory IgA enhances avidity.
The primary mucosal IgA response peaks within the first 6 weeks after infection and usually decreases to a low, often barely detectable, level by 3 months. The transient nature of the primary mucosal Ab response is a factor in the ability of many viruses to reinfect mucosal surfaces and underlies the need for two or more doses of vaccine to efficiently immunize mucosal surfaces. The virus-specific IgA Ab response is greater at the site of virus replication or of Ag administration than at distal sites, suggesting that mucosal immunity is best induced by antigenic stimulation of sites directly involved in replication of the wild-type virus.
Adverse Effects of Antibodies
Abs can contribute to the potentiation of disease. For example, heterotypic Abs to the envelope glycoprotein of dengue virus appear to play a role in the severe clinical entity known as dengue hemorrhagic fever/shock syndrome.231 Dengue viruses replicate primarily in cells of the mononuclear phagocyte lineage, and subneutralizing amounts of dengue virus Abs enhance dengue virus infection of these cells in vitro by increasing uptake of virus via IgG Fc receptors present on these cells.180 However, the mechanism by which augmented disease results from enhanced access of dengue virus into mononuclear cells remains unclear.377 Immune complexes were detected in the lung tissues of fatal cases of influenza during the 1957 and 2009 pandemics, suggesting a potential role for enhancing Abs in severe influenza disease.287
Obstacles to Immunization in Early Life and in the Elderly
Immunization of Infants
The developmental immaturity of the immune response to viruses in the very young is seen clearly in the difficulty to immunize neonates and infants.402 Young infants are a preferred target population for immunization, because most children present to health systems with the highest frequency at birth or shortly after. A high level of access to infants allows universal immunization, and many viral diseases are most severe early in life. Many obstacles arise, however, in attempts to immunize infants in the first several weeks or months of life against disease caused by viruses.
First, most infants possess maternal Abs that can interfere with response to infection or the infectivity or immunogenicity of primary immunization. Live attenuated measles virus cannot be given effectively prior to 1 year of age, because many infants possess maternal Abs until that time that reduce or eliminate immunogenicity of the vaccine.10 Ab-mediated suppression can be overcome in some instances by increasing the dose of the vaccine—for example, the inactivated hepatitis A vaccine or a replicating influenza vaccine.84,101 The immune suppression by maternal Abs is more potent against B-cell responses than against induction of virus-specific T cells, possibly because the Ab response generally depends more on Ag dose than T-cell responses.97
Second, the ability to respond to viral surface proteins with a protective Ab response is acquired gradually over the first year of life, reflecting developmental maturation of the neonatal immune system. Responses of seronegative infants to live measles vaccine remain relatively poor during the second 6 months of life.144 Responses to live VZV vaccine also are reduced in the first year of life.436 The youngest infants mount Ab responses to experimental mucosal immunization with live influenza, RSV, or parainfluenza virus vaccines that are low in magnitude, quality, and durability.84,213,214 The molecular and cellular basis for immunological immaturity is not fully defined. Infant virus-specific Ab sequences are characterized
by marked lack of somatic mutations, resulting in low-affinity Abs of low function.448 Infant B cells exhibit limited capability to switch isotype from IgM to IgG2 Abs, and the isotype profile of circulating Abs differs from adults until age 5 to 6 years. Infants possess reduced levels of some components of the complement cascade, especially C8 and C9 that participate in membrane attack complexes, leading to impaired complement-mediated reactions. Infants exhibit a high proportion of transitional immature B cells and poor organization of the splenic marginal zone.
by marked lack of somatic mutations, resulting in low-affinity Abs of low function.448 Infant B cells exhibit limited capability to switch isotype from IgM to IgG2 Abs, and the isotype profile of circulating Abs differs from adults until age 5 to 6 years. Infants possess reduced levels of some components of the complement cascade, especially C8 and C9 that participate in membrane attack complexes, leading to impaired complement-mediated reactions. Infants exhibit a high proportion of transitional immature B cells and poor organization of the splenic marginal zone.
The Ab responses that young infants make to respiratory virus surface glycoproteins are significantly lower in quality than those of adults. Studies have described a dissociation of the level of neutralizing and binding Abs in the very young infant.457 This dissociation may be of functional significance, because neutralizing Ab titers correlate well with protective efficacy in studies of protection for viruses. Dissociation between neutralizing and binding Abs also characterized the serum Abs induced by the ill-fated formalin-inactivated RSV experimental vaccine of the 1960s and probably accounts for the lack of protective efficacy of Abs induced by that vaccine candidate.301 Ab responses induced by infections of infants are less durable than those of older subjects. Postinfection serum Ab levels to respiratory viruses often wane significantly several months after primary infection, especially if infection occurs at a very young age. Animal models suggest that B-cell priming occurs effectively early in life or in the presence of suppressive Abs96,97; however, the deficient maintenance of long-lived plasma cells in the bone marrow may lead to rapid waning of humoral immunity.349 Reduced humoral immunity to vaccines early in life also may reflect reduced T-helper cell function and altered dendritic cell function. Neonatal CD4 T cells exhibit reduced ability to secrete IFN-γ, reflecting limited secretion of IFN-α and IL-12 by dendritic cells.106,155
Immunization of the Elderly
Obstacles to effective immunization also arise late in life owing to immune senescence. Immune senescence in the elderly is marked by a decrease in the magnitude, quality, and duration of Ab responses and a reduction in functional T cells, especially CD8 T cells. Elderly T cells are distinguished by alterations in cytokine production, co-stimulatory molecule expression, susceptibility to apoptosis, and changes in the level of DNA damage and repair and telomerase length.145 Chronic virus infection may contribute to immune senescence. For example, CMV-specific T cells progress to a differentiated state in the elderly owing to chronic antigenic stimulation.344 This syndrome has been described as an immune risk phenotype in the elderly, reflecting the accumulation of a high percentage of circulating CMV-specific CD8 T cells at a terminal stage of differentiation that may detract from the flexibility of the T-cell repertoire to respond to other Ags. The CD4 T-cell responses of healthy elderly individuals appear to sustain a good level of function until late in life. Waning immunity to latent viruses may be boosted in the elderly in some cases. Immunization of the elderly with live attenuated varicella virus vaccine reduces the incidence of shingles in that population.333
Aging has varying effects on the response to different Ags. For example, the immunogenicity of recombinant hepatitis B vaccine is reduced starting in the fifth decade and is poor in the elderly.455 Ab responses to influenza virus vaccine also are reduced in the elderly. Practically speaking, however, although vaccination in the elderly may be reduced in efficacy and may require frequent boosters or other alterations in strategy, the effectiveness of many vaccines such as influenza virus vaccine are reasonable enough to support vaccination of this group. It also may be possible to improve the immune responses in the elderly by utilizing adjuvants or alternative vaccination strategies. Investigational gene-based vaccines have been shown to be highly immunogenic in older adults compared to the typical age-related decrease in responses seen with many traditional vaccines.243
Goals of Immunization Against Viral Diseases
Prevention
The major goals of immunization against viruses are the prevention or modification of disease in an individual and the control of epidemic infection within populations. Most viral vaccines prevent or modify disease without necessarily preventing infection. The ways in which this objective is achieved are diverse. Whereas most viral vaccines are administered before infection occurs, prevention of CNS disease in individuals inoculated with rabies virus by an animal bite can be achieved by administration of a vaccine regimen initiated shortly after infection has occurred. This postexposure prophylaxis is possible because the long incubation period of the infection permits the development of an effective immune response in time to modify infection and prevent disease. Interestingly, even for viruses with a more rapid course, such as variola, individuals can be protected against a fatal outcome if vaccinia virus vaccine is administered up to 4 days after the initial exposure to the virus. This effect may be attributable to augmented innate immune responses or more rapid induction of adaptive immune responses.
The major goal of immunization against rubella virus is the prevention of fetal abnormalities caused by intrauterine infection. To achieve this goal, both males and females are immunized in the United States. Immunization of males has little direct benefit to the vaccinee other than prevention of the relatively mild illness caused by the rubella virus, and males are not targeted in all national vaccine programs. The fetus of a vaccinated female is protected by immunization of the mother before pregnancy. Herd immunity resulting from extensive immunization of males and females provides some protection to the fetus of an unvaccinated female by decreasing the circulation of virus in the community. An example of herd immunity was seen early after introducing the mumps vaccine. The incidence of mumps declined precipitously before vaccine coverage was significantly expanded. A modest reduction in the number of susceptible persons and transmitters had a significant impact on the epidemic (Fig. 14.2) and e-Fig. 14.2.
In addition to reducing the frequency of susceptible persons and transmitters, some live viral vaccines can improve herd immunity by the spread of vaccine from recipients to contacts. This phenomenon has been observed with oral live poliovirus vaccine383 resulting in the indirect immunization of a significant proportion of the population and a marked reduction in the incidence of poliomyelitis in both vaccinated and “unvaccinated”
individuals. Spread of vaccine virus has been viewed by many as a desirable property because this transmission confers the benefits of immunization on many individuals who were not vaccinated. However, spread of vaccine in the population also raises concern because of the rare occurrence of vaccine-associated paralytic disease among contacts of vaccinees, and immunization of persons without their prior consent presents ethical issues.
individuals. Spread of vaccine virus has been viewed by many as a desirable property because this transmission confers the benefits of immunization on many individuals who were not vaccinated. However, spread of vaccine in the population also raises concern because of the rare occurrence of vaccine-associated paralytic disease among contacts of vaccinees, and immunization of persons without their prior consent presents ethical issues.
Although most vaccines in use today are effective in preventing disease, they usually are inefficient at completely preventing infection. Prevention of infection is a desirable property for specific vaccines such as HBV and HIV. Infection with HBV during the neonatal period is associated with an increased risk of progression to the chronic carrier state and associated with the development of chronic liver disease and hepatocellular carcinoma later in life. Therefore, a major goal of HBV vaccination is to prevent transmission of infection from persistently infected mothers to their infants during the neonatal period. Considerable success in this effort has been achieved through the use of hepatitis B surface antigen (HBsAg) vaccine or hyperimmune HBV antiserum plus vaccine immediately after delivery.195 HIV is a lentivirus, and the possibility of progression to AIDS can occur many years after initial infection. Therefore, it is desirable to develop a vaccine that induces immunity to prevent infection or result in an abortive or transient HIV infection.
Immunotherapy
Herpes simplex virus type 1 (HSV-1) or type 2 (HSV-2) and VZV produce latent infections in sensory ganglia that periodically reactivate, resulting in development of new lesions and increased opportunity for transmission of virus. Therefore, a goal of immunization of persons previously infected with these viruses is to prevent or ameliorate recurrent disease caused by reactivation of their latent infection.35 This form of immunization is referred to as immunotherapy. Other illnesses that are candidates for immunotherapeutic vaccines include warts and cancer caused by HPV and chronic hepatitis caused by HBV or HCV. HIV-1 has also been considered as a target for immunotherapy to prevent the long-term consequences of chronic infection or to reduce the requirements for ongoing antiretroviral drug therapy. Because of the extent of repeated Ag exposure and damage to many elements of the adaptive immune system, it may be difficult to elicit new immune responses to effectively control virus.62 Effective immunotherapeutic vaccines for recurrent HSV face a similar challenge because the vaccinees previously infected by wild-type virus are repeatedly reimmunized during each recurrence. However, for a related herpesvirus, VZV, where recurrence is relatively uncommon, immunization with live attenuated vaccine reduces the incidence of herpes zoster and postherpetic neuralgia.333
Eradication or Elimination
Appropriate use of a highly effective vaccine can help to eradicate a major disease when humans are the only natural host for the virus and there is no natural reservoir or intermediate host. During the 1970s, this goal of eradication was achieved for smallpox largely through the use of live vaccinia virus vaccine. This campaign ranks as one of the major public health achievements in all history. Two other human viruses have been targeted similarly for eradication, namely poliovirus and measles virus, and significant progress toward these goals has been made for both viral pathogens. The United Nations officially declared eradication of the morbillivirus animal pathogen rinderpest in 2011, after a concerted worldwide eradication program. Unfortunately, eradication of viral pathogens usually is an elusive goal because of setbacks from social instability, antivaccine sentiments, the threat of bioterrorism, and the potential for intentional reintroduction or resurrection of previously eradicated viral pathogens. Therefore, it will be prudent to find ways of maintaining immunity to serious virus pathogens even after eradication of virus in the natural reservoir has been achieved. The new technologies that have improved our ability to identify emerging pathogens and to develop biologics for vaccines and therapeutics have also created an intellectual reservoir that is a formidable barrier to true eradication efforts. Elimination of a virus pathogen suggests that epidemic and endemic disease is controlled and no active cases are present. However, setting the goal of elimination acknowledges the possibility of re-emergence and would include maintenance of active vaccine-induced immunity.
History of Viral Vaccine Development and Future Prospects
The history of discovery and development for each viral vaccine is unique and instructional. Although space limitations prevent the full description of each vaccine, some of the major events are outlined. Although each vaccine development story is different, there are common events and themes that have been catalogued in a supplementary table (e-Table 14.1) that includes (a) disease description and virus discovery, (b) vaccine discovery and/or proof of concept, (c) vaccine licensure or first human use, and (d) unique or unanticipated events.
Origins of Vaccinology
Origin of Live Virus Vaccination
Most viral vaccines were developed as a result of both fortuitous events and empirical findings. The origin of modern vaccinology, and particularly live virus vaccination, is based on the practice of variolization (mechanical attenuation and
intentional low-dose infection) to reduce the virulence of subsequent smallpox infection, which was initiated more than 1,000 years ago in India and China. From this arose the concept of vaccination (using an alternative agent to provide immunity against an infectious disease) and the first vaccine used in humans—live cowpox virus. In 1796, Edward Jenner inoculated 8-year old James Phipps with purulent material taken from a cowpox pustule on the hand of milkmaid Sarah Nelmes and introduced it into an incision on the boy’s arm. Jenner subsequently proved that the boy was protected from an inoculation of material from a smallpox lesion. He published the work in 1798 and coined the word vaccine from the Latin vacca for cow, designating the process vaccination. For 80 years, live virus was transferred almost exclusively from human arm to arm by collection of purulent material. Subsequently, vaccine was propagated on the skin of calves using serial inoculation, resulting in vaccinia virus as the distinct vaccine strain.260 This vaccine was used successfully to eradicate smallpox from the population, and smallpox remains the only human virus infection to be eradicated through a vaccination campaign (Fig. 14.3).
intentional low-dose infection) to reduce the virulence of subsequent smallpox infection, which was initiated more than 1,000 years ago in India and China. From this arose the concept of vaccination (using an alternative agent to provide immunity against an infectious disease) and the first vaccine used in humans—live cowpox virus. In 1796, Edward Jenner inoculated 8-year old James Phipps with purulent material taken from a cowpox pustule on the hand of milkmaid Sarah Nelmes and introduced it into an incision on the boy’s arm. Jenner subsequently proved that the boy was protected from an inoculation of material from a smallpox lesion. He published the work in 1798 and coined the word vaccine from the Latin vacca for cow, designating the process vaccination. For 80 years, live virus was transferred almost exclusively from human arm to arm by collection of purulent material. Subsequently, vaccine was propagated on the skin of calves using serial inoculation, resulting in vaccinia virus as the distinct vaccine strain.260 This vaccine was used successfully to eradicate smallpox from the population, and smallpox remains the only human virus infection to be eradicated through a vaccination campaign (Fig. 14.3).
Origin of Inactivated Virus Vaccination
In the 1880s, Louis Pasteur and his colleagues attributed rabies to virus replication to the CNS. Next, they discovered that drying rabies-infected tissue at room temperature attenuated the infectivity of the tissue. The dried rabies-infected tissue was determined to be noninfectious, although serial inoculation of dogs with the tissues provided protection against an otherwise lethal rabies virus challenge. Inoculation of dried rabies-infected CNS tissue was tested in a single human postexposure case in 1885 when Pasteur serially inoculated Joseph Meister, a 9-year-old boy bitten by a rabid dog more than 2 days earlier. The initial inoculations contained highly attenuated virus from rabbit spinal cord dried for 15 days, followed by inoculations with less attenuated virus-infected tissue. In total, the regimen included 13 immunizations over 10 days. The boy was fully protected from disease, representing the beginning of an era in which inactivated vaccines were used for other viral diseases following Pasteur’s paradigm of identification, inactivation, and inoculation (Fig. 14.4) and e-Table 14.1.
Critical Events in the History of Viral Vaccines
Since the introduction of vaccinia virus (Chapters 66 and 67), licensed vaccines have been developed for 15 other viral pathogens (Table 14.1). Notable advances in viral vaccine development over the past century include semieradication of many virus infections; processes for optimization of manufacturing cell lines for next-generation vaccines; utilization of human immune correlates to establish efficacy for licensure; and improvements in vaccine safety monitoring, which is important for public safety but also serves to keep safe and efficacious vaccines from unwarranted negative assessment.
Semieradication Secondary to Effective Vaccine Campaigns
Several vaccines have resulted in regional semieradication of virus disease or dramatic reduction in the incidence or morbidity from infection (polio, measles, mumps, rubella, rabies, and VZV). Other vaccines are highly effective and have been reduced to use only for travelers to endemic areas of the world (yellow fever, Japanese encephalitis). Licensed vaccines for influenza and hepatitis A and B are highly effective, and their impact on frequency of infection has been improved by new strategies for distribution (Table 14.2).
Modernization of Vaccine Manufacturing
The process of manufacturing a vaccine is complex, and the primary cornerstone in overall production is the substrate used for vaccine growth or antigen production. Early-generation vaccines were often grown in animal organ cells, including adenovirus vaccine in monkey kidney cells and Japanese encephalitis virus (JEV) vaccine in mouse brains. During the second half of the 20th century, modernization of vaccine production included the progression to use of cell lines as substrates, including current JEV vaccine in Vero cells and hepatitis B vaccine in yeast. Influenza vaccine researchers continue to develop U.S. marketed vaccines using chicken eggs as the substrate but have developed cell culture–based substrates that are used effectively in some European countries. The transition to cell-based substrates offers a more efficient, more reliable, and more stable manufacturing process.
Surrogate End Points for Vaccine Efficacy
Traditionally, the assessment of vaccine efficacy has been made by determining whether infection or disease has been
prevented. In the process of establishing vaccine efficacy, the use of a human immune correlate of protection is potentially more efficient and has been implemented successfully in the yearly approval process for influenza vaccines. Influenza vaccines typically are approved based on the accepted surrogate of protection, a hemagglutination inhibition (HAI) titer of 1:40 or higher or a fourfold increase over the prevaccination HAI titer. In this case, the immune response serves as a surrogate marker that is predetermined to predict clinical benefit; therefore, the more lengthy process of assessing the presence or absence of influenza infection in a clinical trial is not always needed (http://www.fda.gov/BiologicsBloodVaccines/GuidanceComplianceRegulatoryInformation/Guidances/Vaccines/ucm074794.htm). Similarly, assessing the end result of infection (or absence of) rather than documenting an effect on the virus itself can also be used to assess protective efficacy. HPV vaccines were licensed based on efficacy derived from the prevention of cervical and vulvar cancer, precancerous lesions, and dysplastic lesions (http://www.fda.gov/BiologicsBloodVaccines/Vaccines/ApprovedProducts/ucm111283.htm).
prevented. In the process of establishing vaccine efficacy, the use of a human immune correlate of protection is potentially more efficient and has been implemented successfully in the yearly approval process for influenza vaccines. Influenza vaccines typically are approved based on the accepted surrogate of protection, a hemagglutination inhibition (HAI) titer of 1:40 or higher or a fourfold increase over the prevaccination HAI titer. In this case, the immune response serves as a surrogate marker that is predetermined to predict clinical benefit; therefore, the more lengthy process of assessing the presence or absence of influenza infection in a clinical trial is not always needed (http://www.fda.gov/BiologicsBloodVaccines/GuidanceComplianceRegulatoryInformation/Guidances/Vaccines/ucm074794.htm). Similarly, assessing the end result of infection (or absence of) rather than documenting an effect on the virus itself can also be used to assess protective efficacy. HPV vaccines were licensed based on efficacy derived from the prevention of cervical and vulvar cancer, precancerous lesions, and dysplastic lesions (http://www.fda.gov/BiologicsBloodVaccines/Vaccines/ApprovedProducts/ucm111283.htm).
Table 14.1 Viral Vaccines Licensed in the United Statesa | |||||||||||||||||||||||||||||||||||||||||||||||||||||||||||||||||||||||||||||||||||||||||||||||||||||||||||||||||||||||||||||||||||||||||||||||||||||||||||||||||
---|---|---|---|---|---|---|---|---|---|---|---|---|---|---|---|---|---|---|---|---|---|---|---|---|---|---|---|---|---|---|---|---|---|---|---|---|---|---|---|---|---|---|---|---|---|---|---|---|---|---|---|---|---|---|---|---|---|---|---|---|---|---|---|---|---|---|---|---|---|---|---|---|---|---|---|---|---|---|---|---|---|---|---|---|---|---|---|---|---|---|---|---|---|---|---|---|---|---|---|---|---|---|---|---|---|---|---|---|---|---|---|---|---|---|---|---|---|---|---|---|---|---|---|---|---|---|---|---|---|---|---|---|---|---|---|---|---|---|---|---|---|---|---|---|---|---|---|---|---|---|---|---|---|---|---|---|---|---|---|---|---|
|
Table 14.2 Impact of Licensed Vaccines on Viral Diseases with Well-Defined Surveillance and Estimated Impact on Disease Burden for Other Viral Vaccines | ||||||||||||||||||||||||||||||||||||||||||||||||||||||||||||||||||||||||||||||||||||
---|---|---|---|---|---|---|---|---|---|---|---|---|---|---|---|---|---|---|---|---|---|---|---|---|---|---|---|---|---|---|---|---|---|---|---|---|---|---|---|---|---|---|---|---|---|---|---|---|---|---|---|---|---|---|---|---|---|---|---|---|---|---|---|---|---|---|---|---|---|---|---|---|---|---|---|---|---|---|---|---|---|---|---|---|
|
Maintaining Vaccine Availability
Vaccine efficacy, need, utilization, and safety (or perceived safety) all have a significant impact on the success of licensure and sustained availability of a vaccine. Two licensed vaccines that are no longer available are the adenovirus serotypes 4 and 7 and the tetravalent rhesus rotavirus vaccine. Production of the adenovirus vaccine, selectively given to new military recruits, was stopped by the sole manufacturer in 1995 for economic reasons. Since then, morbidity from adenovirus infections has increased among military recruits, and epidemics have occurred in training centers.169 In addition to economic concerns, safety concerns have also prompted an abrupt end to the use of vaccines. Specifically, RotaShield, a live tetravalent rhesus rotavirus reassortant vaccine against the four major prevalent serotypes (based on VP7, a major outer capsid glycoprotein), was effective but was later withdrawn from market. A postmarketing analysis revealed that the vaccine was associated with an excess rate of intussusception of up to 1 per 2,500 vaccinees that occurred in the immediate postvaccination period, predominantly following the first dose of vaccine.306 Following withdrawal of RotaShield, subsequent studies led to a significant downward revision of these estimates and have suggested that the early period of increase in intussusception seen after the first dose was followed by a period of compensatory decrease such that there was no net overall increase in the number of cases in vaccinees less than 1 year of age.306 Furthermore, the excess cases of intussusception occurred mostly when the vaccine was used in children 3 months of age or older to “catch up” rather than in vaccinees who received the ideally recommended schedule of 2, 4, and 6 months of age.212,403 This case highlights the importance of the analysis of postmarketing data, which needs to be especially thorough and comprehensive because it can dramatically impact the availability of a vaccine that may otherwise have a
favorable risk/benefit ratio. Despite the initial conclusions and withdrawal of RotaShield from the market, two newer versions of a rotavirus vaccine have been developed. Pentavalent RotaTeq has been licensed in the United States for infants less than 3 months of age. It consists of a mixture of live attenuated, bovine/human reassortant viruses that express a single VP4 (P1A) as well as VP7 (G) Ag from G1 through G4 strains of rotavirus and VP4 (P1A[8]). P1A is the most common VP4 in G1, G3, and G4 strains and is present in a major portion of G9 viruses that are becoming more prevalent in some regions. The second new vaccine is a monovalent VP7 G1:P1A[8] attenuated human rotavirus strain that was first licensed in Mexico and the Dominican Republic151 and later in 2008 in the United States. These two vaccines protect against severe gastroenteritis attributable to rotavirus. Large-scale postlicensure evaluations will be essential to more clearly elucidate the overall risks associated with rotavirus vaccines in the general population.
favorable risk/benefit ratio. Despite the initial conclusions and withdrawal of RotaShield from the market, two newer versions of a rotavirus vaccine have been developed. Pentavalent RotaTeq has been licensed in the United States for infants less than 3 months of age. It consists of a mixture of live attenuated, bovine/human reassortant viruses that express a single VP4 (P1A) as well as VP7 (G) Ag from G1 through G4 strains of rotavirus and VP4 (P1A[8]). P1A is the most common VP4 in G1, G3, and G4 strains and is present in a major portion of G9 viruses that are becoming more prevalent in some regions. The second new vaccine is a monovalent VP7 G1:P1A[8] attenuated human rotavirus strain that was first licensed in Mexico and the Dominican Republic151 and later in 2008 in the United States. These two vaccines protect against severe gastroenteritis attributable to rotavirus. Large-scale postlicensure evaluations will be essential to more clearly elucidate the overall risks associated with rotavirus vaccines in the general population.
Future Directions in Vaccinology
In recent decades, major advances in viral vaccine development have coincided with the emergence of new technologies (Fig. 14.5). Therefore, selected technologies that are likely to influence future vaccine design are discussed briefly.
Impact of Advances in High-Throughput Sequencing Technology
The Human Genome Project simulated major investments in sequencing technology, resulting in breakthroughs that have increased throughput by orders of magnitude. As a result, it is now feasible to sequence all nucleic acids in samples of interest, such as vaccine preparations. Not surprisingly, such experiments have identified previously unidentified agents or nucleic acids in licensed vaccines, such as porcine circovirus type 1 (PCV1) in the commercial rotavirus vaccine Rotarix.438 The U.S. Food and Drug Administration (FDA) temporarily suspended use of the vaccine in 2010; however, experts agreed that this highly prevalent nonpathogenic pig virus does not infect humans, and the use of vaccine was reinstituted. Some vaccines are produced in avian or primate cell lines, and thus it was not surprising that RNAs from avian leukosis virus, which is noninfectious for humans, or genetically defective DNAs from simian retrovirus (SRV) were present in products of such cell lines. Another interesting finding of such sequencing efforts is that the vaccine virus populations contain minority variants with polymorphisms or mutations (compared to the known sequence of the vaccine strains). Such variants have been observed for oral polio vaccine (OPV), mumps virus, and VZVs but are not known to affect attenuation.
Advances in Monoclonal Antibody Isolation
The neutralizing determinants on vaccine Ags are de facto the epitopes recognized by human Abs that mediate antiviral function. The study of the molecular and structural basis for neutralization has been limited by the difficulty in isolating human mAbs. Recent breakthroughs in this field using enhanced EBV transformation with CpG,34 human hybridoma formation,467 plasmablast sorting and cloning,456 single B-cell sorting and expansion,440 and other techniques have increased the numbers of human Abs for study by several orders of magnitude. Structural studies of complexes of human Abs with viral Ags have begun to yield fascinating insights into the features of protective Ab epitopes, which often are conformational, quaternary, and otherwise complex.237
Advances in Structural Biology
The ability to design optimal antigenic surfaces for induction of neutralizing Abs has long been a dream of vaccine scientists. The goal is to develop synthetic constructs that elicit Abs with predetermined structural specificity. In particular, induction of Abs against epitopes that are hidden in the native viral protein has been a challenge for vaccine design. Investigators working in the areas of structural biology and computational modeling
recently have made significant progress in this regard. Computationally designed epitope-scaffolds that are immunogenic have been developed for the HIV-1 gp41 epitopes of the broadly neutralizing mAbs 2F5326 and 4E1093 and the RSV F motavizumab epitope.274 Structural information from HIV gp120 CD4 binding site epitope recognized by the neutralizing Ab b12 has been used to design gp120 reagents to select additional CD4 binding site Abs that have broad neutralizing activity against HIV.474 Fragments of influenza HA have been expressed to focus the immune response to conserved epitopes in the HA stem region.41 Computational and structural tools also can be used for design of viral inhibitors—for example, the design of proteins targeting the influenza HA stem that mimic the action of neutralizing Abs. It seems likely that computationally designed Ags will increasingly influence viral vaccine design.
recently have made significant progress in this regard. Computationally designed epitope-scaffolds that are immunogenic have been developed for the HIV-1 gp41 epitopes of the broadly neutralizing mAbs 2F5326 and 4E1093 and the RSV F motavizumab epitope.274 Structural information from HIV gp120 CD4 binding site epitope recognized by the neutralizing Ab b12 has been used to design gp120 reagents to select additional CD4 binding site Abs that have broad neutralizing activity against HIV.474 Fragments of influenza HA have been expressed to focus the immune response to conserved epitopes in the HA stem region.41 Computational and structural tools also can be used for design of viral inhibitors—for example, the design of proteins targeting the influenza HA stem that mimic the action of neutralizing Abs. It seems likely that computationally designed Ags will increasingly influence viral vaccine design.
Live Virus Vaccines
History of Methods for Isolating Attenuated Strains
Varying methods have been used to achieve attenuation for live virus vaccines. Type 2 poliovirus, strain 712, one of the three poliovirus vaccine strains in the live vaccine preparation, is a naturally occurring attenuated human poliovirus that was identified by its lack of virulence for the brain and spinal cord of monkeys.382 Adenovirus vaccine strains (types 4 and 7) are wild-type human respiratory viruses used in the U.S. military that produced an asymptomatic infection following oral administration that was restricted in replication to the intestinal tract.68 The remaining attenuated vaccine viruses were derived from wild-type human viruses by serial passage in cell cultures prepared from an unnatural host, leading to the emergence of mutants that were partially restricted in humans at the portal of entry and/or the target organ(s). In this manner, attenuated mutants of rubella virus and types 1 and 3 poliovirus were selected after passage in monkey kidney tissue culture. Vaccine strains of yellow fever virus (17D strain) and measles virus were generated by multiple passage in chick embryo cell culture, whereas mumps virus was attenuated by multiple passages in embryonated eggs.
A critical feature of most successful live vaccine development programs is the identification of preclinical laboratory predictors of attenuation. For the yellow fever virus and poliovirus vaccines, promising vaccine strains were identified by their attenuation for experimental animals. In monkeys, the 17D strain of yellow fever virus exhibited decreased tropism for the liver, suggesting that this mutant might be suitably attenuated for humans423—a hypothesis that was confirmed during subsequent clinical trials. Naturally occurring or tissue culture–passaged strains of poliovirus were evaluated for neurovirulence in monkeys by intraspinal inoculation, which was a central innovation that allowed Sabin to identify attenuated strains.381,382 This testing system was selected because several observations suggested that the spinal cord of monkeys was more permissive than that of humans to the neuronolytic effect of poliovirus.382 Viruses that caused the least neurovirulence in monkeys were identified, and these strains then were cloned and recloned biologically by the plaque technique to yield progeny with the lowest possible level of monkey neurovirulence. These candidate vaccine strains then were subjected to additional cycles of selection, which led to the identification of mutants that replicated with high efficiency in the intestinal tract without significant increase in neurovirulence for nonhuman primates. These viruses were evaluated in clinical trials and were shown to be satisfactorily attenuated and immunogenic.
Experimental animal systems were not available for evaluating virulence of the other vaccine viruses. Therefore, these viruses were tested for attenuation directly in humans. Initial candidate measles and rubella virus vaccines were insufficiently attenuated, although further passage of rubella virus in monkey kidney cell culture and selection of a cold-adapted, temperature-sensitive mutant of measles virus yielded satisfactory vaccine strains. Attenuation of mumps virus passaged in eggs also was determined in humans. Interestingly, the Jeryl Lynn strain of mumps virus vaccine developed by Maurice Hilleman as a clinical isolate from his daughter was found to be a mixture of two closely related strains of mumps virus.7 Several other vaccines have been found to be mixtures of virus strains, including the yellow fever virus vaccine and some vaccinia stocks used for smallpox vaccination.
Poliovirus and adenovirus vaccines are administered orally, whereas yellow fever, rubella, measles, and mumps virus vaccines are given parenterally. Each of the currently licensed live virus vaccines is directed against a virus that has a complex pathogenesis of infection in which virus is introduced by inoculation (yellow fever) or by implantation on a mucosal surface (measles, mumps, rubella, and poliovirus) with subsequent spread systemically to the target organ(s). The requirement of such viruses to pass through blood to reach the target organ of disease makes them generally susceptible to serum Abs. It is more difficult to protect against infection by viruses that cause disease at a mucosal portal of entry without the need for viremia.
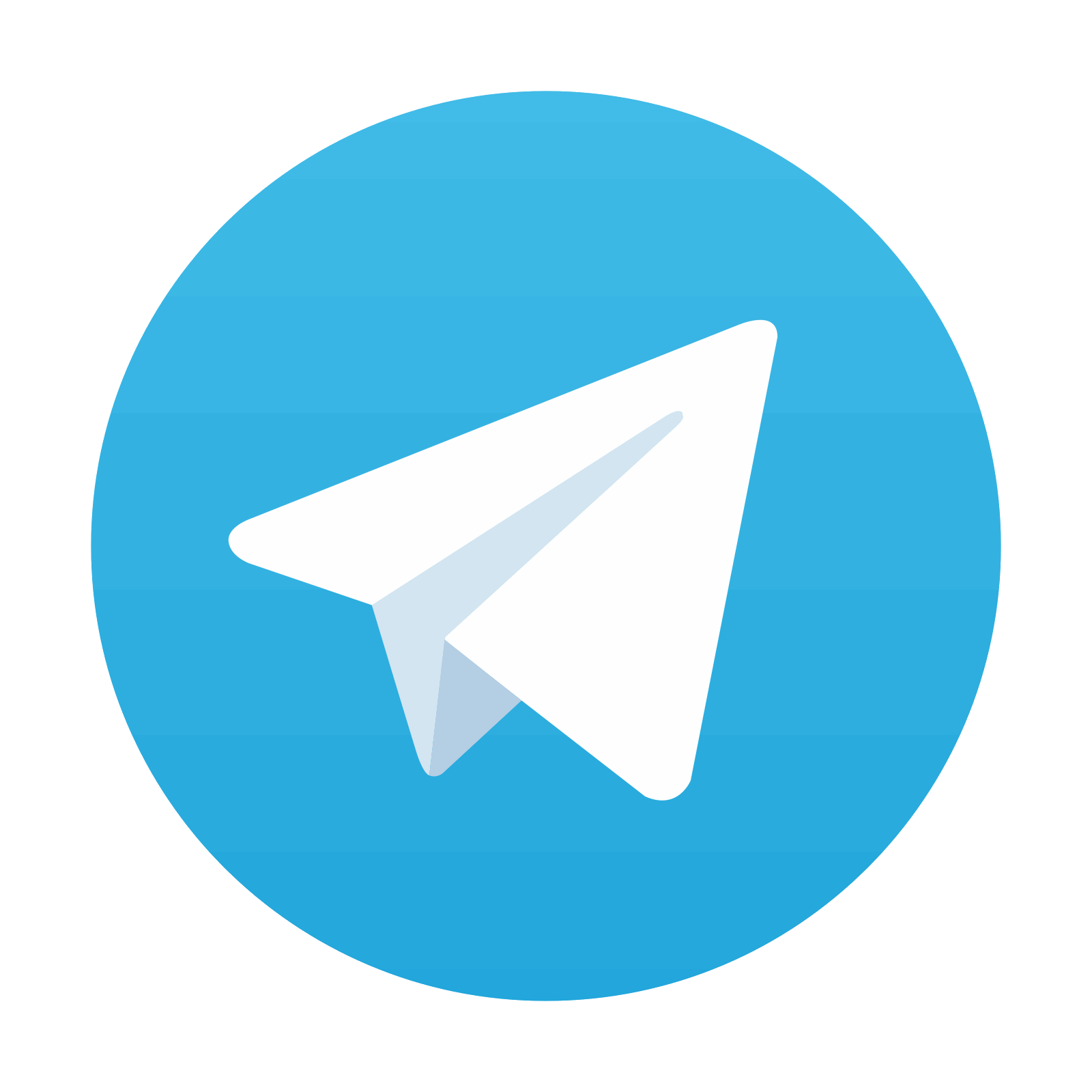
Stay updated, free articles. Join our Telegram channel

Full access? Get Clinical Tree
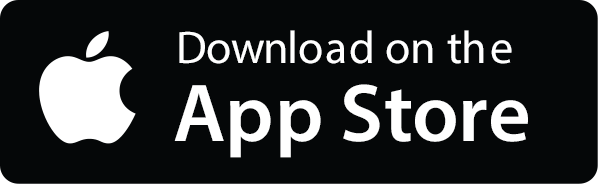
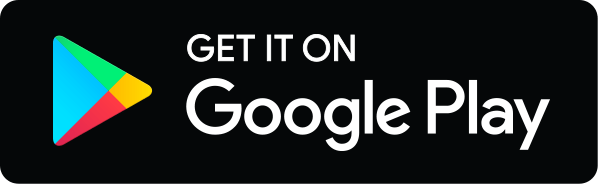