14 Immune defences in action
The barrier effects of the skin and mucous membranes and their adjuncts such as cilia have already been referred to (see Ch. 9). We now turn to the back-up mechanisms called rapidly into play when an organism has penetrated these barriers – namely, complement, the phagocytic and cytotoxic cells, and a variety of cytotoxic molecules. While they lack the dramatic specificity and memory of adaptive (i.e. lymphocyte-based) immune mechanisms, these natural defences are vital to survival – particularly in invertebrates, where they are the only defence against infection (adaptive responses only evolved with the earliest vertebrates).
In addition to these non-specific mechanisms, the immune system enables the specific recognition of antigens by T and B cells as part of adaptive immunity. Broadly speaking, antibodies are particularly important in combating infection by extracellular microbes, particularly pyogenic bacteria, while T-cell immunity is required to control intracellular infections with bacteria, viruses, fungi or protozoa. Their value is illustrated by the generally disastrous results of defects in T and/or B cells, or their products, discussed in more detail in Chapter 30. This chapter gives examples of how these different types of immunity contribute to the body’s defences against microbes.
Antimicrobial peptides protect the skin against invading bacteria
An interesting innate defence mechanism is the formation of neutrophil extracellular traps or NETS. Neutrophil serine proteinases such as cathepsin G, neutrophil elastase and proteinase 3 can be exocytosed by neutrophils to form neutrophil extracellular traps with chromatin that bind both Gram-positive and Gram-negative bacteria (Fig. 14.1) Of course, the bacteria can fight back, in this case through secreting DNAases or by having capsules to prevent entrapment.
Lysozyme is one of the most abundant antimicrobial proteins in the lung. Genetically engineered transgenic mice that had a lot more lysozyme activity than control mice in their bronchoalveolar lavage were much better at killing group B streptococci, and Pseudomonas aeruginosa (Fig. 14.2).
Complement
The alternative pathway and lectin binding pathways of complement activation are part of the early defence system
The basic biology of the complement system and its role in inducing the inflammatory response and promoting chemotaxis, phagocytosis and vascular permeability have been described in Chapter 9. Here we are concerned with its ability to directly damage microorganisms as part of the early response to infection. Contrary to what might be expected from the dramatic lysis of many kinds of bacteria in the test tube, the action of complement in vivo is restricted mainly to the Neisseria. Patients deficient in C5, C6, C7, C8 or C9 are unable to eliminate gonococci and meningococci, with the increased risk of developing septicaemia or becoming a carrier.
Acute phase proteins and pattern recognition receptors
C-reactive protein is an antibacterial agent produced by liver cells in response to cytokines
Among the acute phase proteins produced in the course of most inflammatory reactions, C-reactive protein (CRP) is particularly interesting in being an antibacterial agent, albeit of very restricted range. CRP is a pentameric β-globulin, somewhat resembling a miniature version of IgM (molecular weight 130 000 compared with 900 000 for IgM). It reacts with phosphorylcholine in the wall of some streptococci and subsequently activates both complement and phagocytosis. CRP is produced by liver cells in response to cytokines, particularly interleukin-6 (IL-6, see Ch. 11), and levels can rise as much as 1000-fold in 24 h – a much more rapid response than that of antibody (see Ch. 9). Therefore, CRP levels are often used to monitor inflammation, for example, in rheumatic diseases. Most of the other acute phase proteins are produced in increased amounts early in infection and have not just antimicrobial activity but can act as opsonins, antiproteases, play an immunomodulatory role or be involved in the fibrinolytic or anticoagulant pathways. For example, many of the complement components are acute phase proteins. Those with a role in protection against infection are also termed pattern recognition receptors, such as mannose-binding lectin. Some acute phase proteins such as lipopolysaccharide binding protein may reduce pathology by binding toxic bacterial products such as lipopolysaccharide.
Macrophages can recognize bacteria as foreign using Toll-like receptors
Another family of surface receptors, called Toll-like receptors, on macrophages and other cells, bind conserved microbial molecules such as lipopolysaccharide (LPS) (endotoxin), bacterial DNA, double-stranded RNA or bacterial flagellin. Pattern recognition receptors recognize these repeated structures (pathogen-associated molecular patterns, see Ch. 9) and this leads to release of proinflammatory cytokines such as tumour necrosis factor alpha (TNFα), IL-1 and IL-6. Signalling through the Toll-like receptors also leads to the increased expression of major histocompatibility complex (MHC) molecules and of co-stimulatory molecules, thus enhancing antigen presentation and usually leading to the activation of T-helper 1 (Th1) cells. It was recently suggested that a number of rare single nucleotide polymorphisms within the TLR4 gene (TLR4 binds endotoxin) were more common in people with meningococcal disease compared with controls.
Collectins and ficolins
Mannose-binding lectin (MBL) is another collectin found in serum. Binding of MBL to carbohydrates containing mannose on microorganisms leads to complement activation, through the mannan-binding lectin pathway. Bacteria opsonized by MBL bind to the C1q receptor on macrophages, leading to phagocytosis. Many individuals have low serum concentrations of MBL due to mutations in the MBL gene or its promoter. A recent study of children with malignancies showed that MBL deficiency increased the duration of infections. Lung surfactant proteins A and D, and MBL, bind to the surface spikes or S protein of the SARS virus (see Ch. 19), and so people with low MBL genotypes may be at increased risk of SARS infection.
Fever
A raised temperature almost invariably accompanies infection (see Ch. 29). In many cases, the cause can be traced to the release of cytokines such as IL-1 or IL-6, which play important roles in both immunity and pathology (see Ch. 11). However, the interesting question as to whether the raised temperature itself is useful to the host remains unresolved.
Natural killer cells
Natural killer cells are a rapid but non-specific means of controlling viral and other intracellular infections
NK cells can also act as cytotoxic effector cells, lysing host cells infected with viruses and some bacteria, as they make both cytotoxic granules and perforin. They recognize their targets by means of a series of activating and inhibitory receptors that are not antigen-specific. The inhibitory receptors recognize the complex of MHC class I and self peptide; if both this inhibitory receptor and another NK-cell-activating receptor are engaged, the NK cell will not be activated. However, if there is insufficient MHC class I on the cell surface, the inhibitory receptor is not engaged and the NK cell is activated to kill the target cell. This is an effective strategy, as some viruses inhibit MHC class I expression on the cells they infect. NK cells are therefore a more rapid but less specific means of controlling viral and other intracellular infections. The importance of NK cells is highlighted by the ability of mice lacking both T and B cells (severe combined immunodeficiency, SCID) to control some virus infections, and humans with NK cell defects are also susceptible to certain viruses (Table 14.1).
Table 14.1 Natural killer cells play an important role in controlling infections
Infections where NK cells have been shown to help control infection | |
---|---|
Human | Mouse |
Human cytomegalovirus (HCMV; human herpesvirus 5) | Mouse cytomegalovirus (MCMV) |
Vesicular stomatitits virus (VSV) | Herpes simplex virus |
Herpes simplex virus (HSV) | Vaccinia virus |
Human papilloma virus (HPV) | Influenza virus |
Phagocytosis
Phagocytes engulf, kill and digest would-be parasites
Perhaps the greatest danger to the would-be parasite is to be recognized by a phagocytic cell, engulfed, killed and digested (Fig. 14.3). A description of the various stages of phagocytosis is given in Chapter 9. Phagocytes (principally macrophages) are normally found in the tissues where invading microorganisms are more likely to be encountered. In addition, phagocytes present in the blood (principally the PMNs) can be rapidly recruited into the tissues when and where required. Only about 1% of the normal adult bone marrow reserve of 3 × 1012 PMNs is present in the blood at any one time, representing a turnover of about 1011 PMNs/day. Most macrophages remain within the tissues, and well under 1% of our phagocytes are present in the blood as monocytes. PMNs are short lived, but macrophages can live for many years (see below).
Intracellular killing by phagocytes
Phagocytes kill organisms using either an oxidative or a non-oxidative mechanism
The mechanisms by which phagocytes kill the organisms they ingest are traditionally divided into oxidative and non-oxidative, depending upon whether the cell consumes oxygen in the process. Respiration in PMNs is non-mitochondrial and anaerobic, and the burst of oxygen consumption, the so-called ‘respiratory burst’ (Fig. 14.4) that accompanies phagocytosis represents the generation of microbicidal reactive oxygen intermediates (ROIs).
Oxidative killing
Oxidative killing involves the use of ROIs
The importance of ROIs in bacterial killing was revealed by the discovery that PMNs from patients with chronic granulomatous disease (CGD) did not consume oxygen after phagocytosing staphylococci. Patients with CGD have one of three kinds of genetic defect in a PMN membrane enzyme system involving nicotinamide adenine dinucleotide phosphate (NADPH) oxidase, PHOX (see Ch. 30). The normal activity of this system is the progressive reduction of atmospheric oxygen to water with the production of ROIs such as the superoxide ion, hydrogen peroxide and free hydroxyl radicals, all of which can be extremely toxic to microorganisms (Table 14.2).
Table 14.2 Some organisms killed by reactive oxygen and nitrogen species
Bacteria | Fungi | Protozoa |
---|---|---|
Staphylococcus aureus | Candida albicans | Plasmodium |
E. coli | Aspergillus | Leishmania (nitric oxide) |
Serratia marcescens |
CGD patients are unable to kill staphylococci and certain other bacteria and fungi, which consequently cause deep chronic abscesses. They can, however, deal with catalase-negative bacteria such as pneumococci because these produce, and do not destroy, their own hydrogen peroxide in sufficient amounts to interact with the cell myeloperoxidase, producing the highly toxic hypochlorous acid. The defective PMNs from CGD patients can be readily identified in vitro by their failure to reduce the yellow dye nitroblue tetrazolium to a blue compound (the ‘NBT test’, see Ch. 31).
Non-oxidative killing
Non-oxidative killing involves the use of the phagocyte’s cytotoxic granules
Oxygen is not always available for killing microorganisms; indeed, some bacteria grow best in anaerobic conditions (e.g. the Clostridia of gas gangrene), and oxygen would in any case be in short supply in a deep tissue abscess or a TB granuloma. Phagocytic cells therefore contain a number of other cytotoxic molecules. The best studied are the proteins in the various PMN granules (Table 14.3), which act on the contents of the phagosome as the granules fuse with it. Note that the transient fall in pH accompanying the respiratory burst enhances the activity of the cationic microbicidal proteins and defensins. Neutrophil serine proteinases have homology to the cytotoxic granzymes released by cytotoxic T cells.
Table 14.3 Contents of polymorphonuclear leukocyte (PMN) and eosinophil granules
PMN and eosinophil granule contents | ||
---|---|---|
PMN | Eosinophil | |
Primary (azurophil) | Specific (heterophil) | Cationic |
Myeloperoxidase | Lysozyme | Peroxidase |
Acid hydrolases | Lactoferrin | Cationic proteins |
Cathepsins G, B, D | Alkaline phosphatase | ECP |
Defensins | NADPH oxidase | MBP |
BPI | Collagenase | Neurotoxin |
Cationic proteins | Histaminase | Lysophospholipase |
Lysozyme |
BPI, bactericidal permeability increasing protein; ECP, eosinophil cationic protein; MBP, major basic protein; NADPH, nicotinamide adenine dinucleotide phosphate.
Another phagocytic cell, the eosinophil, is particularly rich in cytotoxic granules (Table 14.3). The highly cationic (i.e. basic) contents of these granules give them their characteristic acidophilic staining pattern. Five distinct eosinophil cationic proteins are known and seem to be particularly toxic to parasitic worms, at least in vitro. Because of the enormous difference in size between parasitic worms and eosinophils, this type of damage is limited to the outer surfaces of the parasite. The eosinophilia typical of worm infections is presumably an attempt to cope with these large and almost indestructible parasites. Both the production and level of activity of eosinophils is regulated by T cells and macrophages and mediated by cytokines such as interleukin 5 (IL-5) and tumour necrosis factor alpha (TNFα).
Monocytes and macrophages also contain cytotoxic granules. Unlike PMNs (Table 14.4), macrophages contain little or no myeloperoxidase, but secrete large amounts of lysozyme. Lysozyme is an antibacterial molecule maintained at a concentration of about 30 mg/mL in serum, though this concentration can increase to as high as 800 mg/mL in rare cases of monocytic leukaemia. Macrophages are extremely sensitive to activation by bacterial products (e.g. LPS) and T-cell products, e.g. IFNγ. Activated macrophages have a greatly enhanced ability to kill both intracellular and extracellular targets.
Table 14.4 The major phagocytic cells – PMNs and macrophages – differ in a number of important respects
Polymorphonuclear leukocytes and macrophages compared | ||
---|---|---|
PMN | Macrophage | |
Site of production | Bone marrow | Bone marrow |
Duration in marrow | 14 days | 54 h |
Duration in blood | 7–10 h | 20–40 h (monocyte) |
Average life span | 4 days | Months–years |
Numbers in blood | (2.5–7.5) × 109/L | (0.2–0.8) × 109/L |
Marrow reserve | 10 × blood | |
Numbers in tissues | (Transient) | 100 × blood |
Principal killing mechanisms | Oxidative, non-oxidative | Oxidative, nitric oxide, cytokines |
Activated by | TNFα, IFNγ, GM-CSF, microbial products | TNFα, IFNγ, GM-CSF, microbial products (e.g. LPS) |
Important deficiencies | CGD Myeloperoxidase Chemotactic Chediak–Higashi | Lipid storage diseases |
Major secretory products | Lysozyme | Over 80, including: lysozyme, cytokines (TNFα, IL-1), complement factors |
CGD, chronic granulomatous disease; GM-CSF, granulocyte-macrophage colony-stimulating factor; IFN, interferon; IL, interleukin; LPS, lipopolysaccharide; TNFα, tumour necrosis factor alpha.
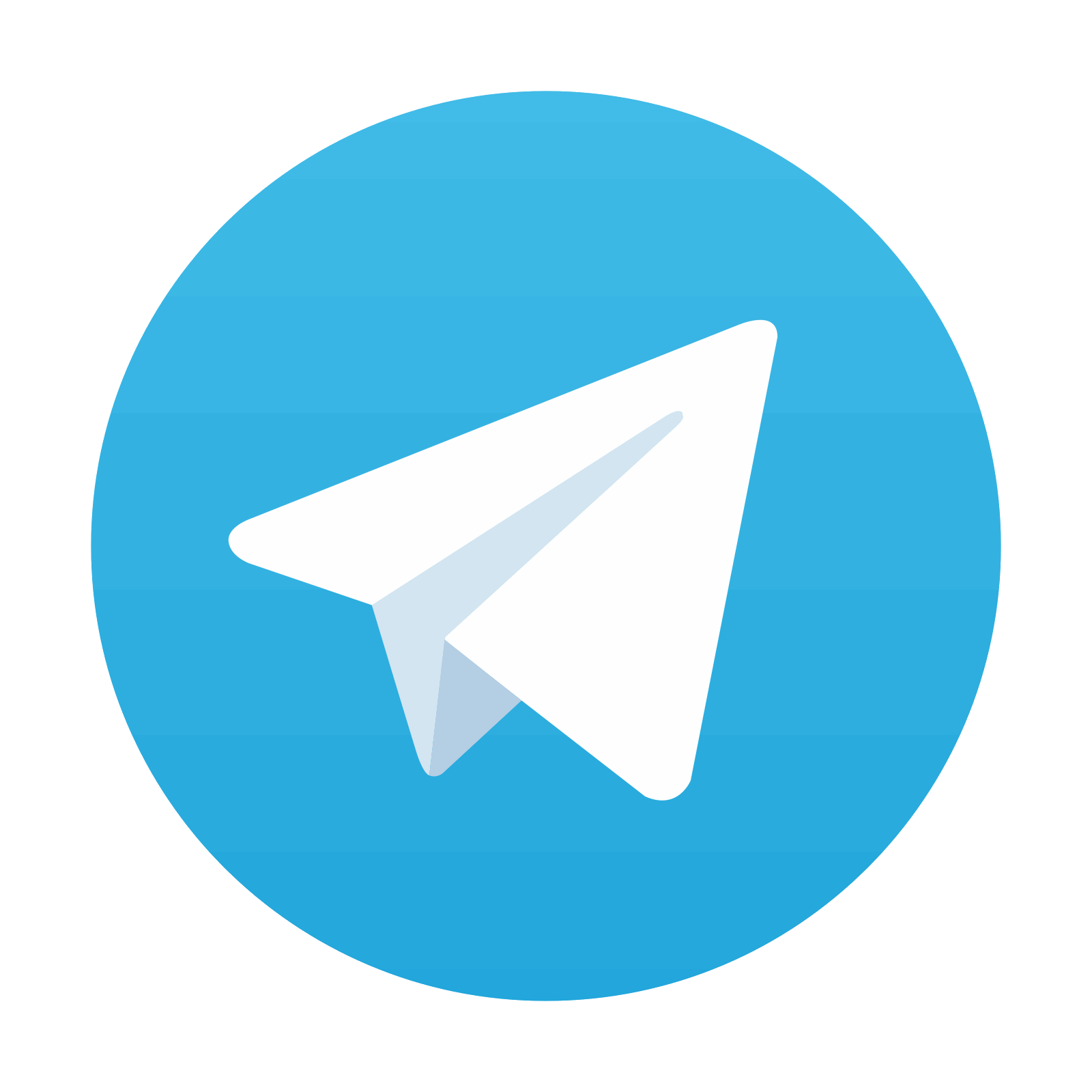
Stay updated, free articles. Join our Telegram channel

Full access? Get Clinical Tree
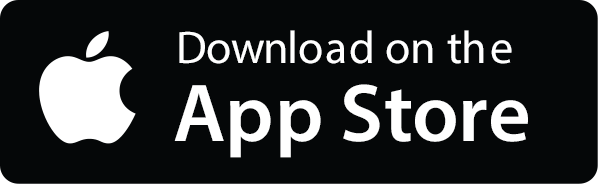
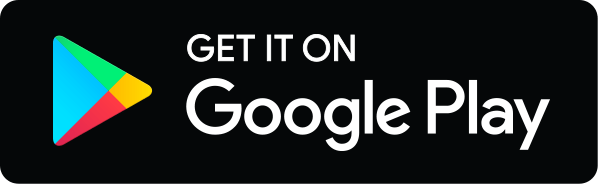