Figure 3.1PCcaps overall length and dimensions showing locking ring. (Courtesy of Capsugel.)
Shen and Zhong [5] studied the bioavailability of atorvastatin in beagle dogs using SMEDDS consisting excipients such as Labrafil and Cremophor RH40. The SMEDDS formulation was encapsulated in hard gelatin capsules. The authors compared the bioavailability (BA) data in comparison to the conventional tablet equivalent.
They reported higher BA in the encapsulated formulation. In an earlier study, Serajuddin et al. [6] investigated the dissolution and bioavailability of a poorly water-soluble drug (REV-5901) in different polyethylene glycols and in Gelucire 44/14 filled in hard gelatin capsule. Sherif et al. [7] also used hard gelatin capsules to contain a coprecipitate of danazol–hydroxypropyl-β-cyclodextrin for the purpose of studying the bioavailability of the poorly water-soluble danazol in beagle dogs. The oral absorption was reported to be rapid in the dogs and comparable to oral dosing in rats.
Clinical Phase
The use of hard shell capsules for developing “first” clinical batch formulation has increased within the last 15 years owing to the timelines that have to be met in the process of clinical batch manufacturing [8]. In addition, encapsulation as a technology is relatively simple and cost-effective in blinding investigators and clinical subjects compared to other dosage forms that could be used such as tablets, pellets, semisolids, liquids, and parenterals. The goal during early phase (Phase 0 or Phase I) is to assess safety of the molecule and proof of concept for the intended therapy. Limited quantity of active pharmaceutical ingredient (API) is available and the decision is often skewed toward use of capsule dosage form. The clinical supply capsule units can range from several hundreds to several thousands.
Realizing that more than 90% of drugs at the drug discovery and development stage do not make it to the market, it behooves the early preformulation stage scientist to use appropriate dosage form for the safety and effectiveness assessment. Despite the fact that the physicochemical properties (e.g., dissociation constant, partition coefficient, solution-state stability, solubility, polymorphism, thermal events) of a potential drug candidate might have been identified, there is no assurance that including excipients at this stage with the drug in tablet dosage form will result in a promising formulation; therefore, a provisional formulation, such as hard gelatin capsules containing only the drug, is often used at this early phase [9]. Several types of shells made of different materials are now being used for early phase clinical trials. As will be discussed in more detail later in the chapter, these include materials such as hard gelatin, soft gelatin, hydroxypropyl methylcellulose (hypromellose) or HPMC [10] starch [11], calcium pectinate [12], chitosan [13], and various polymer-coated capsules intended for delivery in the lower part of the intestine and the colon [14,15].
Based on the preclinical and clinical trial phase considerations in early phase development, capsule engineering has evolved to accommodate different types of capsule fabrications and technologies, and this will be discussed in the chapter. The manufacturing of hard shells for clinical trials will also be considered for the different capsule technologies such as miniaturization, overencapsulation, and liquid- or semisolid-filled hard shells. In particular, the manufacturing variables that could affect the delivery of the drug in preclinical and clinical trials will be discussed. The challenges associated with choice are usually related to manufacturability, cost–benefit ratio, and therapeutic outcomes. The chapter will underscore the uniqueness of hard shells in polyherbal formulation in clinical trials and in commercially available products. The functionality of hard shells in targeted oral delivery of small molecules and peptides/proteins in chronotherapy for drugs that follow circadian rhythm is emphasized. References will be made to drugs that otherwise could not be delivered orally because of the poor water solubility and which are delivered in lipid-based formulations using hard shells.
Irrespective of the capsule type, clinical development goal, and targeted delivery outcomes, the hard shell formulation development and subsequent use in preclinical or clinical trial should be approached from the quality of the product attributes or design of the formulation (i.e., quality-by-design [QbD] approach). This will eventually determine the quality of in vivo performance. Thus, QbD, relative to hard shells and clinical trials will be examined. Knowing the inevitability of regulatory oversight in product development, approval, and registration, perspectives of in vitro dissolution and respective in vivo performance and regulatory concerns regarding the choice of hard shell in the oral delivery of drugs over tablets will be discussed.
Miniaturization Technology
Miniaturization is a new concept in pharmaceutical technology of solid dosage forms that involves the use of smaller quantities of new chemical entities (NCEs). This is to allow for essential information at the preformulation stage that would expedite the potential formulation development to be gathered. Formulations could be delivered earlier using smaller, and more readily available APIs and all clinical studies can be supported with more realistic and representative dosage forms [16]. Aside from this, a go–or–no-go decision could be made earlier regarding the formulation process, thus saving precious development time and improving efficiency. This is unlike the traditional formulation method where relatively large amount of API is typically needed and which may not be available until late Phase I or early Phase II. Knowing that the NCE is available only for clinical demands, the development team can become very creative in using precision dosing technologies available as part of miniaturization.
Xcelodose Technology
It incorporates the miniaturization concept, and one of these technologies is Meridica’s non-excipient filling Xcelodose. It allows for the use of very little drug (100 μg to 100 mg) filled into a hard gelatin capsule for early preformulation or formulation studies in early clinical trials. It is also called powder in capsule (PIC), API in capsule, or chemical in capsule. It has been reported that 9 out of the top 10 pharmaceutical companies use Xcelodose [17], and it reduces time to Phase I significantly [18]. The technology makes go–no-go decisions earlier without too much overhead that usually comes with the conventional program. This is because technical problems or delays that otherwise could arise as a result of preformulation studies, such as drug-excipient compatibility, and formulation and analytical development are avoided. It allows the API to be screened according to the Critical Path Initiative strategy of the US Food and Drug Administration (FDA) that allows a drug to fail quickly without incurring too much costs and time [19]. Xcelodose is particularly suited for oral and inhalation delivery. The goal of this pharmaceutical technology should be to produce safe and quality formulations that can be achieved by built-in robust science via design of experiment (DOE).
Knowing that 0.02% of NCEs will reach the market, typically 10–15 years after discovery, and that it costs at least $500 million to successfully develop an approved molecule, the concept of miniaturization becomes very logical. It will expedite rapid selection of drug candidates and development of lead compounds [20]. As companies strive to accelerate time to market, the paradigm increases the chances of taking a market advantage, period of market exclusivity, and greater yield of revenues [16].
One of the challenges of miniaturization is use of machines (capsule filling machines) that can provide adequate information with the use of not more than 10 g. The machines should be capable of producing capsules that are equivalent to those produced by large-scale machines. The machines used in miniaturization technology need not be based on geometric similarity; however, the instrumentation should be such that equivalent products will be produced. The equipment will be discussed in “Clinical Batch Manufacturing or Fabrication of Hard Gelatin Capsules” section. Other disadvantages of the Xcelodose technology include the following: poorly water-soluble drugs (Classes II and IV Biopharmaceutics Classification System [BCS] drugs) will not be suited for the technology [21]; high-dose and low-bulk density drugs will not be suitable for the filling into capsules [22]; APIs that are micronized and susceptible to electrostatic charge, agglomeration, and moisture may also create a problem during filling; the technology may be limited to only Phase I clinical trials; and API incompatible with hard gelatin will be excluded.
It is inevitable that the technology will be well used as a twenty-first century-adopted formulation development approach especially considering the genomic-driven revolution in drug discovery that calls for elucidation of 4000 new drug targets within the next 10 years. As reported by the author, miniaturization will need to be combined with high-throughput screening and combinatorial chemistry in order to achieve rational lead information [16].
Capsule Design and Specialty Shells
Hard Gelatin Capsules PCcaps
The fabrication of PCcaps is based on easy oral dosing in preclinical trials. The capsules have locked rings for improved closure (Figure 3.2), and it is available in a kit that contains a stainless steel tamper for filling powder with poor flow properties (Figure 3.3). The volume as stated earlier is 21.8 μL or 0.02 mL. The capacity in weight, which varies from 12 to 24 mg, depends on the bulk density of the powder that also varies from 0.6 to 1.2, respectively.

Figure 3.2PCcaps with locking rings. (Courtesy of Capsugel.)

Figure 3.3(See color insert.) PCcaps for a preclinical trial study. (Courtesy of Capsugel.)
Coni-Snap Hard Gelatin Capsules
The standard Coni-Snap hard gelatin capsules are widely used for clinical trials or for overencapsulation of double-blind clinical trials. In these cases, size 0 and size 00 capsules are often used, and in some instances, the larger size 000 capsules are used. However, the Coni-Snap capsules might not always provide the features that are needed for the broad variety of clinical trial goals and for comparison to other solid dosage forms. To overcome these limitations for certain trials, specific double-blind capsules (DBcaps) are now available.
Hard Gelatin DBcaps
DBcaps are two-piece hard gelatin capsules designed for double-blind clinical trials in which the capsule body is completely covered by the elongated design of the cap. The design is such that it is virtually impossible to open the capsule without causing clearly visible damage, thus alerting investigators of blind breaking and bias. The importance of blinding is underscored in clinical trials involving antipsychotic drugs where patients may not want to participate in placebo-controlled clinical trials [23]. The refusal can be attributed to fear of deterioration of disease state if a placebo is given. Therefore, use of a capsule such as DBcaps provides the added security of blinding that cannot be easily broken.
There are eight different sizes of DBcaps—AAA, AAel, AA, A, B, C, D, and E (Table 3.1). The wider diameter of DBcaps offers an advantage of relative ease of containment of several shapes and sizes of tablets, while the shorter length facilitates ease of swallowing. More than 90% of most commonly tableted drugs can be overencapsulated using DBcaps. The sizing guide of DBcaps for overencapsulation of Coni-Snap capsules, tablets, and caplets of different shapes are shown in the table.

Source: Courtesy of Capsugel.
DBcaps allow direct filling of whole tablets of various shapes without a need for breaking or grinding of tablets, thus eliminating the concern for inaccurate dosage or modification of intended performance of drug (Figure 3.4). When the standard two-piece hard gelatin capsules (Coni-Snap) are used for blinding tablets for clinical trials, the smaller diameter (compared to DBcaps) limits the size of the tablet that can be filled into the capsule.

Figure 3.4(See color insert.) DBcaps showing dual locking rings and extended cap length. (a) Extended cap length of DBcaps capsules that completely covers the sidewall of the capsule body. This makes it virtually impossible to open the capsule without causing visible damage. A dual locking ring design provides a full-circumference, leak-free closure. (b) DBcaps capsules formulated in a wide array of colors. The five standard color formulations were developed based on globally accepted colorants and on sufficient opacity to ensure blinding. (Courtesy of Capsugel.)
Hypromellose (HPMC) Capsules
The use of HPMC capsules commonly called VCaps has gained attention in recent years in over-the-counter formulations, especially in the encapsulation of herbs and dietary supplements (Figure 3.5). The reason is that it is preferred over bovine-derived material like gelatin to rule out the possibility of transmission of bovine spongiform encephalopathy and spongiform encephalopathy.

Figure 3.5Hypromellose capsules (VCaps). (Courtesy of Capsugel.)
Preference for HPMC has also been attributed to the lack of cross-linking potential and drug–shell interaction that the gelatin capsule has shown [24]. Because of its vegetable origin, some cultural and religious preferences have become part of the reasons for the increased use of HPMC. However, because HPMC is bioadhesive, esophageal sticking tendency exists. This has been widely reported in literature.
Perkins et al. [25] reported this observation in a study involving elderly healthy subjects and use of radiolabeled capsules and tablets for comparison. Longer residence time (20.9 s) was observed in the HPMC capsule compared to tablet dosage form with a residence time of 4.3 s. Other investigators such as Cole et al. [26], McCargar et al. [27], and Osmanoglou et al. [28] also made similar observations. Hypromellose capsules are available in physical dimensions and shell weights similar to hard gelatin capsules, although the latter have wider size ranges.
HPMC capsules have been recommended for use in unit dose inhalers over hard gelatin capsules because of the higher moisture content of the latter (13–16%) compared to HPMC capsules with 4–6% moisture content. The higher moisture content of the gelatin capsules can cause interaction with the powdered material, resulting in the adherence of the powder to the capsule wall, inadequate discharge of powder, and possible dose failing [24]. Being a bioadhesive material, HPMC capsules are expected to stick to the esophagus compared to gelatin capsules, slowing down the transit time. As referred to earlier, Perkins et al. studied the esophageal transit time of HPMC capsules versus gelatin capsules [25]. The authors observed that the HPMC detached more easily compared to gelatin. Based on the nature of the two materials, it has been recommended that at least 150–200 mL of water be used when taking either capsule with the patient in an upright position that is maintained for several minutes [29]. An earlier study had indicated that there was tendency for HPMC capsules to stick to isolated porcine esophagus. However, it has been shown by gamma scintigraphy that the esophageal transit in the porcine model does not correlate with the human model [27].
In another study by Honkanen et al. [30], where six human subjects were used, the disintegration of two prolonged-release, radiolabeled formulations containing different grades of HPMC (K100 and K4M) in size 0 capsules was monitored. The disintegration time was measured at the midpoint of the time interval between the last image of the capsule with clear outlines and visually detectable spreading of the radioactivity plus the time of first detection of spreading radiation. It was observed that 4 out of the 12 capsules were lodged in the esophagus for 22–43 min. The initial disintegration time range was from 33 to 75 min, with very little difference between the two polymers at the 5% level. The profiles are shown in Figure 3.6.

Figure 3.6In vivo initial disintegration time (minutes) for the HPMC capsules in six healthy volunteers filled with two different prolonged formulations containing different viscosity grades of HPMC powder (HPMC K100 and HPMC K4M). (From www.oclc.org.)
Tuleu et al. [31] also compared the in vivo disintegration of uncoated 4-aminosalicylic acid Na (550 mg) HPMC capsules (that included carrageenan as gelling agent) with hard gelatin capsules in fasting eight healthy male volunteers. The two capsules were radiolabeled with indium 111 and technetium-99m and filled with a plug of lactose formulation. The capsule was administered to the subjects with 180 mL of water. The disintegration time was recorded when the scintigraphic image first shows the spread of radioactivity from the “core” of the capsule. It was observed that the disintegration times for both capsules are not significantly different.
Comparison of HPMC and Hard Gelatin Encapsulation and Performance
Unlike hard gelatin capsules, there was low correlation between the in vitro dissolution/disintegration and in vivo performance of HPMC capsules. Cole et al. [26] reported this in their investigation where ibuprofen-filled formulations in hard gelatin and HPMC capsules (containing gellan gum for gelling [HPMCgell]) were used. The study was a crossover experiment involving 11 subjects in fasted and fed states. Scintigraphic and pharmacokinetics parameters were used to evaluate the disintegration and extent of drug distribution (AUC).
The authors reported significant differences in the time before absorption was detected (Tlag) for the two capsules whether in fasted or fed state due to the delayed initial disintegration. However, the study revealed longer in vivo opening of the HPMCgell capsules compared to hard gelatin capsules. This was attributed to the gelling agent such as carrageenan and gellan gum that are usually used in HPMC capsule formulation. The cations such as potassium used in the gelling system of HPMC capsule and which are present in the in vivo media interacted with the HPMC, causing persistent gelling of the capsule shells. In vivo disintegration/dissolution of HPMCgell capsules has been reported to also decrease in acidic medium [24]. Avoiding the gelling agent may reduce the delay in disintegration/dissolution.
In addition, unlike hard gelatin capsules, the disintegration/dissolution of HPMC decreases with an increase in temperature; therefore, it has been suggested that hard gelatin capsules should be administered with a warm drink (>30°C), while HPMC capsules, which is soluble below 30°C, could be taken with cold water. The disintegration/dissolution and machinability of HPMC capsules have been their major drawbacks, while their main advantage is the preference based on cultural or religious reasons. Manufacturing of HPMC is more expensive compared to hard gelatin because of needed modification in the processing and accommodation of the gelling system that will need to be added. Other advantages of HPMC capsules are the better compatibility with hygroscopic materials based on its lower moisture content and the non-cross-linking tendency compared to hard gelatin capsules [24].
In a recent report by Cade [32], a newer type of HPMC capsules was developed (VCaps Plus) without the gelling agent using a proprietary thermal gelling process. The impact of the mold pin temperature and the conditions to achieve optimal capsule drying were assessed. The author reported that capsules that had no delay in dissolution/disintegration were produced because of the absence of cations in the gelling system that otherwise would have interacted with the in vivo media and slowed down the dissolution/disintegration.
Plantcaps
It is a plant-based capsule made from pullulan—naturally fermented from tapioca. Its vegetable-origin, preservative-free, gluten-free, starch-free, allergen-free, and non-GMO identity makes it very attractive for customers who want purely organic supplement and those with discerning healthy lifestyle. The capsule has GRAS (generally recognized as safe) status, Kosher and Halal certifications, and approval by the Vegetarian Society [33]. Its vegetable origin makes it suitable for Asian regulatory agencies especially for use in Japan. Pullulan offers the best oxygen barrier of all available plant-based products and is therefore suitable for encapsulation of vitamin C known to produce “spotting” associated with encapsulation using gelatin capsule. It is also suitable for masking pungent tastes and odors and will find suitability in encapsulation of pungent herbal or polyherbal products. Plantcaps can be easily manufactured using the regular capsule filling machines.
Starch Capsules
Starch capsules may be an alternative to hard gelatin or HPMC capsules if there is a need for such choice. The capsule is made from potato starch using injection molding. For filling and sealing, modification of the existing capsule manufacturing process is needed because the body and cap are manufactured separately. A benchtop, a semiautomatic, and an automatic machine can be used for the filling and sealing of starch capsules [11]. The capsules are manufactured in different sizes—0, 1, 2, 3, and 4. An advantage of starch capsules over other hard shells is the fact that the same size cap is made to fit all the different sizes. The cap fits evenly in place over the body, resulting in a good surface finish unlike the “lipped” seal on gelatin capsules.
The moisture content of starch capsules is comparable to that of hard gelatin capsules, although the moisture may be more tightly bound to the starch, thus reducing migration of moisture to the product. In an unpublished data, West Pharmaceuticals studied the stability of acetaminophen starch capsules in blister packages and reported acceptable stability after storage for 18 months. It would have been more informative if the blister packaging was compared to bulk packaging in a bottle.
Specialty Shells Design for Targeted Drug Delivery
PIC Technology
As referred to in “Miniaturization Technology” section, the Xcelodose miniaturization technology has gained a lot of attention in recent years for the filling of small amounts of drugs (usually without excipients) into capsule (PIC) for Phase I clinical trials. Some advantages and disadvantages of the technology have been highlighted in the section earlier. The emphases that could further be made of PIC are the contradictions of the technology. Although PIC could expedite Phase I clinical trials (3–6 months faster) compared to the conventional method, it could add to overall clinical and development cost since development work usually goes beyond Phase I trials. Therefore, the needed development work that should go into Phase II clinical trials is essentially postponed, thus slowing down New Drug Application (NDA) filing, approval, and overall development time and cost [21,22]. Pharmacokinetic data gathered using PIC technology for poorly soluble drugs could also be misleading, causing manufacturers to immaturely terminate the development without robust consideration of other means of improving the dissolution and absorption characteristics [21]. Another issue with PIC technology is the absence of the QbD requirements in the FDA guidance. More on this will be discussed in “Elements of QbD in Development of Capsules for Clinical Trial” section.
Impermeable or Coated Hard Gelatin Encapsulation and Pulsatile Delivery
The use of impermeable or coated hard gelatin capsules has increased in recent years as investigators develop oral dosage forms that can deliver new drugs and new vaccines or target the colon specifically during preclinical and clinical phases.
A comparison of the coated hard gelatin and HPMC capsules was made by Dvorakova et al. [15], where they used different levels of Eudragit L and S 12.5, respectively, to target the intestine and ileum. Bhat et al. [34] devised a hard gelatin in which the capsule body was rendered impermeable without the cap or the plug by cross-linking via exposure to formaldehyde plus potassium permanganate (to facilitate generation of formalin vapor). The cross-linking was processed in a dessicator. The meltable erodible plug was made in a mold using several polymers such as Xanthan gum, Karaya gum, and Veegum, and processed by direct compression. The plug was placed by hand on the content inside the capsule body followed by the replacement of the cap. The whole capsule assembly was then dip-coated using 5% ethanolic solution of ethyl cellulose. The goal was to achieve a pulsatile drug delivery that can be used for nocturnal asthma. The authors reported that the drug release was dependent on the polymer level and the lag time before pulsatile release was determined by the properties of the plug. They also observed programmable pulsatile delivery that varied between 2 and 36 h as expected with chronopharmaceutical drug delivery. More of the release mechanism will be discussed later in the chapter.
Capsular systems are more independent of the capsule content and more dependent on the capsule device. Pulsincap system, as an example, is an osmotic system that has an impermeable or insoluble capsule body and a swellable plug [35,36]. The impermeable capsule half is filled with the drug formulation while the capsule half (the cap) is closed at the open end with a swellable hydrogel plug. The drug is released rapidly once the plug swells and is ejected when in contact with dissolution media or gastrointestinal fluids [37,38].
Fused and Banded Hard Gelatin Capsules
In an invention reported by Samantaray et al. [39], fused hard gelatin capsule was designed to deliver a liquid formulation of paricalcitol (synthesized form of calcitriol, an active metabolite of vitamin D) for the prevention and treatment of hyperparathyroidism in chronic kidney disease. The formulation contains solubilizers such as Cremophor ELP and other pharmaceutically acceptable excipients. The capsules are filled and then sealed by spraying a water–ethanol mixture at the cap and body interface, followed by warming to fuse the cap and body together. Banding is done by rectifying the capsules and passing over a serrated revolving wheel that revolves in a gelatin bath. The gelatin that is picked up by the wheel is applied to the cap and body interface. A two-way crossover single-dose comparative bioavailability study of the liquid-filled fused capsules and commercially available tablets (Zemplar) of the drug showed similar pharmacokinetic profiles.
Acid-Resistant HPMC Capsules
Acid-resistant DRCaps have been developed to meet the challenges often encountered in manufacturing [40]. The goal is targeted delivery of drugs to the intestine. Acid-sensitive drugs and drugs/excipients that may be prone to acid-reflux will qualify for encapsulation using DRCaps. Because of its low moisture content, DRCaps will be useful for moisture-sensitive drugs and excipients. Unlike enteric-coated HPMC capsules, DRCaps development does not include a laborious coating process that may include solvents and heat that could compromise the stability of the drugs and excipients. The capsule is fully compatible with Capsugel’s Xcelodose S precision powder micro-dosing system.
Gastro-Resistant (Enteric-Coated) HPMC Capsules
Enteric-coated capsules have been used in a double-blind, placebo-controlled clinical trial in children (5–16 years) with Crohn’s disease [41]. In the study, one group received 5-aminosalicylic acid (5-ASA) + ω-3 fatty acids as triglycerides in gastroresistant capsules and 3 g/day Triolip capsule containing 400 mg eicosapentaenoic acid and 200 mg docosahexaenoic acid. The other group received 5-ASA + olive placebo capsules. The authors reported that the enteric-coated 5-ASA capsules in addition to the ω-3 fatty acids delayed the relapse of the disease even if it could not prevent it compared to the 5-ASA alone. The capsule material was not mentioned.
In a publication by Kadri and Greene, enteric-coated HPMC was developed using cellulose acetate phthalate (Aquacoat CPD) and polyvinyl acetate phthalate (Sureteric). The authors reported that the Aquacoat CPD-coated capsules were weaker at the cap/body junction than the Sureteric-coated capsules that remained rigid after 1 h. The testing was done in simulated gastric fluid although both provided similar gastric media protection at comparable coating levels [42]. If the coating is intended for colonic delivery, the pH solubility has to be chosen to accommodate the physiological condition. The pH solubilities for enteric delivery and colonic delivery are pH 5.5 and pH 7, respectively.
Examples of other polymers include cellulose trimellitiate, hydroxypropylmethyl cellulose phthalate, polyvinyl acetate phthalate, shellac copolymer of methacrylic acid and methylmethacrylate, azopolymers, disulfide polymers, and amylase [15]. Direct pan coating of HPMC capsule has been reported to be much easier than coating of gelatin when Eudragit L and S 12.5 were used because of insufficient surface adhesion to the smoother surface of gelatin and its relative brittleness [43].
Coated Hypromellose Capsules
Hydroxypropyl methylcellulose capsules containing 4-aminosalicylic acid was coated with amylose-ethylcellulose film coat by a dip coating process in an investigation. The coated capsules, monitored by scintigraphic imaging, remained intact throughout transit in the upper GIT before arrival in the colon. Plasma and urine concentrations of N-acetyl-4-aminosalicylic acid (a metabolite of 4-aminosalicylic acid) were used as primary endpoints in in-vivo evaluation monitoring of drug release in the healthy adults. Amylose/ethylcellulose coating film is called COLAL and prednisolone sodium metasulfobenzoate integrated into this system is designated COLAL-PRED [44,45].
In another related study, COLAL-PRED oral capsules were used in multicentered Phase III clinical trials involving 796 participants for the treatment of moderate acute ulcerative colitis. The 8-week randomized parallel study involved once-daily dosing of 40-, 60-, and 80-mg COLAL-PRED oral capsules, with prednisolone tablets as the active comparator. Colitis activity index and cortisol level were used as the endpoints of this safety and efficacy study. Although there were no differences in efficacy, the authors suggested that the safety profile of the test product was better than that of the comparator [46] COLAL-PRED has the potential to become the first bacteria-aided biomaterials and pH-sensitive polymeric film (BPSF)-coated delivery system approved for use in humans. Guar gum/Eudragit RS PO/S100 blend was used in coating hypromellose theophylline capsules using triethyl citrate as plasticizer and the dip coating method in another study [47]. The intent of the study was colon-delivery but there was no justification made in the report for colonic delivery of theophylline [14].
Enteric-Coated Starch Capsules
For drugs that may be destroyed by acidic medium in the gastric region or may cause gastric irritation or nausea, or targeted delivery, especially to the colon (for ulcerative colitis or Crohn’s disease therapy), starch capsules have been coated for delayed release for these purposes. The coating was reported in an unpublished report not to be problematic owing to the smoothness of the cap/body seal and the higher bulk density of the capsules. In a different study, Vilivalam et al. [48] evaluated the stability of 5-ASA starch capsules by monitoring the dissolution and disintegration indices at 25°C and 55% relative humidity (RH), and at 40°C and 75% RH. They reported that the to be 5-ASA release remains unchanged at the temperature and RH conditions.
Watts and Illum [49] studied the development of coated starch capsules for colon-targeted delivery and reported delayed release to meet the objective. To follow the transit characteristics of dosage forms through the GIT, scintigraphic study has been used in healthy human volunteers [50]. Doll et al. studied the release characteristics (capsule opening) and transit times of coated starch capsules and hard gelatin capsules though the GIT [51]. The GIT transit data were compared to plasma concentrations of the drug amoxicillin. In the study, coated starch or gelatin capsule containing 250 mg of the drug and a radiolabel was administered to the volunteers under fasted conditions. Analysis of the data showed no significant differences between the plasma concentrations and the transit time for both capsules.
In another study, Wilding et al. [52] studied the gastro-resistance of starch capsules enteric-coated using Aquateric coating. The authors observed capsule integrity in the stomach following administration after either an overnight fast or a medium breakfast, confirming the gastro-resistance of the capsules. Effect of food on the gastro-resistance was studied in another investigation [53] and the authors reported that the capsules remained intact for more than 2 h in 0.1 N hydrochloric acid but disintegrated within 20 min after the acid medium was exchanged for pH 6.8 phosphate buffer (simulated intestinal fluid pH). Gamma scintigraphy confirmed the gastro-resistance of the capsules in the stomach until disintegration occurred in the small intestine at 68 ± 28 and 95 ± 3.3 min following fasted and fed administrations, respectively, after leaving the stomach.
Targit Technology
It is an enteric-coated starch capsule designed for targeted delivery to different sites within the colonic region. Eudragit L and S were used for the coating to provide timed dissolving of the capsule as it enters the small intestine after leaving the stomach [54]. The coating is designed such that the capsule disintegrates at a predetermined region such as the terminal ileum and the ascending, the transverse, and the descending colon (Figure 3.7). Therefore, drug release from the Targit system is pH- and time-dependent, which is preferred or fail-safe compared to a system that is only dependent on pH change in the environment.

Figure 3.7(See color insert.) Scintigraphic images of a radiolabeled TARGIT capsule in a human after oral administration. At 0 h, the capsule is in the stomach (a); 1 h, small intestine (b); 3.5 h, ascending colon (c); and 8.5 h, dispersed in transverse and descending colon (d). (Courtesy of Taylor & Francis.)
Osmotic Capsules
Waterman et al. [55] developed osmotic capsule technology for the purpose of controlling the delivery of the drug based on the water permeability of the capsule shell and the ratio of the combination polymers used to compose the capsule. The osmotic capsule was made with the combination of a water-insoluble polymer (cellulose acetate) and a water-soluble polymer (polyethylene glycol 3350).
The intent of the capsule device was to rapidly develop a delivery system in the early development phase that could facilitate the understanding of the drug release profiles using needed additives but with minimal formulation optimization. The ultimate goal is to shorten the time to market. The osmotic capsule features include drug-independent drug release profiles, easily varied drug loading and simple fabrication without specialized equipment, simple formulation, and good in vitro–in vivo correlation. The device is made of a capsule body (which has a laser-drilled hole through the closed end), an active tablet, a push tablet, and a capsule cap.
Other osmotic capsular systems have been reported by several authors to provide a sustained drug release throughout the large bowel after a silent phase that coincides with the time necessary for their activation. In an investigation by Crison et al., osmotic excipients were added to the formulation in the capsule body to expedite the plug ejection at the end of the lag phase. In a capsule-like delivery system, an erodible plug made using the injection-molding technology was positioned at each open end of an impermeable shell cylinder that has the drug in the core [56]. The system can also be made such that an insoluble plug was matched with a semipermeable capsule body containing the active ingredient along with an osmotic charge (programmable oral release technology [PORT]) [57]. Upon inflow of aqueous fluid, hydrostatic pressure that develops inside the capsule pushes the plug out. The mechanism was used to create lag phases that could be suitable for time-dependent colonic delivery.
The osmotic pump mechanism was also exploited to achieve lag phases potentially suitable for time-based colonic delivery [58]. As stated in “Capsule Design and Specialty Shells” section, Zhang et al. reported a similar system in an earlier investigation [35].
Clinical Batch Manufacturing or Fabrication of Hard Gelatin Capsules
Miniaturization Technology Encapsulation
The miniaturization technology used in the Xcelodose system is a precision micro-dose automated encapsulator (Figure 3.8). It allows for direct filling of minute quantities of drug (100 μg to 100 mg) into hard gelatin capsules (PIC) without excipients at a rate of several hundred capsules per hour. This is particularly suited in clinical batch manufacturing or production of formulations for clinical trials.

Figure 3.8Xcelolab encapsulator. (Courtesy of Capsugel.)
The Xcelolab dispenser or encapsulator is used for Xcelodose technology [59]. The Xcelodose 120S system can be used to manually fill capsules and small vials, tubes, and cassettes. The programmable dispensing of dose weight through the weighing balance assembly ensures dose accuracy. It has a closed-loop dispensing mechanism into the capsules and for powder characterization, preparation, and stability setup. The encapsulation can be done in a regular laboratory, under a laminar flow setting containment enclosure. It uses a tapping mechanism that involves a building a micro-bridge (Figure 3.9) that upon impact breaks down and rebuild [60]. The technology ensures that the drug is not compacted and the stability is not compromised as a result of compaction. The technology is suitable for free-flowing actives, including cohesive substances and micronized or inhalation powders. The filling occurs without segregation, and for moisture-sensitive actives, operation can be done under humidity-controlled environment, for example, <5% RH.

Figure 3.9Xcelolab tapping and micro-bridging powder encapsulation. (Courtesy of Capsugel.)
The capacity for Xcelolab to fill drug alone makes analysis and stability testing easier and the development time faster. It also reduces waste of actives especially at the early development phase when limited amount of active is available.
Xcelodose 600 is an automated programmable 21 CFR Part 11 compliant machine (Figure 3.10) that is used for precise metering of API or simply blending of formulations (100 μg to 300 mg) into capsules of varied sizes (00 to 4) [61]. The powder is dispensed through dispensing heads (a and b) through dispensing fingers (c) for cohesive materials (Figure 3.11).

Figure 3.10Xcelodose 600 micro filling system. (Marketed by Capsugel BVBA.)

Figure 3.11(See color insert.) Xcelodose 600 micro filling system dispensing heads (a and b) and fingers (c). (Courtesy of Capsugel.)
Clinical trial (Phase I) supplies up to several thousand capsules can be manufactured using the automatic feeding high-throughput unit (HTU) (Figure 3.12) attached to Xcelodose.

Figure 3.12Xcelience automatic feeding high-throughput unit (HTU). (Courtesy of Capsugel.)
Liquid or Semisolid Filled Hard Gelatin Capsules
Hard gelatin capsules are used to contain liquids and semisolids during the early stages of drug development. This is applicable where the drug is poorly water-soluble or there is a problem of recrystallization if the formulation is developed as a solution form. At this stage, there is limited supply of the active. Therefore, producing small batches of formulations for clinical trials becomes critical. Manual filling can be done in the laboratory using a pipette or a semiautomatic machine. An example of a simple machine used for such clinical batch manufacturing is CFS 1200 that was developed by Capsugel. The machine can fill up to 1200 capsules/h with hot melt liquid or cold thixotropic semisolid/liquid materials. Liquid filling and sealing technologies for hard shell capsules provide the formulator with the flexibility of in-house development of formulations from a small scale, as required for Phase I studies, up to production [62]. If hard gelatin capsules cannot be used because of any formulation, preference, or cultural reason in early stage development, alternative materials such as polymer-based shells or hypromellose may be used.
Overencapsulation Hard Gelatin Capsules (DBcaps)
Overencapsulation is a process that hides a dosage form (tablets and capsules) in another capsule shell for the purpose of blinding in clinical trials. The DBcaps are available in eight different sizes to accommodate various sizes of comparator tablet, caplet, or capsule shapes in clinical trials blinding (Figure 3.13). Because there is no need for modification of comparator dosage forms, the process of blinding becomes easier and the errors that could be associated with the bioequivalence data become reduced.

Figure 3.13(See color insert.) Different DBcaps sizes. (Courtesy of Capsugel.)
The capsules can be filled manually (Profiller 3700), as shown in Figure 3.14, and with most semiautomatic and automatic capsule filling machines such as the ProFiller 3800 DB machine, CFS 1200, and Ultra 8 II combined with TFR 8 loading ring (Figures 3.15 and 3.16). The ProFiller 3800 DB machine is a good alternative to hand-filling small batches for Phase I and Phase II double-blind studies because it comes with a semiautomatic orienter. The machine is designed specifically for filling DBcaps. It orients, separates, and locks DBcaps capsules without vacuum or pneumatics. It can double blind 1000 to 1500 doses of caplets, tablets, or capsules per hour. A separate attachment is used for different capsule sizes.

Figure 3.14ProFiller 3700 and 3800 stainless steel upgrade series. (Courtesy of Capsugel.)

Figure 3.15Ultra III semiautomatic capsule. (Courtesy of Capsugel.)

Figure 3.16TFR 8 tablet filling ring filling machine. (Courtesy of Capsugel.)
Manufacturing Considerations of DBcaps and Clinical Trials
The intent to use DBcaps in clinical trials should be based on several factors such as capsule size, target population, the cultural acceptance of the capsule color, the influence of backfill on capsule filling, the capsule filling machine and change parts, and ordering of clinical supplies.
Target Population
Geriatric and pediatric populations cannot swallow bigger size capsules easily; therefore, in clinical trials, this factor has to be taken into consideration. When a smaller capsule size is needed for overencapsulation, the regular Coni-Snap sizes 1, 2, or 3 may be used for blinding.
Choice of Capsule Color
The choice of color is crucial in clinical trials, and DBcaps has the advantage of availability in many colors, which can be chosen to completely hide the encapsulated or enclosed unit (Figure 3.17). Examples of colors are shown below.

Figure 3.17(See color insert.) DBcaps in different colors. (Courtesy of Capsugel.)
The DBcaps should not reveal any shadow or air pockets resulting from the backfill or any printing or monogram on the encapsulated unit [63]. The choice of color dyes and pigments may sometimes be based on cultural acceptance. Regulatory agencies such as the World Health Organization, the US Code of Federal Regulations, and the Japanese Pharmaceutical Excipients Directory have set guidelines on ingestion of iron oxide and elemental iron. The reason is that iron oxides are commonly used in pharmaceutical grade colorants in DBcaps. Although several colors are accepted globally, many countries have restrictions on some colors. This must be confirmed before clinical investigation in order to dialogue with capsule vendor according in terms of ordering of the capsules in the right colors. A good lead time of 2–3 months should be built into the planning for ordering the capsules.
Backfill
The choice of backfill (excipient that can be added to the encapsulated unit) is sometimes determined by the excipients that are used for the formulation of the comparator product [64]. It is best to choose a similar excipient but it is not required. This is to avoid problems of excipient–excipient interaction that could result in compromise of disintegration or dissolution results. The information regarding excipients used in the comparator product is usually listed in the package insert or in Physician Desk Reference. The most commonly used excipients are microcrystalline cellulose or lactose monohydrate.
The preference for the two excipients is attributed to their hydrophilicity and water solubility. Microcrystalline cellulose is incompatible with strong oxidizing agents and is hygroscopic; it also has disintegrating properties depending on the amount in the formulation. Lactose monohydrate is incompatible with primary and some secondary amine drugs such as aminophylline and amphetamines due to browning that results from the Maillard reaction. Therefore, lactose should be avoided in lactose-intolerant populations especially if a larger amount is needed in the backfill.
Faust [64] studied the effect of variations in the excipients on disintegration and dissolution. Lactose anhydrous, which is sometimes used as backfill, may contain 5-(hydroxymethyl)-2 furfuraldehyde as an impurity that could cause cross-linking. Examples of other excipients that may form aldehyde upon autooxidation are starch, polysorbate 80, and polyethylene glycol. Therefore, drug or excipients should not contain aldehydes that could cross-link with the gelatin. It was reported that backfill with a high amount of microcrystalline cellulose pulled more water from the shell, thus causing faster disintegration of the shells compared to backfill made up of lactose monohydrate. However, mounding (coning of excipients under the dissolution paddle) occurred in the microcrystalline backfill that resulted in slower dissolution of the comparator product compared to lactose monohydrate. The author then concluded that a 50:50 blend of both excipients would counterbalance the effect of each and provide the expected outcomes in disintegration and dissolution of the comparator product. The blend also met the SUPAC-IR guidance for sameness/equivalence as compared to the comparator product.
Huynh-Ba and Aubry [65] reported that the dissolution profiles of two encapsulated forms of the same tablet with different overfill or backfill excipient gave significantly different results in dissolution (Figure 3.18). The difference could be attributed to the excipients, which the authors did not include in the report.

Figure 3.18Comparison of dissolution profiles of innovator’s tablet and two overencapsulated formulations. (From Huynh-Ba K, Aubry AF. Analytical development strategies for comparator products. American Pharmaceutical Review 2003; 6(2): 92–97.)
Use of DBcaps can significantly reduce the amount of backfill or overfill that will be used during manufacturing. Thus, the potential for drug–excipient or excipient–excipient interaction is reduced. For example, using a size C DBcaps instead of size 0 of the regular Coni-Snap reduces the volume for overfilling by 40% (Figure 3.19). The manufacturing considerations highlighted above and other formulation and process attributes can be used to design a formulation in a systematic manner in order to ensure quality and reduce risk in early phase development using the QbD approach that will be discussed later in the chapter.

Figure 3.19Size comparison of DBcaps capsules versus standard capsules. (From Huynh-Ba K, Aubry AF. Analytical development strategies for comparator products. American Pharmaceutical Review 2003; 6(2): 92–97.)
Dissolution of DBcaps Capsules
The dissolution of drug from an overencapsulated product is dependent on the drug BCS, the characteristics of the product (e.g., tablets, capsules, mini-tablets, pellets, etc.), and the nature of the backfill. It has been reported that the profiles of overencapsulated and un-encapsulated products are comparable enough not to affect the dissolution endpoint; however, there could be a lag time of 5–10 min as a result of the capsule opening.
Esseku et al. [8] studied the dissolution of DBcaps overencapsulated tablets of drugs that belonged to different BCS groups—propranolol, a highly soluble, highly permeable BCS class I, and rofecoxib, a low-solubility and high-permeability BCS class II drug that is susceptible to oxidation and photolysis [8]. The influence of moisture on the disintegration and dissolution of the encapsulated tablets has not been studied for either class of drugs. Two blocks of 2 × 2 × 2 randomized full factorial design were used to determine the effect of three independent parameters. Two levels, that is, two drug types (propranolol and rofecoxib), two capsule types (DBcaps and Coni-Snap), and two filler levels (microcrystalline cellulose/lactose 1:1 mixture and no filler), were used in the design. They reported that the disintegration time (D-time) for rofecoxib was slightly higher than the D-time for propranolol, possibly because of the lower solubility of the former. D-times for the capsule and filler effects were similar, ranging from 3.67 to 3.85 min, respectively, an indication that the variables had no effect on the D-times and were not statistically significant (P > 0.05).
To compare the dissolution profiles, the similarity factor F2 was used. For propranolol tablets, the F2 between CS and DBcaps was 60.67, indicating that the dissolution profiles of the two were similar. The similarity factor between rofecoxib tablets encapsulated in standard and DBcaps was 62.48. This was also indicative of similarity in dissolution profiles.
Encapsulated Polyherbal Formulations and Clinical Trials
Hard shell capsules have found great utility in nutraceuticals and polyherbals. HPMC capsules have particularly drawn interest from manufacturers of herbal formulations and nutraceuticals because of the non-bovine origin. Encapsulation using different hard shells in general is also preferred because of convenience of manufacturing. Examples of the products in the market that have sufficient information are shown in Table 3.2.
Product | Nature of the Formulation | Manufacturing Company |
---|---|---|
Damiana Herb 300 mg | Pure powdered herbs (Damiana turnera aphrodisiaca) | Bio-Health Ltd., UK |
Thera Veda’s Ajay-Allergy Support Formula | Vegetable extracts and powders | Organix South, USA |
Natren Life Start 2 | Bacteria, vitamin C, potato powders and whole goat milk | NATREN, Inc., USA |
Coloclear (in VegiCap) | Flax seeds, slippery elm and other herbs | Higher Nature Ltd., USA |
Jarro-Dophilus EPS | 8 probiotic species and ascorbic acid | Jarrow Formulas, USA |
Culturelle HS Capsules | 80 mg lactobacillus GG (L. rhamnosus GG) Vegetarian formula | Kirkman Labs, USA |
Align Daily Probiotic Supplement Capsules | Bifidobacterium infantis | Procter & Gamble, USA |
Sportlegs Supplement | Vitamin D, calcium and magnesium | Sportlegs, USA |
Planetary Herbals Cinnamon Extract | Cinnamomum aromacticum 300 mg, bark extract 10:1 yielding 8% flavonoids, cinnamomum aromaticum bark 100 mg | Planetary Herbals, USA |
Ex-Tox II | Folic acid, cilantro powder (leaf), ethylenediamine tetra-acetic acid, N-acetyl L-cysteine, fulvic (humic) acid, R-lipoic acid (K-RALA), L-methionine | Progressive Labs, USA |
Source: Al-Tabakha MM, HPMC capsules: Current status and future prospects. J Pharm Pharm Sci 2010; 13 (3): 428–442. Permission granted by the Canadian Society of Pharmaceutical Sciences.
Establishment of safety and efficacy of polyherbals has had some pitfalls in the past because of lack of adequate evaluation. Therefore, to overcome this, encapsulation has been used for the formulation and evaluation of polyherbals; Khan et al. [66] evaluated the physicochemical parameters including the preformulation characteristics of four anti-stress plants, namely, Withania somnifera, Tinospora cordifolia, Emblica officinalis, and Eugenia caryophyllus. Each plant was prepared after drying and milling by wet granulation in readiness for encapsulation into size 00 hard gelatin capsules. Among other tests conducted, high-performance thin-layer chromatography and differential scanning calorimetry indicated that the plants contain withanolide, berberine, gallic acid, and eugenol markers, respectively. Disintegration test of the capsules was performed using a digital microprocessor disintegration apparatus. The authors reported that the disintegration was 8 min 28 s.
Another pitfall in herbal product evaluation is lack of assessment of the effectiveness of the blind. Lack of blinding may lead to bias in performance assessment and in the choice of analytical strategies and methods. Based on this premise, Zick et al. [67] conducted a randomized placebo-controlled clinical trial to assess blinding with ginger (Zingiber officinale) using healthy individuals. The goal of the study is to establish whether the participants can determine accurately if they receive a ginger or placebo capsule in blister pack and a bottle filled with ginger or placebo capsules. The authors reported that volunteers couldn’t determine which type of individual capsule they receive but could distinguish a bottle with ginger capsules. This outcome of the research is that use of ginger capsules in blister packs enhanced blinding. They recommended that, for studies that include powdered standardized ginger root, one should place the ginger capsules in blister packs where the participants receive individual capsules and where the distinctive ginger aroma that otherwise is present in bulk packing of many capsules in a bottle is absent. The authors also recommended that, in general, clinical trials of herbal products include blinding procedures for the above-stated reasons.
Hard shell capsules were used in another clinical study where a comparative clinical evaluation of a polyherbal formulation with multivitamin formulation was undertaken for learning and memory enhancement [68]. In the randomized, placebo-controlled, double-blind study, the authors used 47 human volunteers (18–24 years) that were given either a capsule of the placebo or two capsules of the polyherbal formulation (500 mg) at night or one capsule of the multivitamin formulation (500 mg) two times a day for a period of 3 months. The subjects were monitored for neuropsychological tests such as short-term memory and IQ scores. They reported an increase in short-term memory and IQ scores in the polyherbal- and multivitamin-treated group, suggesting that both formulations improved learning and memory compared to the placebo in young healthy subjects. The polyherbal appeared more effective than the multivitamin formulation.
A clinical evaluation of herbal formulation was also reported by Tasawar et al. [69] where Nigella sativa was studied for the lowering of body total lipid concentration and prevention of atherosclerosis. The authors used 80 subjects (in fasted state) that were divided into two groups—an interventional group (given capsules of N. sativa and statin) and a non-interventional group that received statin capsule only. The study, which lasted 6 months, showed that there was a significant decrease in cholesterol, LDL, VLDL, and triglycerides and a significant increase in HDL in the interventional group compared to the non-interventional group that received only statin. This is an indication that N. sativa has the therapeutic effectiveness to reduce lipid profile in patients with coronary artery disease.
The pharmacokinetics and bioavailability of soy extract capsules were studied and compared with a soy beverage in a randomized two-phase crossover design. The two products have isoflavones—daidzein and genistein—but in different ratios. The results revealed that the normalized bioavailability of daidzein, following a single oral administration of soy beverage, was slightly (but significantly) less than that of soy extract capsules, whereas the bioavailability of genistein from both soy preparations was comparable. The other pharmacokinetic parameters of daidzein and genistein, including Cmax adjusted for the dose, Tmax, and t1/2, were similar for both soy preparations.
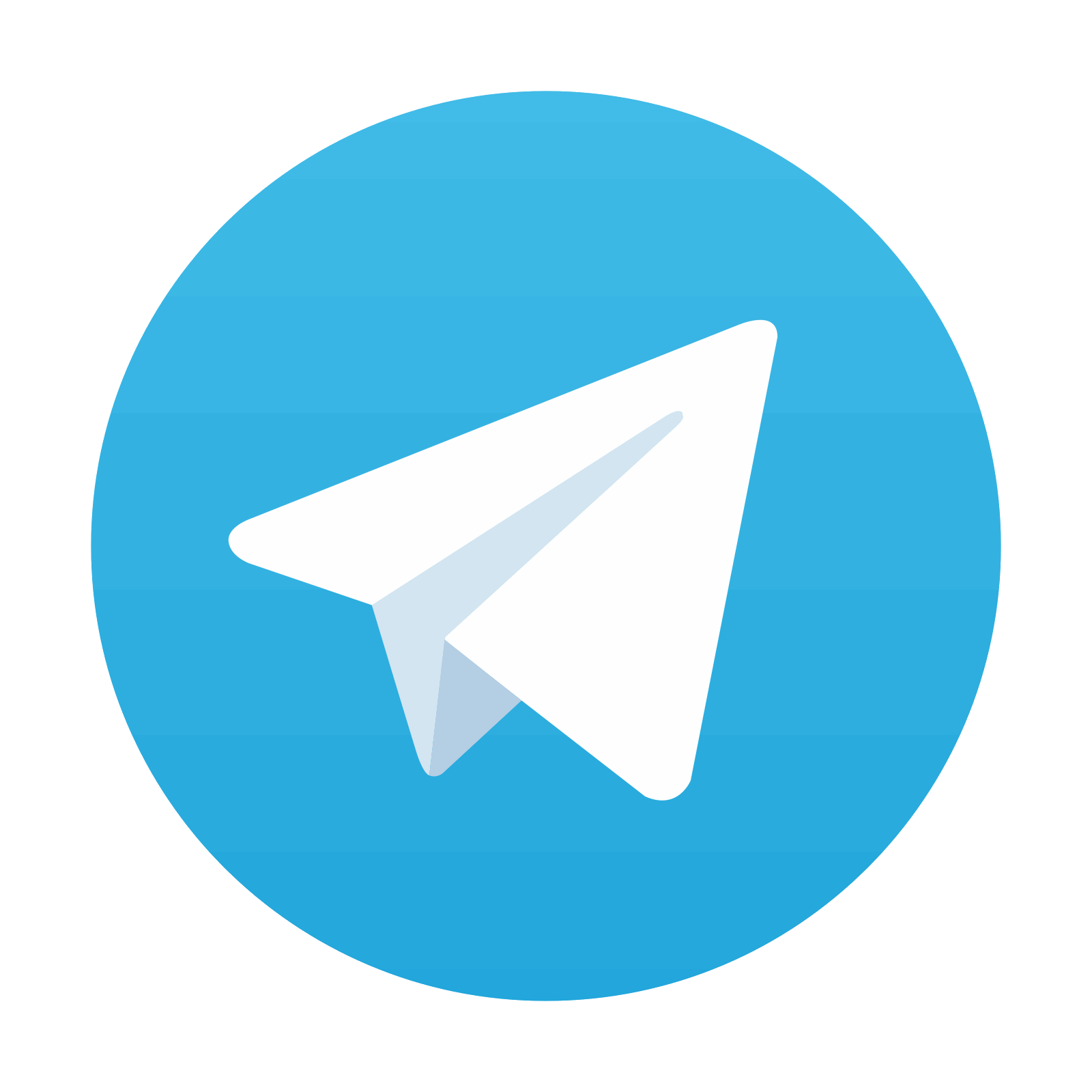
Stay updated, free articles. Join our Telegram channel

Full access? Get Clinical Tree
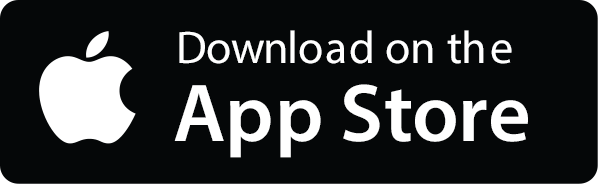
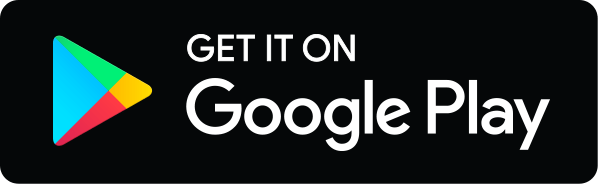