18 Genitourinary system
ANATOMY
KIDNEYS
Macroscopic anatomy
The renal vein, renal artery and renal pelvis enter and leave the kidney at the level of the hilum and are situated anatomically in relation to each other in the order mentioned above, moving in an anteroposterior direction. The anatomy of the contents of the hilum can be variable: for instance the renal pelvis can be bifid, and the artery and vein may split into branches or receive tributaries, respectively, to a variable extent at the hilum – which can, on occasion, lead to confusion at the time of surgical dissection in this area.
The hilum of the kidney opens into a space called the renal sinus which is surrounded by the kidney parenchyma and contains branches of the renal artery, major tributaries of the renal vein, and both the major and minor calyces (Fig. 18.1). All of the structures are surrounded and cushioned by fat.
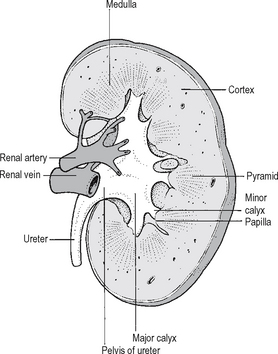
Fig. 18.1 The cut surface of the kidney.
Source: Rogers A W, Textbook of anatomy; Churchill Livingstone, Edinburgh (1992).
There are two to three major calyces in each kidney, each of which comprises a number of minor calyces. An aid to visualising the anatomy is provided by the linguistic derivation of the term ‘calyx’ (latin for a wine glass). There are as many minor calyces as there are papillae, usually six to ten per kidney. The renal parenchyma comprises two zones (Fig. 18.1):
The renal arteries
These are direct branches from the aorta to each kidney, and in 30% of cases accessory renal arteries are present. The renal artery divides into three to five segmental arteries at the hilum, which divide within the sinus into six to ten lobar arteries – one for each each pyramid and associated cortex (Fig. 18.2). Each lobar artery divides into six interlobar arteries which are associated with papillae. The interlobar arteries give rise to arcuate arteries which run in a plane between cortex and medulla. The arterial supply to the cortex is derived from interlobular arteries which are branches of the arcuate arteries directed radially towards the kidney capsule, and each of these in turn gives off the afferent arterioles and supply glomeruli. The efferent arterioles that leave the glomeruli supply the capillary bed that surrounds the convoluted tubules. Arteries enter and leave each pyramid at its base and, as they penetrate deeper towards the papilla, they lose water by osmosis and gain ions by diffusion – thereby reaching equilibrium with surrounding extracellular fluid. They then loop back towards the base of the pyramid, lose ions by diffusion and gain water by osmosis until they reach normal osmolarity at the base of the pyramid. These medullary vessels lie in bundles named vasa recta and are fed on the arterial side by efferent arterioles with adjacent glomeruli lying near the corticomedullary junction.
The renal veins
These follow the arterial pattern closely. In the sinus of the kidney, interlobar veins unite into lobar veins then into segmental veins which join together to form the renal vein at the hilum of the kidney. The renal veins empty directly into the vena cava. Since the vena cava lies on the right side of the abdomen, the left renal vein is longer than the right and usually passes anterior to the aorta just caudal to the origin of the superior mesenteric artery. The left renal vein receives tributaries from the adrenal gland and gonad before entering the IVC. On the right side the adrenal vein usually drains directly into the IVC.
Each kidney lies within a cushioning bed of fat and tissue. The kidney itself is surrounded by renal fascia. There is a layer of perirenal fat which lies between the renal fascia and the true capsule of the kidney, which is continuous at the hilum with the fat and the renal sinus. In addition there is a layer of perirenal fat lying outside of the perirenal fascia (Gerota’s fascia) which is particularly obvious posterior to the kidney (Fig. 18.3).
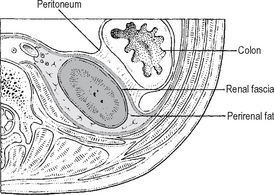
Fig. 18.3 Cross-section of the posterior part of the abdomen, showing the coverings of the kidney.
Source: Rogers op. cit.
The adrenal glands lie within a separate compartment of the renal fascia. The surface markings of the kidney are shown in Fig. 18.4. The hilum of both kidneys lie roughly at the level of L1 (the transpyloric plane).
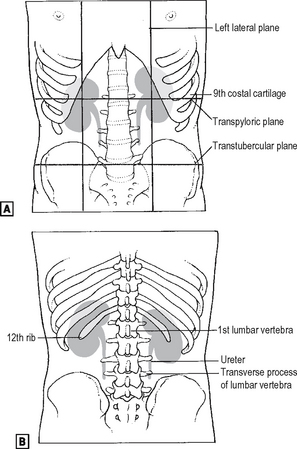
Fig. 18.4 The surface markings of the kidney. anterior view.
posterior view.
Source: Rogers op. cit.
In surgical practice it is very important to be aware of the anterior and posterior relationships of the kidneys – particularly in situations where normal tissue planes between the kidney and its adjacent structures are disordered due to either malignant or inflammatory disease processes, where it is important to be aware of which structures need to be mobilised (Figs. 18.5 and 18.6). The relationship of the kidneys to surface markings is also important in planning the surgical approach to the patient during any procedure.
URETER
The wall of the ureter is composed of smooth muscle with a rich innervation comprising both sympathetic and parasympathetic fibres. The sympathetic fibres arise from spinal segments T11–L1 and the parasympathetic fibres from sacral segments S2–S4. Most of the nerves to the ureters are sensory. Stretching the wall, for instance with the passage of a ureteric calculus, produces acute pain. In view of the segmental innervation of the ureter this pain is usually referred to T11–L1. In addition the pain may radiate down the front of the thigh to the area supplied by L2. The ureter is lined by transitional epithelium continuous with that of the renal pelvis and calyces. The transitional cell lining of the urinary tract deserves some comment because this is a highly specialised epithelial layer designed to prevent diffusion of urine and other solids out of the urine and conversely passage of water into the urine by osmosis. Despite this it must be borne in mind that the urothelium is not purely an inert barrier, and there are a number of active transport mechanisms which are present, particularly within the urothelium of the bladder, which facilitate the passage of many substances, including drugs, through the urothelial lining and hence into surrounding tissues and ultimately the blood stream.
Surgical injuries to the ureter are most common in its lower third, owing to the close proximity of the ureter to the blood supply of the uterus, where the ureter is easily damaged during hysterectomy. It is important to appreciate the relations of the ureters and in particular the close proximity of the ureter to the gonadal vessels (Fig. 18.7), particularly the gonadal vein and its lower abdominal course through the bony pelvis, since in this area it is not unknown for the gonadal vein to be mistaken for the ureter and indeed mobilised instead of the ureter! The anterior relations of the ureter are easily dealt with in the majority of circumstances, providing its retroperitoneal position is borne in mind (Fig. 18.8).
BLADDER
Macroscopic anatomy and innervation
The relations of the bladder are as follows:
The relationship of the bladder to adjacent structures in both the male and female is best appreciated on a sagittal view (Fig. 18.9).
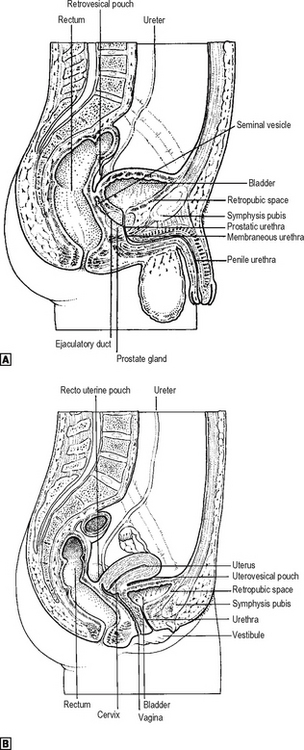
Fig. 18.9 the male pelvis in sagittal section.
the female pelvis in sagittal section.
Source: Rogers op. cit.
The bladder is a remarkable organ. As with the rest of the urinary tract it is lined by urothelium which acts as a watertight layer which nevertheless retains the ability to allow the active transport of a number of substances across its wall. The bladder should be considered to comprise two distinct functional and anatomical components. One is the trigone, which is triangular in structure and receives the two ureters at its uppermost lateral angles (Waldeyer’s sheath) and extends down to its apex at the internal urethral meatus, where the smooth muscle of the trigone is contiguous with a ridge of smooth muscle extending down the urethra to the urethral sphincter mechanism. The innervation of the trigone is distinct from that of the remainder of the bladder muscle (detrusor muscle) in that it is predominantly adrenergic (sympathetic nervous system), relying upon the release of the neurotransmitter norepinephrine. The other component is the detrusor muscle, which constitutes the majority of the bladder and forms the cap on the base provided by the trigone. The word detrusor is derived from the term detrudare (= to drive out) and represents a complex admixture of muscle fibres, passing in different directions, which are predominantly under parasympathetic neural control acting via the release of the neurotransmitter acetylcholine acting on muscarinic receptors (the M3 subtype is functionally predominant).
Despite the great deal of work that has been carried out looking at the innervation of the lower urinary tract, a number of aspects of the innervation of the bladder remain unclear. The extrinsic nerves innervating the bladder are demonstrated in Fig. 18.10. The intrinsic nerves are derived from a perivesical plexus which lies on the connective tissue at the base of the bladder and which receives autonomic fibres from two sources: (a) parasympathetic fibres from segments S2–S4, (b) sympathetic fibres from segments T11–L2. It must be borne in mind that the contemporary textbook view of the innervation of the bladder and of the disposition of the autonomic nervous system is oversimplistic, particularly considering the fact that there are ganglia on both the sympathetic and parasympathetic nerves along their course from the spinal cord to the target organ with other ganglia both around and within the target organ, e.g. bladder, prostate, and that it is likely that there are interconnections between both the parasympathetic and sympathetic nervous systems at all of these levels. Furthermore it is now well recognised that there are a number of other sensory/motor neurotransmitters, additional to the classical neurotransmitters norepinephrine and acetylcholine, which may well be implicated in neural control pathways. There is considerable debate as to the sensory innervation of the bladder although recently the importance of purinergic and nitric oxide pathways has been clearly demonstrated. No sensory end organs have yet been identified in the human bladder, and it is thought that sensory nerves are represented by non-myelinated fibres lying within the submucosa and proprioceptive receptors associated with the peritoneum and the adventitia overlying the detrusor muscle at its dome. There has been suggestion of an intramural plexus of sensorimotor neurones, similar to that seen in the intestine, but this is as yet not fully clarified.
URETHRA AND URETHRAL SPHINCTER MECHANISMS
Female urethra
The female urethra is approximately 4 cm long. It opens into the anterior wall of the vagina at the urethral meatus, situated in the vestibule between the anterior ends of the labia minora about 2.5 cm behind the clitoris. Like the rest of the urinary tract it is lined by transitional urothelium. There is an area at the internal urethral meatus on the trigone where the lining is comprised of squamous epithelium which appears to be under hormonal control and which changes its character at different phases during the menstrual cycle. In the female the principal sphincter mechanism is the urethral sphincter mechanism which extends down the length of the female urethra. There is an internal component composed of smooth muscle, the so-called lissosphincter, and an extrinsic component composed of striated muscle, the so-called rhabdosphincter. The sphincter is particularly well developed in the middle third of the urethra. In addition to this sphincter the submucosa of the urethra acts by producing a passive occlusive effect during urethral closure. This submucosa is under hormonal control and is very sensitive to changes in oestrogen levels. There is a very poorly developed bladder neck in the female which does not appear to have a significant functional role.
Male urethra
The male urethra (Fig. 18.11) is approximately 20 cm inlength and comprises an anterior and posterior part. The posterior urethra, approximately 6 cm in length, is composed of that area which traverses the prostate, which is approximately 3–4 cm in length, and that which lies within the confines of the distal sphincter mechanism, which is 2 cm in length. At the external border of the distal sphincter mechanism is the junction of the posterior urethra with the anterior urethra. The anterior urethra can be further subdivided into two areas which are divided on the basis of the areas anterior and posterior to the penoscrotal junction.
REPRODUCTIVE SYSTEMS
In the male there are paired testes where spermatozoa are produced. A pair of tubes, the vasa deferentia, carry sperm back from the testes into the pelvic cavity and into the urethra. There are paired seminal vesicles which produce materials, including sugars, needed for sperm to mature and which drain into the common termination of the vasa deferentia to form the paired common ejaculatory ducts opening into the prostatic urethra. The prostate gland produces much of the bulk of the semen. The penis is traversed by the urethra. In addition to the corpus spongiosum which surrounds the urethra, it comprises paired corpora cavernosa which represent the erectile tissue. It must be remembered that at the tip of the penis, the glans penis is contiguous with the corpus spongiosum and abuts against the corpus cavernosum (Fig. 18.12). Penile erection is essential to successful intercourse and is mediated by the nervi erigentes arising from the S2–S4 nerve roots. Disorders of both penile and clitoral erection have been increasingly recognised in recent years to be a cause of significant concern within the population, and management of these disorders is an important mainstay of the subspecialty of andrology.
FEMALE REPRODUCTIVE TRACT
Ovary
The ovary is the size and shape of an almond and is attached to the posterior aspect of the broad ligament by the mesovarium (Fig. 18.13). At the superior (tubal) pole of the ovary is attached a prominent fold of peritoneum, the suspensory ligament of the ovary, which passes upwards over the pelvic brim and external iliac vessels to merge with the peritoneum over psoas major muscle. The ovarian artery gains access to the ovary through the mesovarium and suspensory ligament. A further ligament, the ovarian ligament, runs within the broad ligament to the cornu of the uterus.
Relations
The relations of the ovary are of considerable importance clinically. They may be divided into:
Uterus
The uterus is a pear-shaped organ which is approximately 7 cm long, 5 cm from side to side at its widest point, and 3 cm anteroposteriorly. It is composed of a fundus, body and cervix. The fallopian tubes enter into each supralateral angle, above which lies the fundus. The features of the uterus, uterine tubes and vagina are shown in Fig. 18.14.
Relations
Blood supply (Fig. 18.15)
The uterine artery, which is a branch of the internal iliac artery, runs in the base of the broad ligament, and about 2 cm lateral to the cervix it passes anterior and superior to the ureter, reaching the uterus at the level of the internal os. The artery then ascends in a tortuous manner, running up the lateral side of the body of the uterus before turning laterally and inferiorly to the uterine tube, where it terminates by anastomosing with the terminal branches of the ovarian artery. The uterine artery also gives off a descending branch which supplies the cervix and the upper vagina. The uterine veins accompany the arteries, draining to the internal iliac vein.
Lymphatic drainage
Lymphatic vessels from the uterus drain in the following manner.
Fallopian tubes
The fallopian tube is covered by peritoneum except for the intramural part. It contains a muscular coat of outer longitudinal and inner circular fibres, and the mucosa is formed of columnar-ciliated cells and lies in longitudinal ridges, each of which is thrown into numerous folds. The function of the tube is to propel ova along the lumen to the uterus. This is accomplished by muscular contraction, ciliary action and by the production of a lubricating fluid. A fertilised ovum may occasionally implant ectopically in the tube. This gives rise to an ectopic pregnancy which may cause rupture of the tube with consequent intraperitoneal haemorrhage. The distal end of the tube is open into the peritoneal cavity, providing direct communication between the peritoneum and the outside and, therefore, a potential pathway for infection.
Vagina
Relations
MALE REPRODUCTIVE TRACT
Testis and epididymis
Prostate
Relations
Clinically the prostate gland is divided into lobes:
Seminal vesicles
The seminal vesicles lie, one on each side, in the interval between the base of the bladder anteriorly and the rectum posteriorly. They lie lateral to the termination of the vasa. Each seminal vesicle has a common drainage with its neighbouring vas via the common ejaculatory duct (Fig. 18.17). The vesicles synthesise and secrete a sticky, yellowish fluid rich in fructose. The normal vesicles cannot be palpated on rectal examination. However, if they are enlarged by infection, e.g. tuberculosis, or local invasion by a prostatic malignancy they may become palpable.
PHYSIOLOGY
FLUID AND ELECTROLYTE BALANCE
The proximal tubule
This decreases the volume of glomerular filtrate by 75–80%, with active resorbtion of glucose, phosphate, bicarbonate, potassium and chloride. It is important to realise that glucose is resorbed entirely from the proximal tubules, unless the glucose load exceeds the capacity for absorption. The majority of filtered sodium and bicarbonate are reabsorbed from the proximal tubules, and sodium is actually pumped via hydrogen/potassium-linked pump mechanisms. The proximal tubular filtrate is iso-osmotic as a consequence of passive absorption of both water and urea. Sulphates, amino acids and low molecular weight proteins are reabsorbed, as is potassium.
HORMONE PRODUCTION BY THE KIDNEY
A number of important hormones are produced with-in the kidney. The renin-angiotensin system is important, with renin being released from juxtaglomerular cells in response to sympathetic nerve stimulation via a decrease in afferent arteriolar pressure and hyponatraemia. Renin acts on circulating angiotensinogen to produce angiotensin I, which is converted by a circulating enzyme to angiotensin II. Angiotensin II stimulates the zona glomerulosa of the adrenal gland to produce aldosterone, which increases the sodium resorbtion by the kidneys and also produces vasoconstriction. These effects feed back in a negative fashion and switch off renin secretion and, therefore, maintain homeostasis. This is a gross oversimplification of an extremely complex system, but nevertheless it is evident that this homeostatic mechanism is essential to maintain a smooth blood pressure and compensate for changes in extracellular fluid volume and sodium excretion.
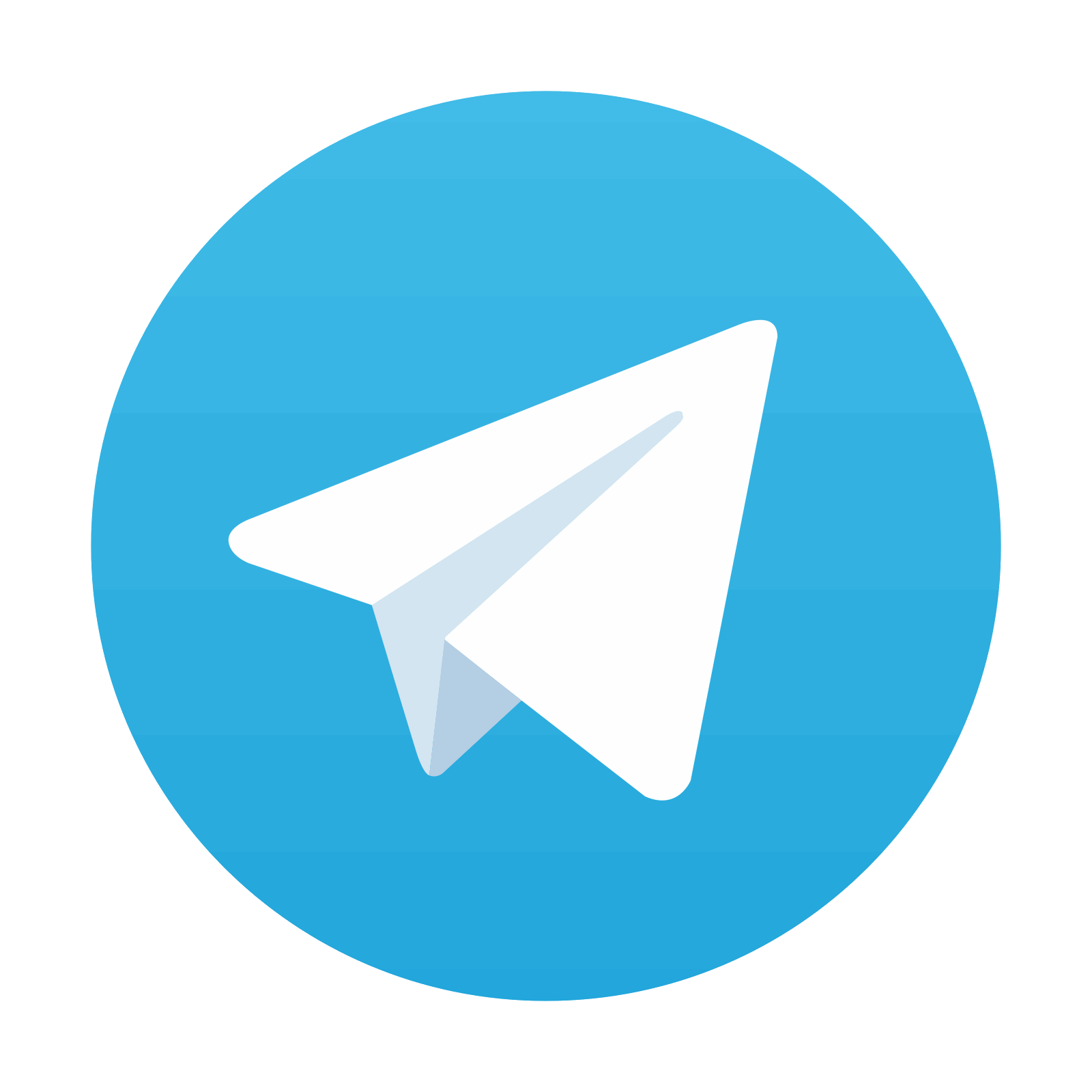
Stay updated, free articles. Join our Telegram channel

Full access? Get Clinical Tree
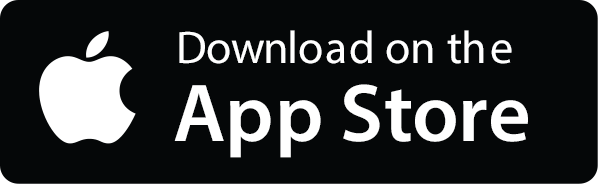
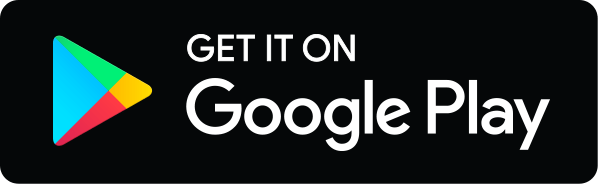