INTRODUCTION
Drugs are typically administered in either pill or injection form, with limited control over release rate and localization. More advanced drug delivery systems have recently been developed, however. The goal of these new technologies is to alter four pharmacokinetic properties: (1) absorption of the drug, including the period of time over which it is released into the systemic circulation or at its final site of action; (2) distribution of the drug, whether it be to the entire body or to a specific tissue or organ system; (3) metabolism of the drug, either to be avoided entirely or used to convert a prodrug to an active form; and (4) elimination of the drug.
This chapter describes several existing and emerging delivery modalities and discusses how these modalities influence one or more of these four properties. The field of drug delivery is large and encompasses many disciplines, and this discussion will highlight approaches that illustrate these properties rather than provide an exhaustive description of all ongoing practice and research. The highlighted modalities include the novel use of existing delivery routes, polymer-based delivery systems, and liposome-based delivery systems.
March 1988: Mr. F is 13 years old. His parents begin to notice that he is tired much of the time, despite getting plenty of sleep. He can no longer participate on his school’s track team because he becomes exhausted in the middle of races—the same races he had often won less than a year earlier. Also, Mr. F complains of being thirsty constantly and, as a result, consumes large quantities of water. Mr. F goes to his family physician, who measures his blood glucose level at 650 mg/dL (approximately six times normal levels) and makes an initial diagnosis of type 1 diabetes mellitus. The diagnosis is confirmed in the hospital, where Mr. F’s physicians stabilize his blood glucose and develop an insulin therapy regimen. He is taught how to draw a drop of blood from his fingertip to measure his blood glucose and how to give himself subcutaneous injections of insulin. Each day, Mr. F injects recombinant human insulin before breakfast and before dinner.
January 1997: Throughout high school and most of college, Mr. F rarely monitors his glucose levels and purposely keeps them higher than recommended. He wants to be as “normal” as possible, which for him means never allowing his glucose level to fall so low as to require food in the middle of a class or at other unusual times. As Mr. F becomes older, he begins to appreciate that avoiding the long-term consequences of poorly controlled diabetes—atherosclerosis, retinopathy, nephropathy, and peripheral neuropathy, among others—is worth the inconvenience of better control. He switches to a regimen involving four injections a day and begins checking his blood glucose four to five times a day. Eventually, he switches from multiple subcutaneous injections (MSI) to continuous subcutaneous insulin infusion (CSII) with an insulin pump. The pump delivers a constant basal level of insulin that can be supplemented with bolus releases before meals, thereby more closely approximating the body’s physiologic control of blood glucose levels.
September 2024: Back in 1997, Mr. F used his insulin pump for only about 3 months, deciding that the small machine he needed to keep constantly attached to his body was not compatible with his active lifestyle or self-image. He resumed MSI therapy for several more years, until he began participating in human trials for a new, implantable insulin delivery system. Now, a 2-year supply of insulin is incorporated into a polymer matrix that can be implanted in the subcutaneous fat of the abdomen. A device in Mr. F’s wristwatch constantly measures his glucose levels transdermally, and it transmits instructions to a magnetic oscillator implanted near the polymer delivery system. The dosing advantages of the insulin pump are thus achieved without Mr. F feeling limited or tied to a machine in any way. He simply has the polymer system replaced every 2 years and makes minor daily adjustments to the programmed delivery parameters in his wristwatch device. Mr. F is looking forward to receiving a transplant of pancreatic beta cells, developed from his own stem cells, that will cure his diabetes.
Questions
1. Why is oral administration of insulin not practical?
2. Which other routes of insulin administration have been tried?
3. Which technologies may make it possible to monitor blood glucose levels transdermally?
4. How can polymers be used to optimize and simplify administration of some drugs?
NOVEL USE OF EXISTING DELIVERY ROUTES
Oral administration of small molecules is currently the most common method of drug delivery. The main advantages of oral delivery are ease of use and relatively low cost, both of which can improve patient adherence. However, incomplete absorption, metabolism of the drug during absorption, and metabolism of the drug during the first pass through the liver can decrease bioavailability of the drug. The variability of these factors, as well as limitations in dosing frequency, also affect the ability to maintain a therapeutic drug concentration in the blood. In addition, only relatively small molecules can be used in conventional pills: the intestine generally cannot absorb large molecules intact. Intact peptide and protein drugs, such as insulin, are poorly absorbed orally because of proteolysis in the digestive tract. Recent advances and ongoing research in oral drug delivery are beginning to address these issues.
Sustained or extended-release formulations can prolong plasma drug concentrations with less frequent doses. In early approaches to sustained release, pill or capsule solubility was modified with one or more inert substances known as excipients. By formulating the drug in an emulsion or suspension that is relatively difficult to digest, the period of time over which the drug dissolves and is absorbed can be extended. Similar results have been achieved by coating the drug with substances such as cellulose derivatives or wax. This approach is used in a wide variety of both prescription and over-the-counter medications. Another successful and more recent approach to sustained release oral formulations involves an osmotic pump capsule (see below).
Techniques are also being developed for delivery of larger molecules, such as proteins and DNA, in oral formulations. Several designs make use of drug-carrying vehicles, including liposomes and microspheres. Liposomes, small vesicles with lipid bilayer membranes, are lipophilic and can be taken up by intestinal Peyer’s patches when targeted to M cells (specialized epithelial cells) with appropriate ligands. Certain types of liposomes have been moderately successful in experimental oral vaccine delivery; their use in intravenous delivery systems is discussed below. Polyanhydride microspheres, which adhere strongly to the intestinal mucosal surface, have been shown to penetrate intestinal epithelium. After the microspheres are absorbed, presumably because they can stay in contact with the intestinal epithelium for long periods of time, the complex molecules carried within them can be released into the blood.
Another potential approach to delivering proteins orally involves targeting the drug to the colon, which has lower levels of protease activity than the upper gastrointestinal tract. For example, microsphere delivery vehicles can be synthesized from polymers that have enzymatically degradable azoaromatic cross-links. The colon has a relatively high concentration of azoreductases, facilitating degradation of the microspheres and protein release within the colon. Substances that transiently increase the permeability of colonic epithelium, possibly co-incorporated in the microspheres, may improve the absorption of proteins delivered to the colon. Another approach involves carrier molecules that may be able to shuttle large molecules across the epithelial lining of the intestine.
Patients suffering from asthma and other respiratory diseases have long been able to treat their condition by inhaling aerosols of drugs directly into their lungs: β2-adrenergic agonists, such as albuterol, and glucocorticoid analogues are widely used examples of such locally delivered drugs. In early metered-dose inhaler designs, many of which are still used, the drug is delivered in liquid form using a high velocity chlorofluorocarbon (CFC) propellant. With this technique, very little drug is reproducibly delivered to the lung—often less than 10%. Particles often accumulate in the mouth and throat, and many are immediately exhaled. Components of the immune system and macrophages in the lung can also clear some of the drug before it can act. In addition, many patients use their inhalers incorrectly; common mistakes include not shaking the inhaler well enough, pressing the inhaler too early or too late during inhalation, or using an empty inhaler. Incorrect use further reduces delivery efficiency.
Inhaler design continues to improve. Recent advances include more consistent dosing, greater ease of use via electronic breath actuation, and non-CFC propellants. The aerosol formulations have also been improved by adjusting several properties of the particles themselves. For example, optimized particle chemistry and surface morphology can minimize undesirable particle–particle aggregation. Similarly, particle solubility can be modified to influence the rate of therapeutic release once delivered. Dry powder aerosol clouds that reach deep into the lungs can be generated by blowing compressed air into a drug powder, breaking the powder into tiny (1–5 μm) particles inside the inhaler. Devices that take advantage of these improvements have reduced both dosing frequency and cost for local applications of pulmonary drug delivery in patients with asthma and cystic fibrosis.
The lung also offers several potential advantages for noninvasive, systemic delivery of molecules. The large alveolar surface area, thin tissue lining, and limited numbers of proteolytic enzymes make the lung an ideal tissue for proteins and peptides to enter the bloodstream. One dry powder aerosol device has been approved for the pulmonary delivery of insulin but is not currently manufactured or marketed due to poor acceptance among patients and physicians. Insulin remains an attractive candidate for inhalation therapy, however, as do other biotherapeutics that are currently administered subcutaneously—such as growth hormone, glucagon, and α1-antitrypsin.
One approach to achieving increased delivery efficiency is the design of large, highly porous aerosol particles with very low densities. Such particles tend to aggregate less than smaller, denser particles, resulting in more efficient aerosolization. In addition, these particles have an “aerodynamic diameter,” a parameter based on both density and actual particle dimensions, similar to conventional aerosol particles; thus, they can reach the deep parts of the lung through an airstream, despite their relatively large (5–20 μm) size. Once deposited, the particles can escape clearance by alveolar macrophages, because phagocytosis of particles by macrophages diminishes with increasing particle size beyond 2–3 μm. Thus, drugs can be delivered more efficiently over longer periods of time. In one study, insulin was encapsulated in biodegradable polymer microspheres. Some of the microspheres were small and nonporous, and some were large and porous (low density), but both types had similar aerodynamic diameters. When the microspheres were delivered into the lungs, the relative bioavailability of the large, porous insulin particle was about seven times greater, and the total time of insulin release into the systemic circulation was about 24 times longer, than that of the conventional particle.
The stratum corneum, composed of lipids and keratinocytes, is the outermost skin layer and the major barrier to transdermal transport. Small, lipophilic drugs have been successfully delivered through the skin into the systemic circulation by passive diffusion at low flux rates, thereby avoiding first-pass metabolism by the liver. Currently, passive transdermal patches are available for hormone replacement and for pharmacologic treatment of motion sickness, angina, nicotine withdrawal, hypertension, pain, and other conditions.
In addition to providing higher bioavailability while remaining noninvasive, transdermal delivery systems are often associated with fewer adverse effects than conventional oral dosage forms. For example, potential liver damage during first-pass metabolism is avoided when a drug is delivered by the transdermal route. Thus, more sophisticated transdermal systems are under development in an attempt to provide these advantages for drug molecules that are otherwise unable to penetrate the skin. Iontophoresis is one approach to enhancing the transport of charged, low-molecular-mass molecules through the skin. Iontophoresis involves the application of low-voltage electric pulses for long time periods; this technology is already used clinically for local applications, such as therapy for hyperhidrosis (excessive perspiration), and is under development for systemic delivery of small-molecule analgesic drugs. The use of high-voltage pulses for a short time period—on the order of milliseconds—is also being explored. In human cadaver skin, which is commonly used as a model for skin transport, such high-voltage pulses have been shown to induce temporary pores. This phenomenon, known as electroporation, will potentially allow systemic delivery of large, charged molecules, such as heparin and oligonucleotides. Microneedles are also being studied.
Ultrasound enhancement of drug delivery through the skin, termed sonophoresis, is also being explored for molecules such as insulin, interferon, and erythropoietin. Application of ultrasound to the skin results in cavitation, the formation of tiny air-filled spaces in lipid bilayers of the stratum corneum. The net result of cavitation is disordering of the lipid bilayers, enhancing diffusivity of the drug through the skin by up to 1,000 times. Sonophoresis does not damage the skin, which typically regains its normal structure within 2 hours, and no undesirable effects have been observed in early clinical trials.
Sonophoresis can also be used to remove diagnostic samples from the extracellular space under the stratum corneum. Experiments have been devised in which a reservoir was placed between an ultrasound transducer and a rat’s skin, and interstitial fluid was extracted. Theophylline, glucose, cholesterol, urea, and calcium could be measured in the sample; the glucose measurements were sufficiently accurate to be used as a surrogate for blood glucose monitoring in diabetics. With a portable ultrasound transducer, this technique could be incorporated in a futuristic device such as the one Mr. F used in 2024.
POLYMER-BASED DELIVERY SYSTEMS
Polymer-based drug delivery systems gradually release drugs into their surroundings. Polymer delivery mechanisms are widely used in diverse applications such as birth control, chemotherapy, and antiarrhythmic therapy. These systems offer advantages in both controlled release and targeting of drugs and are thus the focus of much research. Drug delivery from a polymer-based system can be achieved via three general mechanisms: (1) diffusion, (2) chemical reaction, and (3) solvent activation (Fig. 55-1).
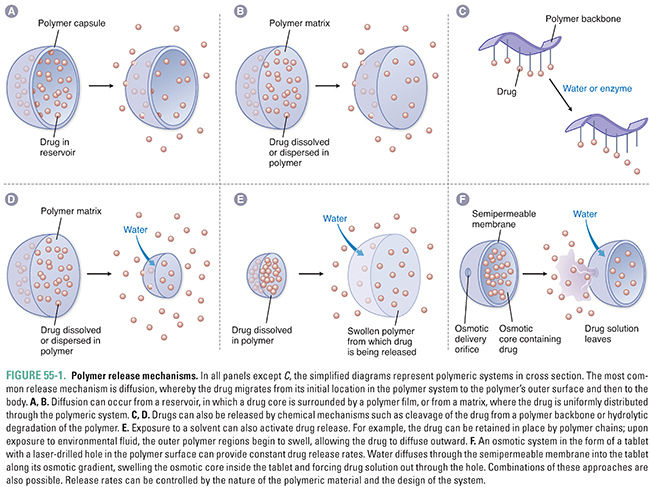
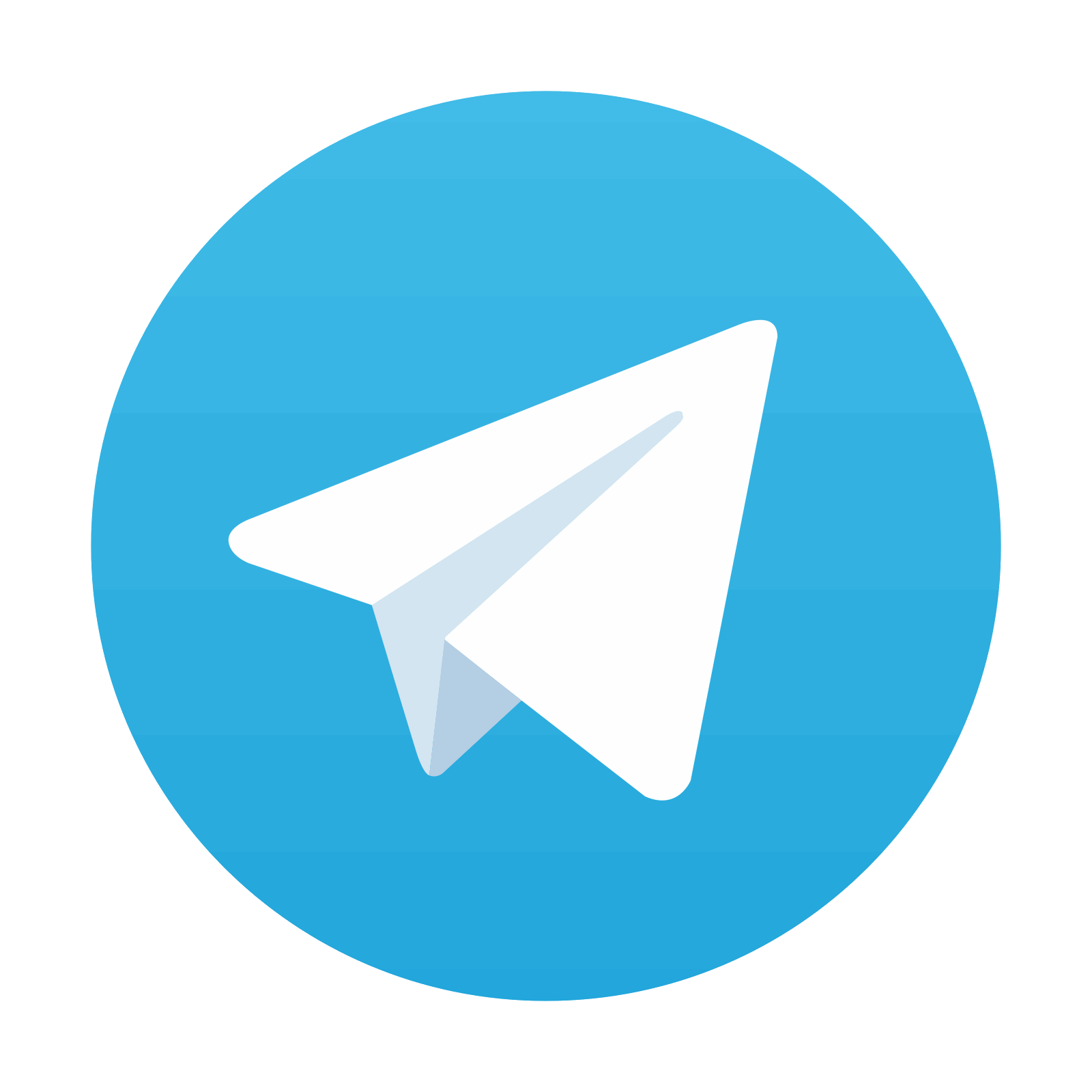
Stay updated, free articles. Join our Telegram channel

Full access? Get Clinical Tree
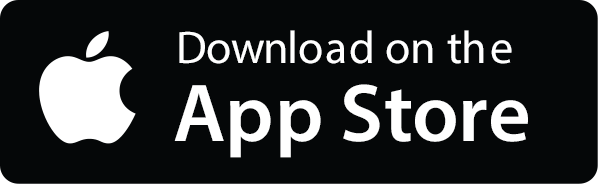
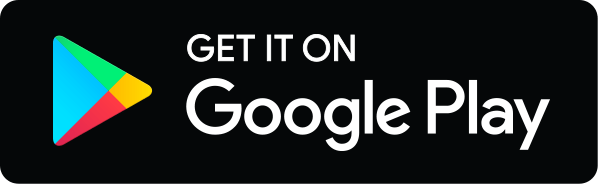