Figure 13.1Equilibrium moisture content of empty gelatin capsule shells stored at different relative humidities for 2 weeks at 20°C. (Reprinted from Adv Drug Del Rev, 60, Cole ET, Cade D, Benameur H, Challenges and opportunities in the encapsulation of liquid and semi-solid formulations into capsules for oral administration, 747–756, Copyright 2008, with permission from Elsevier.)
Another issue pertinent to gelatin capsules is cross-linking reactions from aldehydes. The presence of even low levels of aldehydes inherent in the excipients and drug or produced via decomposition during storage can cause an unexpected delay in drug release as well as stability problems [3–5]. In addition to the presence of aldehydes, the other main contributory factors toward cross-linking were found to be storage stresses (i.e., high temperatures, high humidity, and excessive light exposure) [4].
However, sometimes this reaction can be beneficial. Gelatin, like the majority of protein-based films, has a limited barrier to water vapor transmission. Increasing cross-linking by reacting gelatin films with formaldehyde not only can improve gelatin’s mechanical properties but also can provide gastro-resistance [6,7]. It has also been reported by Kuijpers et al. [8] that chemical cross-linking via the carboxylic acid groups on gelatin can increase the resistance of the gels against thermal degradation.
Pina et al. [9] studied seven drugs with different solubilities in gastric juice. These drugs were then encapsulated and the gelatin capsules were coated with different formaldehyde concentration solutions ranging from 3.0 to 20.0% w/w. The results allowed for the establishment of a relation between the drug’s solubility in gastric juice (S), and formaldehyde concentration in coating solution (C). It is given by

where C is the formaldehyde concentration and S is the drug’s solubility in gastric juice.
The capsule wall may comprise gelatin or another suitable polymer such as hypromellose also called hydroxypropylmethylcellulose (HPMC). The equilibrium moisture content of HPMC capsules is lower and ranges from 2% to 6%. These capsules are also not susceptible to cross-linking reactions with aldehydes as this polymer does not contain chemically reactive groups. The choice of whether to use a hard or soft capsule will depend on several factors, including the nature of the formulation components, the compatibility of the excipients with the shell polymer, the best storage stability conditions, and the drug release characteristics.
The physicochemical properties of both HPMC and gelatin capsules are presented in Table 13.1 [10,11]. The largest difference between the two shell materials is in their moisture contents, which is between 2% and 6% for HPMC capsules and 13% and 16% for gelatin capsules. The relationship between the moisture content of the capsule shells and the resultant brittleness has previously been demonstrated using a hardness tester [12]. HPMC and gelatin capsules were filled with acetylsalicylic acid and stored at 60°C for 2 weeks. While drug potency was maintained above 95% for HPMC capsules, it decreased to 85% of its initial concentration when stored in gelatin capsules, apparently as a result of hydrolysis. Moreover, the percentage of broken gelatin capsules increased to almost 100% as the moisture content of the capsule shells decreased below 10%. In contrast, HPMC capsules remained undamaged even at moisture levels of only 2%. These data suggest that HPMC capsules could be more appropriate for actives that are either hygroscopic or prone to hydrolysis.
Property | HPMC | Gelatin |
---|---|---|
Moisture content | 2–6% | 13–16% |
Glass transition temperature | 170–180°C | 50–60°C |
Water vapor permeability | Low | Higher |
Substrate for protease | No | Yes |
Maillard reaction with drug fill | No | Yes |
Deformation by heat | >80°C | >60°C |
Water dissolution at room temperature | Soluble | Insoluble |
Static | Low | High |
Light degradation | No | Possible |
Source: Honkanen O. Biopharmaceutical Evaluation of Orally and Rectally Administered Hard Hydroxypropyl Methylcellulose Capsules. Dissertation submitted to the Faculty of Pharmacy, University of Helsinki, 2004; Rowe RC, Sheskey PJ, Cook WG, Fenton ME (Eds.). Handbook of Pharmaceutical Excipients, 7th edition, 2012. Pharmaceutical Press, Philadelphia, PA.
Another polymer that has received GRAS (generally recognized as safe) status from the Food and Drug Administration (FDA) in 2002 is pullulan. This polymer has already been approved in Japan for more than 20 years and is currently being evaluated for use as a food additive in Europe. These capsules are made from a pullulan polymer naturally fermented from tapioca starch [13–15]. Pullulan films display excellent oxygen barrier properties and the capsules have a crystal-clear transparency and a high degree of luster, ideal for marketing in the natural products industry. The other marketing advantages for these capsules are that they are Kosher, allergen-free, and manufactured from non-genetically modified organisms.
Capsules may also be enteric coated in order to prevent the premature release of their contents in the stomach, where the low-pH conditions can damage those active ingredients susceptible to acid hydrolysis [7,8,16]. An added benefit is that the capsule coating helps mask any unpleasant ingredient odor or taste. These capsules would be ideal for acid-sensitive active ingredients such as resveratrol, clopidogrel (Plavix), and 5-hydroxytryptophan (5-HTP) and also for items such as garlic.
Each of the different polymers used in the manufacture of capsules have different physicochemical and mechanical properties that will affect the release rate and stability of the drug product. There are a number of analytical methodologies that can yield important information regarding the capsule dosage form itself during manufacturing and storage, and these include the polymer’s glass transition temperature (Tg) and level of moisture loss. The amount of moisture loss can affect the brittleness of the capsule shell during storage and also affect the in vivo drug release.
These analytical techniques include differential scanning calorimetry (DSC), dissolution, and high-performance liquid chromatography (HPLC). DSC can be used to yield a true picture of what is occurring on a micro-scale with the polymeric film before physical changes (e.g., capsule shrinkage, deformation, and/or brittleness) are observed. Moreover, these techniques can also be used with other complementary spectroscopic techniques (i.e., Fourier transform infrared spectroscopy, Raman spectroscopy, or near-infrared spectroscopy [NIRS]) in order to identify potential impurity profiles and to confirm the identity of certain HPLC peaks of interest.
Polymers Used In Capsules
Gelatin
Gelatin is a product obtained by the partial hydrolysis of collagen derived from the skin, connective tissue, and bones of animals. Gelatin is an amphoteric material. When derived from an acid-treated precursor, it is known as Type A; when derived from an alkali-treated precursor, it is known as Type B. Gelatin may be colored with certified colors, may contain no more than 0.15% of sulfur dioxide, and may also contain a suitable concentration of sodium lauryl sulfate (SLS) and suitable antimicrobial agents. The pharmacopeial specifications for gelatin are presented in Table 13.2.
Test | JP XV | PhEur 7.4 | USP35-NF30 |
---|---|---|---|
Identification | + | + | + |
Characters | + | + | − |
Microbial contamination | − | + | + |
Aerobic bacteria | − | ≤103 CFU/g | ≤103 CFU/g |
Fungi | − | ≤102 CFU/g | − |
Residue on ignition | ≤2.0% | − | ≤2.0% |
Loss on drying | ≤15.0% | ≤15.0% | − |
Odor and water-insoluble substances | + | − | + |
Isoelectric point | + | + | − |
Type A | 7.0–9.0 | 6.0–9.5 | − |
Type B | 4.5–5.0 | 4.7–5.6 | − |
Conductivity | − | ≤1 mS/cm | − |
Sulfur dioxide | − | ≤50 ppm | ≤0.15% |
Sulfite | + | − | − |
Arsenic | ≤1 ppm | − | ≤0.8 ppm |
Iron | − | ≤30 ppm | − |
Chromium | − | ≤10 ppm | − |
Zinc | − | ≤30 ppm | − |
Heavy metals | ≤50 ppma | − | ≤50 ppm |
pH | − | 3.8–7.6 | − |
Mercury | ≤0.1 ppm | − | − |
Peroxides | − | ≤10 ppm | − |
Gel strength | − | + | − |
Source: Reprinted with the permission of the American Pharmacists Association (Washington, DC) from Rowe RC, Sheskey PJ, Cook WG, Fenton ME (Eds.). Handbook of Pharmaceutical Excipients, 7th edition, 2012. Pharmaceutical Press, Philadelphia, PA.
a ≤20 ppm for purified gelatin.
A standard test to measure the gelling ability of various gelatins is termed the bloom strength and is measured in grams. This test is described under USP General Chapter gel strength of gelatin <1081> [17]. This test measures the force required to depress the surface of a 6.67% w/w gel, matured at 10°C for 16–18 h in a standard bottle, adjusted for a depression of 4 mm using a 12.7-mm-diameter flat-bottomed plunger.
The physical properties of bloom strength and viscosity vary with the moisture content of the dry gelatin, and it is commonplace to correct these values to a standard moisture content of 11.5%. The bloom strength can vary depending on the source of the gelatin and whether it is used for soft or hard shell manufacture. For example, the bloom strength of Type B, fish-derived, and Type A gelatins used for soft capsules ranges from 150 to 175, from 160 to 210, and from 180 to 210, respectively. However, the bloom strength of Type B and Type A (from bone) ranges from 200 to 220 and from 245 to 270, respectively [1].
Stability Issues: Cross-Linking
Gelatin is mixed with a plasticizer, such as glycerin, to produce soft gelatin capsules. For hard gelatin capsules, the residual water acts as a plasticizer. While it can also be treated with formaldehyde to produce gastro-resistance, there are toxicity issues with this compound [18]. Analytical methods are available for the identification and quantification of aldehydes. While Li et al. [19] described a headspace gas chromatographic method, del Barrio et al. [20] utilized a gas chromatographic–mass spectroscopy (GC-MS) method and claimed that the detection limit for formaldehyde was 0.2 parts per million (ppm) (range, 0.2–10,000 ppm). However, another simple and sensitive method using HPLC can also be utilized for those labs without GC-MS capability [21].
A pivotal study by Meyer et al. [22] compared cross-linked (stressed) hard and soft gelatin capsules of acetaminophen with their unstressed controls. The authors found that the in vitro rate of dissolution dramatically decreased when the degree of cross-linking increased. Additionally, capsules stressed to the greatest extent were not bioequivalent to the unstressed control capsules.
This study resulted in the adoption of a USP two-tier procedure for dissolution testing of gelatin capsules that allowed the use of enzymes such as pepsin and pancreatin in the dissolution media when pellicle formation (i.e., a thick skin or film formed on a liquid) causes a delay in drug dissolution [4,23]. The USP states that pepsin and pancreatin can be added to dissolution media containing either simulated gastric fluid (SGF) or simulated intestinal fluid (SIF), respectively, depending on the dissolution media specified for the product and the drug’s solubility. This revised procedure using enzymes enables an improved ability to distinguish whether gelatin capsules are bioequivalent to each other. Only in cases where the enzymes have been added and the test still shows poor dissolution rate should a negative effect attributed to cross-linking be assumed.
Details on the types and amounts of enzymes to be used can be found in the USP General Chapter <711> Dissolution. Where water or a medium with a pH of less than 6.8 is used, purified pepsin can be added to the medium in an amount that results in an activity of 750,000 U or less per 1000 mL. For media with a pH of 6.8 or greater, pancreatin can be added to produce not more than 1750 U of protease activity per 1000 mL. The pancreatin or pepsin to be used should meet the USP monograph requirements [24].
Marchais et al. [23] designed studies to determine whether the use of both enzyme and surfactant in the dissolution medium changes the in vitro drug release from cross-linked hard gelatin capsules containing the water-insoluble drug carbamazepine. Hard gelatin capsules were cross-linked by a controlled exposure to formaldehyde, resulting in different stressed capsules. In vitro dissolution studies were conducted with enzymes and using SGF containing pepsin and SIF containing pancreatin. SLS was also added in the dissolution medium at a concentration of 2% w/v in both SGF and SIF.
The percentage of carbamazepine dissolved was reduced by increasing the degree of gelatin cross-linking. For unstressed hard gelatin capsules, 36% of the carbamazepine was released after 1 h. However, this was greatly lowered to 5% for highly stressed hard gelatin capsules in the SGF. A similar effect was also observed with SIF. In the case of moderately stressed hard gelatin capsules, addition of enzyme in the dissolution medium enhanced the percentage of carbamazepine dissolved. The dissolution level increased from 12% to 39% in SGF with pepsin for hard gelatin capsules cross-linked with 1500 ppm formaldehyde. On the contrary, the use of enzyme in the dissolution medium did not increase the dissolution of carbamazepine from highly stressed hard gelatin capsules. Surprisingly, the addition of SLS in either medium did not allow the release of the carbamazepine. The results of this study demonstrate that the use of enzymes in the dissolution medium is justified for moderately cross-linked hard gelatin capsules. However, the action of a surfactant added in the medium containing enzymes remains unclear.
The selection of the formulation excipients is important because of their potential interaction with the capsule shells and their negative impact on both long-term stability and drug dissolution rate. Pellicle formation can be dependent on the excipients present in the gelatin shell. For example, Chang et al. [25] observed that after 12 weeks of storage at 40°C/75% RH, gelatin capsules containing lactose-based granules had reduced dissolution rates owing to pellicle formation inside capsule shells, while capsules containing mannitol-based granules showed faster dissolution profiles without noticeable pellicle formation.
There is some concern that a number of excipients for liquid-filled capsule formulations such as fats, polyethylene glycols (PEGs), aliphatic alcohols, phenols, polyoxylenated glycerides, and esters of unsaturated fatty acids can undergo auto-oxidation to form aldehydes [26]. It has previously been found that lactose can be incompatible with gelatin capsules owing to the presence of a preservative, hexamethylenetetramine, which decomposes into formaldehyde and causes cross-linking reactions with gelatin [4]. However, while this additive is approved in the European Union, it is not approved for use in the United States, Australia, or New Zealand.
When glycine and citric acid were incorporated together into a triamterene/hydrochlorithiazide formulation, cross-linking was completely prevented. Dissolution profiles remained the same throughout a 3-month accelerated stability program at 50°C. These results were confirmed using gemfibrozil and piroxicam as model drugs and showed that pellicle formation during dissolution was prevented [27]. The cross-linking susceptibility of capsules under ambient conditions was compared by Ku et al. [28] using lactose spiked with 25 ppm formaldehyde. After 1 week of storage at room temperature, the dissolution of acetaminophen from the HPMC shell (Vcaps Plus, containing no gelling agent) remains unchanged while the gelatin shell showed a significant decrease in the drug release (Figure 13.2).

Figure 13.2Dissolution of acetaminophen in hard gelatin capsules (HGC) and HPMC capsule shells after 1-week exposure to lactose spiked with formaldehyde. (Reprinted from Int J Pharm, 386, Ku SM, Li W, Dulin W, Donahue F, Cade D, Benameur H, Hutchison K. Performance qualification of a new hypromellose capsule: Part I. Comparative evaluation of physical, mechanical and processability quality attributes of Vcaps Plus, Quali-V and gelatin capsules, 30–41, Copyright 2010, with permission from Elsevier.)
Attenuated total reflection Fourier transform infrared spectroscopy (FTIR) has been used by Tengroth et al. [29] to study cross-linking reactions in hard gelatin capsules induced by exposure to formaldehyde, acetaldehyde, and propionaldehyde. These aldehydes are known to cause cross-linking between the amino acid chains of gelatin. Using FTIR spectroscopy, it is possible to analyze the cross-linking mechanisms by studying changes in the vibrational bands of the gelatin spectrum. The FTIR spectrum changes over time when the capsules are left in an aldehyde-rich environment. Analysis of the spectra shows that the early observed spectral changes conform to reaction intermediates proposed in previous work based on nuclear magnetic resonance experiments, specifically the formation of an amine methyl alcohol. Thus, the aldehydes combine with the primary amine groups from the arginine and lysine residues and this is followed by the formation of cross-links.
Further spectral changes appear to be mostly from unreacted aldehydes absorbed to the gelatin, although a minor shift of the amide II peak is attributed to cross-link formation. Another quality issue affecting the stability of capsule shells is moisture transfer. While it has been known that brittleness in hard gelatin capsules is a function of moisture content, a minimum quantity of sorbed moisture is necessary to act as a plasticizer and maintain the capsules in a pliable state. Kontny and Mulski [30] showed that the brittleness of both empty gelatin capsules and formulation-filled capsules becomes prevalent at RH values of less than 40%. The initial moisture contents and masses of all components are important in establishing the final equilibrium RH in a given capsule system.
Variation in moisture content of the capsule shells due to either the change of storage conditions or the moisture transfer between the capsule shell and its contents may lead to undesired physical properties, such as capsule brittleness and stickiness. Chang et al. [31] used Sorption–Desorption Moisture Transfer model fitting to study the effects of using a very hygroscopic drug encapsulated in hard gelatin shells and to predict the equilibrium RH in their system. The neat drug (moisture level, 1.7%) was compared to a formulation where the active was pre-moistened to a moisture content of 7%. Both samples were stored under various stability conditions for a period of 1 year. The authors found that by adding an adequate amount of water to the drug substance, moisture transfer issues and gelatin brittleness on storage can be reduced or eliminated.
Gold et al. [32] used NIRS to predict the degree of cross-linking from formaldehyde-stressed hard gelatin capsules. Capsules were filled with amoxicillin and then exposed to a 150 ppb (parts per billion) atmosphere of formaldehyde vapor for 2.3, 4.6, 9.4, 16, and 24 h. Dissolution profiles were obtained and then principal component regression (PCR) was used to analyze the data. The dissolution of amoxicillin was found to decrease with increasing exposure time to the formaldehyde atmosphere. The authors found that a good correlation (R2 = 0.963) was established when principal components were regressed against amoxicillin percentage dissolved at 45 min. Furthermore, the water content of the capsules was the largest determinant in the variation between the capsule’s spectra at each exposure time. The NIRS absorbance of the capsules at 1450 and 1949 nm decreased with the increased exposure time to formaldehyde.
Hydroxypropylmethylcellulose
Gelatin capsules may not provide sufficient protection against moisture for some active pharmaceutical ingredients (APIs) and the gelatin shells can also become brittle over time leading to capsule breakage [33–35]. Furthermore, while some consumers prefer that their medicines be free of animal-derived ingredients such as gelatin because of issues such as bovine spongiform encephalopathy, vegetarian capsules manufactured using hypromellose or HPMC have been on the market since 1998 [10].
Traditionally, hard gelatin capsule manufacturing is performed using dip molding where stainless steel pins at ambient temperature are dipped into a heated solution of liquid gelatin. The preparation of HPMC capsules is the opposite. The stainless steel molding pins are heated and subsequently dipped into an HPMC solution at ambient temperature. However, previously, HPMC required secondary gelling agents such as kappa-carrageenan or gellan gum as well as ionic promoters (i.e., potassium or sodium ions, respectively), in order to form a quick setting film on the molding pins. The issue with the use of secondary gelling systems is that they can interact with the dissolution media and cause an unexpected delay in drug release [36].
Differences have been shown in the in vitro dissolution rate between gelatin and HPMC capsules [37]. However, the bioavailability of ibuprofen, a BCS Class II drug, delivered from the two capsule types was not statistically different when AUC and Cmax values were compared using pharmacoscintigraphic evaluation. Recent advances made in HPMC capsule manufacturing technology have resulted in the achievement of similar in vitro dissolution rates as compared with gelatin capsules.
Ku et al. [28] described the qualification of a new high-performance hypromellose capsule shell that contains no gelling agent and is dissolution friendly. Comparisons to gelatin and HPMC capsules containing carrageenan showed that the new HPMC capsules without any gelling agent are superior in terms of mechanical strength, hygroscopicity, and compatibility with a wide range of drugs. Specifically, the new HPMC capsules demonstrated improved weight variation, machineability, and powder leakage than the HPMC capsules containing carrageenan. The new capsule also demonstrated a broader applicability than gelatin capsule for new drug development because of its inertness and compatibility for a wide range of excipients including those used for liquid fill formulations. On the basis of the superior dissolution performance and other quality attributes, the new HPMC capsule is satisfactorily qualified and has since been used successfully for nearly 20 investigational new drug compounds.
The HPMC capsule was studied further by Ku et al. [38]. This new capsule does not contain any gelling agent and is manufactured by a thermal gelation process. Rupture time of the carrageenan-containing capsule (HPMC Shell 1) and HPMC Shell 2 (containing no gelling agent), as measured by an improved real-time detection method, showed only slight differences that did not manifest in vivo. The absence of a gelling agent appeared to give HPMC Shell 2 advantages in dissolution in acidic media and in buffers containing potassium ions. Slow drug release of HPMC Shell 1 in 0.1 M HCl was attributed to the interaction of carrageenan with drug compounds, whereas the presence of potassium ions, a gelling promoter for carrageenan, caused a delay in capsule opening and a larger capsule-to-capsule variation. Disintegration and dissolution performances of both hypromellose capsules are comparable in other dissolution media tested.
Ku et al. [38] showed that the HPMC shell containing carrageenan tended to open up somewhat faster than the pure HPMC shell material (Figure 13.3). The authors believed that the carrageenan helped to facilitate the dissolution of the HPMC polymer. The average capsule rupture time in the various media is in the range of 2.7–4.3 min for HPMC Shell 1 and 6.1–8.1 min for HPMC Shell 2. The differences (<4 min) in capsule opening/rupture times between the two HPMC capsules are not expected to have a significant impact on their in vivo performance. Other studies have shown that the in vitro rupture time or disintegration time of HPMC Shell 1 is generally slower than that of hard gelatin capsules in dissolution media at 37°C [39,40]. Additionally, it has also been shown that there is no significant difference in the in vivo capsule disintegration and dissolution times and, thus, no impact on the pharmacokinetic parameters [41].

Figure 13.3Disintegration/rupture time of HPMC Shell 1 (Quali-V) and HPMC Shell 2 (VCaps Plus) in four dissolution media, 0.1 M HCl, pH 4.5 acetate buffer, pH 6.8 phosphate (sodium salt), and 1% SLS in water. The error bars represent standard deviation of six capsules. (Reprinted from Int J Pharm, 416, Ku M, Lu Q, Li W, Chen Y. Performance qualification of a new hypromellose capsule: Part II. Disintegration and dissolution comparison between two types of hypromellose capsules, 16–24, Copyright 2011, with permission from Elsevier.)
The effect of elevated temperature and time was compared between hypromellose and gelatin capsules and studied by Missaghi and Fassihi [42]. The results showed that the longer exposure to elevated temperatures resulted in a larger moisture loss for gelatin shells as compared with hypromellose shells (i.e., 21.99% vs. 3.62% at 72 h, respectively). The authors believed that this extended period was responsible for reducing the plasticity of the gelatin capsules, thus resulting in their increased brittleness in Table 13.3. There are four different grades of HPMC, with the major differences being the levels of methoxy and hydroxypropoxy groups. The pharmacopeial specifications for hypromellose are presented in Table 13.4.
Weight Reduction(%) | ||
---|---|---|
Exposure Time to 45°C (h) | Hypromellose Capsules | Gelatin Capsules |
1 | 2.80 ± 0.22 | 5.92 ± 0.47 |
24 | 2.97 ± 0.27 | 7.55 ± 0.85 |
72 | 3.62 ± 0.32 | 21.99 ± 2.97 |
Source: Adapted from Missaghi S and Fassihi R. Evaluation and comparison of physicomechanical characteristics of gelatin and hypromellose capsules. Drug Dev Ind Pharm 2006; 32: 829–838. Reproduced with permission of Taylor & Francis via Copyright Clearance Center.
Note: Data are reported as mean value and standard deviation (n = 20).
Test | JP XV | PhEur 7.4 | USP35-NF30 |
---|---|---|---|
Identification | + | + | + |
Characters | − | + | − |
Appearance of solution | − | + | − |
pH (2% w/w solution) | 5.0–8.0 | 5.0–8.0 | 5.0–8.0 |
Apparent viscosity | + | +a | + |
<600 mPa s | 80–120% | 80–120% | 80–120% |
≥600 mPa s | 75–140% | 75–140% | 75–140% |
Loss on drying | ≤5.0% | ≤5.0% | ≤5.0% |
Residue on ignition | ≤1.5% | ≤1.5% | ≤1.5% |
Heavy metalsb | ≤20 ppm | ≤20 ppm | ≤20 ppm |
Methoxy content | |||
Type 1828 | 16.5–20.0% | 16.5–20.0% | 16.5–20.0% |
Type 2208 | 19.0–24.0% | 19.0–24.0% | 19.0–24.0% |
Type 2906 | 27.0–30.0% | 27.0–30.0% | 27.0–30.0% |
Type 2910 | 28.0–30.0% | 28.0–30.0% | 28.0–30.0% |
Hydroxypropoxy content | + | +a | + |
Type 1828 | 23.0–32.0% | 23.0–32.0% | 23.0–32.0% |
Type 2208 | 4.0–12.0% | 4.0–12.0% | 4.0–12.0% |
Type 2906 | 4.0–7.5% | 4.0–7.5% | 4.0–7.5% |
Type 2910 | 7.0–12.0% | 7.0–12.0% | 7.0–12.0% |
Source: Reprinted with the permission of the American Pharmacists Association (Washington, DC) from Rowe RC, Sheskey PJ, Cook WG, Fenton ME (Eds.). Handbook of Pharmaceutical Excipients, 7th edition, 2012. Pharmaceutical Press, Philadelphia, PA.
Pullulan
Pullulan is a linear, neutral exopolysaccharide produced by the fungus Aureobasidium pullulans. This polymer consists of maltotriose repeating units joined by α-1,6 linkages. The internal glucose units within maltotriose are connected by α-1,4-glycosidic bonds. The molecular weight for pullulan ranges from 4.5 × 104 to 6.0 × 105 daltons and depends on the growth conditions of the organism. Pullulan is non-hygroscopic and non-reducing, is soluble in hot and cold water but generally insoluble in organic solvents, and has a glass transition temperature of over 150°C [16]. Its unique linkage pattern grants pullulan films several distinctive physical properties such as mucoadhesive ability, the capacity to form fibers and thin biodegradeable films, which are transparent and impermeable to oxygen [43].
The mechanical strength of hard gelatin capsules depends on the RH both inside and outside the capsule. Sakata and Otsuka [44] studied the relationship between moisture content and the mechanical strength of both hard pullulan capsules filled with potato starch and solvent-cast pullulan films (15% w/w) using thermomechanical analysis and FTIR spectroscopy. They observed that the mechanical strength of the capsules decreased along with the water activity in the capsule wall during storage, and that this led to a contraction of the capsule length. Further study of the films using FTIR and PCR showed that the film contraction and water content could be adequately predicted using a calibration model. Moreover, the main peaks for the regression vector (the spectrum pattern comprising the loading vectors of the first two principal components) of the water content were observed to be similar to those for the pullulan film contraction. Thus, the results suggested that the calibration model for the film contraction could be predicted based on the model used for the water content. The compendia specifications for pullulan are similar between Japan and the United States. The pharmacopeial specifications for pullulan are presented in Table 13.5.
Test | JP XV | USP35-NF30 |
---|---|---|
Identification | + | + |
Viscosity (kinematic) | 100–180 mm2 s−1 | 100–180 mm2 s−1 |
Microbial limits | ||
Bacteria | − | <100 CFU/g |
Yeasts and molds | − | <100 CFU/g |
pH | 4.5–6.5 | 4.5–6.5 |
Loss on drying | ≤6.0% | ≤6.0% |
Residue on ignition | ≤0.3% | ≤0.3% |
Heavy metals | ≤5 ppm | ≤5 μg/g |
Content of monosaccharide, disaccharide, and oligosaccharides | ≤10.0% | ≤10.0% |
Nitrogen content | ≤0.05% | ≤0.05% |
Source: Reprinted with the permission of the American Pharmacists Association (Washington, DC) from Rowe RC, Sheskey PJ, Cook WG, Fenton ME (Eds.). Handbook of Pharmaceutical Excipients, 7th edition, 2012. Pharmaceutical Press, Philadelphia, PA.
Thermal Analysis
Changes in temperature and humidity have a significant impact on the quality as well as the physical properties (i.e., brittleness) of the gelatin capsules as water is known to migrate between the powdered excipients or the liquid fill and the gelatin shell. Modulated differential scanning calorimetry (MDSC) or conventional DSC can be used to detect imperceptible changes in the gelatin shells attributed to either external factors or formulation ingredients long before they can be confirmed using visual observation. For improved accuracy, Coleman and Craig [45] advised that at least six modulations in MDSC should be observed throughout the duration of each thermal event.
The shift in the reversible heat flow determined using MDSC is used to measure the glass transition temperature (Tg) of gelatin. During this transition, the heat capacity of the polymer is also decreased. Nazzal and Wang [46] studied various model formulations of Cremophor EL (polyoxyl castor oil) with water, alcohol, and PG for soft gelatin capsules (SGCs) and noted that such thermal events are correlated with the hardness data. The authors observed that there was a good correlation between the shift of gelatin’s Tm (unfolding or melting temperature) toward lower temperatures and the hardness loss for SGCs containing water and PG as part of the fill formulation. It has also been shown by Fitzpatrick and Saklatvala [47] that moisture uptake by an excipient sample and, therefore, moisture loss from the gelatin shell will result in an increase in the glass transition temperature of gelatin.
D’Cruz and Bell [48] studied those polyols that had a plasticizing effect on gelatin, as demonstrated by the lowering of the Tg values below that of anhydrous gelatin (157°C) (Table 13.6). Polyols with a lower glass transition temperature (e.g., glycerol) plasticized gelatin more efficiently than polyols with a higher Tg (i.e., trehalose). The glass transition temperature values of the gelatin mixtures also decreased as the polyol concentrations increased. The plasticizing effect of the polyols promotes increased molecular mobility and also changes the gelatin’s thermal properties. Moreover, the polyols also promoted a lowering of the gelatin’s Tm value. This destabilizing effect also depended on the polyol type and concentration level. The Tm values decreased with decreasing polyol Tg and with increasing polyol concentration. Thus, the polyols promote a reduction in the thermal stability of gelatin, as indicated by lower Tm values.
Polyol (Tg) | Concentration (%) | Onset Tga (°C) | Tma (°C) |
---|---|---|---|
Glycerol (−92°C) | 10 | 85 ± 9 | 117 ± 5 |
20 | 51 ± 1 | 84 ± 1 | |
30 | NDb | 69 ± 2 | |
Xylitol (−18.5°C) | 10 | 101 ± 3 | 133 ± 3 |
20 | 74 ± 3 | 111 ± 1 | |
30 | 53 ± 3 | 88 ± 2 | |
Sorbitol (−2°C) | 10 | 108 ± 5 | 131 ± 1 |
20 | 80 ± 4 | 116 ± 1 | |
30 | 58 ± 6 | 98 ± 1 | |
Sucrose (74°C) | 10 | 109 ± 7 | 142 ± 6 |
20 | 100 ± 5 | 132 ± 7 | |
30 | 86 ± 8 | 125 ± 4 | |
Trehalose (115°C) | 10 | 131 ± 1 | 161 ± 1 |
20 | 119 ± 6 | 145 ± 1 | |
30 | 101 ± 5 | 139 ± 1 |
Source: D’Cruz NM and Bell LN: Thermal unfolding of gelatin in solids as affected by the glass transition. J Food Sci. 2005. 70(2). E64–E68. Copyright Wiley-VCH Verlag GmbH & Co. KGaA. Reproduced with permission via Copyright Clearance Center.
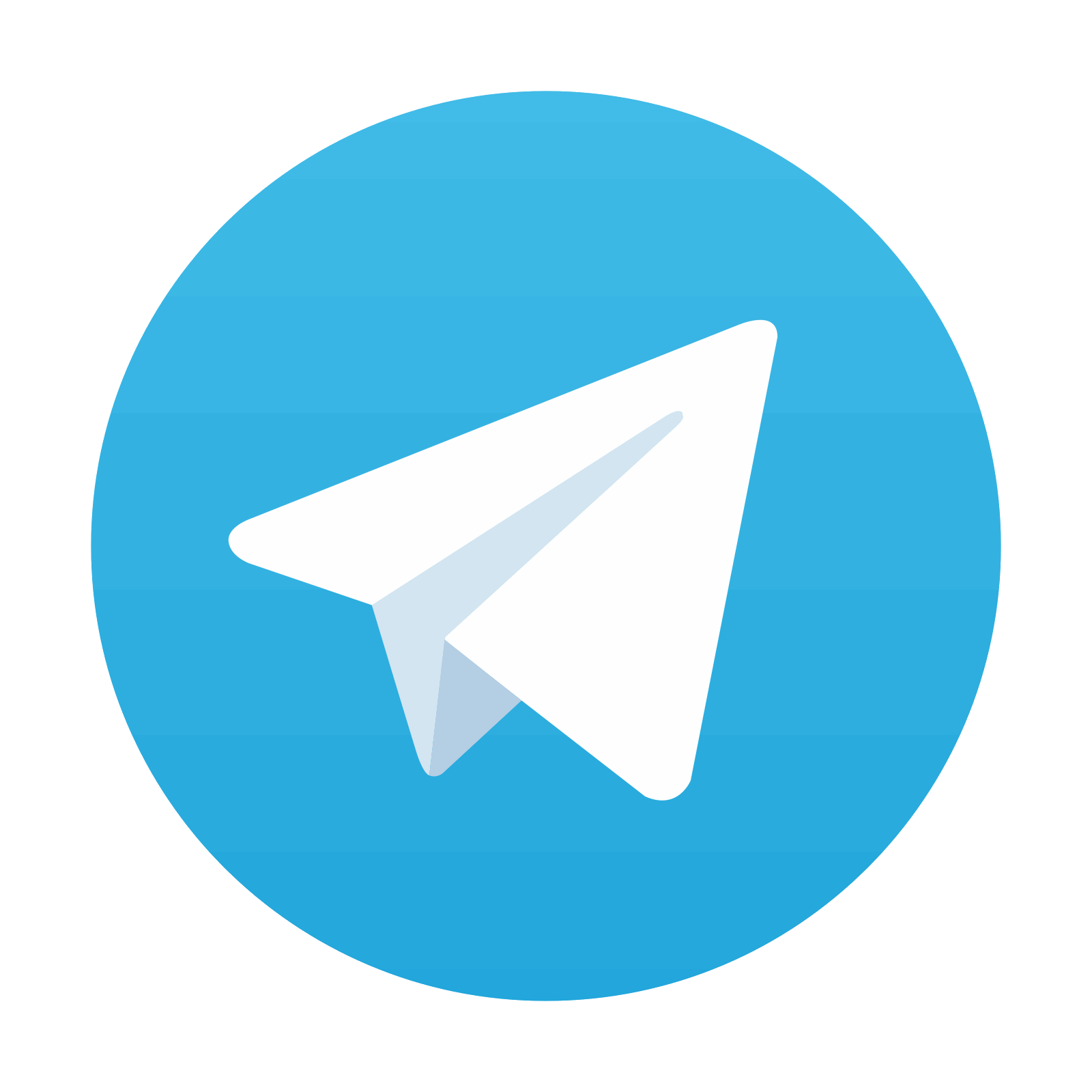
Stay updated, free articles. Join our Telegram channel

Full access? Get Clinical Tree
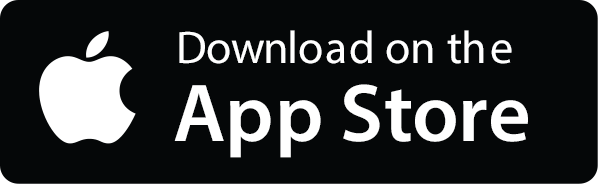
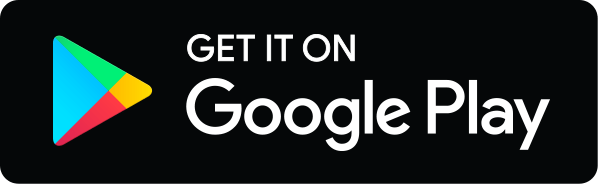
