4 Ultraviolet and visible spectroscopy
Factors governing absorption of radiation in the UV/visible region
UV spectra of some representative drug molecules
Use of UV/visible spectrophotometry to determine pKa values
Applications of UV/visible spectroscopy to pharmaceutical quantitative analysis
Applications of UV/visible spectroscopy in preformulation and formulation
Applications in pharmaceutical analysis
• A robust, workhorse method for the quantification of drugs in formulations where there is no interference from excipients.
• Determination of the pKa values of some drugs.
• Determination of partition coefficients and solubilities of drugs.
• Used to determine the release of drugs from formulations with time, e.g. in dissolution testing.
• Can be used to monitor the reaction kinetics of drug degradation.
• The UV spectrum of a drug is often used as one of a number of pharmacopoeial identity checks.
Strengths
• An easy-to-use, cheap and robust method offering good precision for making quantitative measurements of drugs in formulations.
• Routine method for determining some of the physico-chemical properties of drugs, which need to be known for the purposes of formulation.
• Some of the problems of the basic method can be solved by the use of derivative spectra.
Introduction
The interaction between radiation and matter is a fascinating area in its own right. Most drug molecules absorb radiation in the ultraviolet region of the spectrum, although some are coloured and thus absorb radiation in the visible region, e.g. a substance with a blue colour absorbs radiation in the red region of the spectrum. The absorption of UV/visible radiation occurs through the excitation of electrons within the molecular structure to a higher energy state; Figure 4.1 illustrates the nature of the transitions taking place. These transitions occur from the bottom vibrational state in the electronic ground state of the molecule to any one of a number of vibrational levels in the electronic excited state. The transition from a single ground state energy to one of a number of excited states gives width to UV spectra. Figure 4.1 shows a UV spectrum in which individual bands for different Vo to Vn transitions can be seen. Vibrational fine structure can be seen, although the bands overlap extensively; the vibrational bands themselves have width due to rotational transitions that are intermediate in energy between each vibrational transition. The relative energy of electronic:vibrational:rotational transitions is 100:1:0.01. In most molecules the vibrational behaviour is complex and the degree of overlap of the different energies of the vibrational transitions is too great for vibrational fine structure to be observed.
Factors governing absorption of radiation in the UV/visible region
Radiation in the UV/visible region is absorbed through excitation of the electrons involved in the bonds between the atoms making up the molecule so that the electron cloud holding the atoms together redistributes itself and the orbitals occupied by the bonding electrons no longer overlap. Short wavelength UV radiation < 150 nm (> 8.3 eV) can cause the strongest bonds in organic molecules to break and thus is very damaging to living organisms. It is the weaker bonds in molecules that are of more interest to analysts because they can be excited by longer wavelength UV radiation > 200 nm (> 6.2 eV), which is at a longer wavelength than the region in which air and common solvents absorb. Examining a very simple organic molecule such as ethylene (Fig. 4.2) it can be seen that it contains two types of carbon–carbon bonds, a strong σ bond formed by extensive overlap of the sp2 orbitals of the two carbons and a weaker π bond formed by partial overlap of the p orbitals of the carbon atoms. The σ bond would become excited and break when exposed to radiation at ca 150 nm. The weaker π bond requires less energetic radiation at ca 180 nm to produce the π* excited state shown in Figure 4.2. This excitation can occur without the molecule falling apart since the σ orbitals remain unexcited by the longer wavelength radiation at 180 nm. However, a single double bond is still not useful as a chromophore for determining analytes by UV spectrophotometry since it is still in the region where air and solvents absorb.
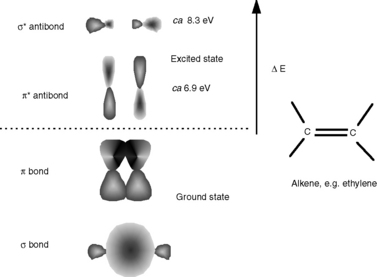
Fig. 4.2 Excitation of the carbon–carbon bonds in ethylene by radiation in the short wavelength UV region.
If more double bonds are present in a structure in conjugation (i.e. two or more double bonds in a series separated by a single bond), absorption takes place at longer wavelengths and with greater intensity, as detailed in Table 4.1 for a series of polyenes. The A (1%, 1 cm) value, which is described later, gives a measure of the intensity of absorption. The type of linear conjugated system which is present in polyenes is not very common in drug molecules.
Table 4.1 Longest wavelength maxima and absorption intensities of some polyenes
Polyene | λ max | A (1%, 1 cm) |
---|---|---|
CH3(CH = CH)3CH3 | 275 | 2800 |
CH3(CH = CH)4CH3 | 310 | 6300 |
CH3(CH = CH)5CH3 | 342 | 9000 |
CH3(CH = CH)6CH3 | 380 | 9800 |
Such extended systems of double bonds are known as ‘chromophores’. The most common chromophore found in drug molecules is a benzene ring (Table 4.2). Benzene itself has its λ max at a much shorter wavelength than a linear triene such as hexatriene (λ max 275 nm) and its strongest absorbance is at the wavelength of absorption of an isolated double bond at 180 nm. It also has a strong absorption band at 204 nm. This is due to the symmetry of benzene; it is not possible to have an excited state involving all three bonds in benzene because this would mean that the dipole (polarisation of the chromophore), a two-dimensional concept which is created in the excited state, would be symmetrical and thus would have to exist in three dimensions rather than two. There is a weak absorption in the benzene spectrum close to the λ max for hexatriene and this can occur because vibration of the benzene ring in a particular direction can distort its symmetry and thus allow all three double bonds to be involved in an excited state. If the symmetry of the benzene ring is lowered by substitution, the bands in the benzene spectrum undergo a bathochromic shift – a shift to longer wavelength. Substitution can involve either extension of the chromophore or attachment of an auxochrome (a group containing one or more lone pair of electrons) to the ring or both. Table 4.2 summarises the absorption bands found in some simple aromatic systems and these chromophore/auxochrome systems provide the basis for absorption of UV radiation by many drugs. The hydroxyl group and amino group auxochromes are affected by pH, undergoing bathochromic (moving to a longer wavelength) and hyperchromic (absorbing more strongly) shifts when a proton is removed under alkaline conditions, releasing an extra lone pair of electrons. The effect is most marked for aromatic amine groups. The absorption spectrum of a drug molecule is due to the particular combination of auxochromes and chromophores present in its structure.
Table 4.2 The UV absorption characteristics of some chromophores based on the benzene ring
Chromophore | Longest wavelength λ max | A (1%, 1 cm) |
---|---|---|
![]() Benzene | 255 nm | 28 |
![]() Benzoic acid | 273 | 85 |
![]() Cinnamic acid | 273 | 1420 |
![]() Protriptyline | 292 | 530 |
![]() | 270 nm ![]() | 72 ![]() |
![]() | 255 nm ![]() | 16 ![]() |
Beer–Lambert Law
Figure 4.3 shows the absorption of radiation by a solution containing a UV-absorbing compound.
which gives the concentration of the analyte in g/100 ml.
What are the concentrations of the following solutions of drugs in g/100 ml and mg/100 ml?
(i) Carbimazole, A (1%, 1 cm) value = 557 at 291 nm, measured absorbance = 0.557 at 291 nm.
(ii) Hydrocortisone sodium phosphate, A (1%, 1 cm) value = 333 at 248 nm, measured absorbance = 0.666 at 248 nm.
(iii) Isoprenaline, A (1%, 1 cm) value = 100 at 280 nm, measured absorbance, = 0.500 at 280 nm.
Instrumentation
A simple diagram of a UV/visible spectrophotometer is shown in Figure 4.4. The components include:
(i) The light sources – a deuterium lamp for the UV region from 190 to 350 nm and a quartz halogen or tungsten lamp for the visible region from 350 to 900 nm.
(ii) The monochromator – used to disperse the light into its constituent wavelengths, which are further selected by the slit. The monochromator is rotated so that a range of wavelengths is passed through the sample as the instrument scans across the spectrum.
(iii) The optics – may be designed to split the light beam so that the beam passes through two sample compartments, and, in such a double-beam instrument, a blank solution can then be used in one compartment to correct the reading or spectrum of the sample. The blank is most commonly the solvent in which the sample is dissolved.
Instrument calibration
Pharmacopoeial monographs usually rely on standard A (1%, 1 cm) values in order to calculate the concentration of drugs in extracts from formulations. In order to use a standard value, the instrument used to make the measurement must be properly calibrated with respect to its wavelength and absorption scales. In addition, checks for stray light and spectral resolution are run. These checks are now often built into the software of UV instruments so that they can be run automatically, to ensure that the instrument meets good manufacturing practice requirements. Some of the practical aspects of UV/visible spectrophotometry are described in Box 4.1.
Box 4.1 Practical aspects of UV/visible spectrophotometry
• Care should be taken to avoid touching the optical surfaces of sample cells with the fingers since fingerprints can cause significant absorbance. The optical surfaces of the cell can be wiped carefully with tissue.
• The precision of the pathlength of cells is important. Tolerances for cells of good quality are ± 0.01 mm for pathlength. For maximum quantitative accuracy, the same cell should be used for measurement of both the standard and the sample. The cell should always face in the same direction in a cell holder to ensure that any cell optical effects are identical for both blank and sample measurements.
• Distilled water is the ideal solvent but is not suitable for many organic compounds. Methanol and ethanol are next best but they cannot be used below a wavelength of 210 nm.
• The solvent used to dissolve the sample, concentration, pH, and temperature can affect the position and intensity of absorption bands of molecules. These factors should be controlled as far as possible. Expansion of organic solvent with temperature can cause a change in the reading, as can evaporation; thus sample cells should have tops, particularly if an organic solvent is being used.
• Ideally absorbances measured should be in the range 0.4–1.0 to avoid being outside the linear range of the instrument.
• Scattering gives an apparent increase in absorbance and is caused by particles suspended in solution. It is important that the sample solutions are free from particles.
Calibration of absorbance scale
The British Pharmacopoeia (BP) uses potassium dichromate solution to calibrate the absorbance scale of a UV spectrophotometer; the A (1%, 1 cm) values at specified wavelengths have to lie within the ranges specified by the BP. The spectrum of a 0.006% w/v solution of potassium dichromate in 0.005 M H2SO4 is shown in Figure 4.5. The absorbance scale calibration wavelengths, with corresponding A (1%, 1 cm) values for 0.006% w/v potassium dichromate solution, that are specified by the BP are as follows: 235 nm (122.9–126.2), 257 nm (142.4–145.7), 313 nm (47.0–50.3), 350 nm (104.9–108.2).
< div class='tao-gold-member'>
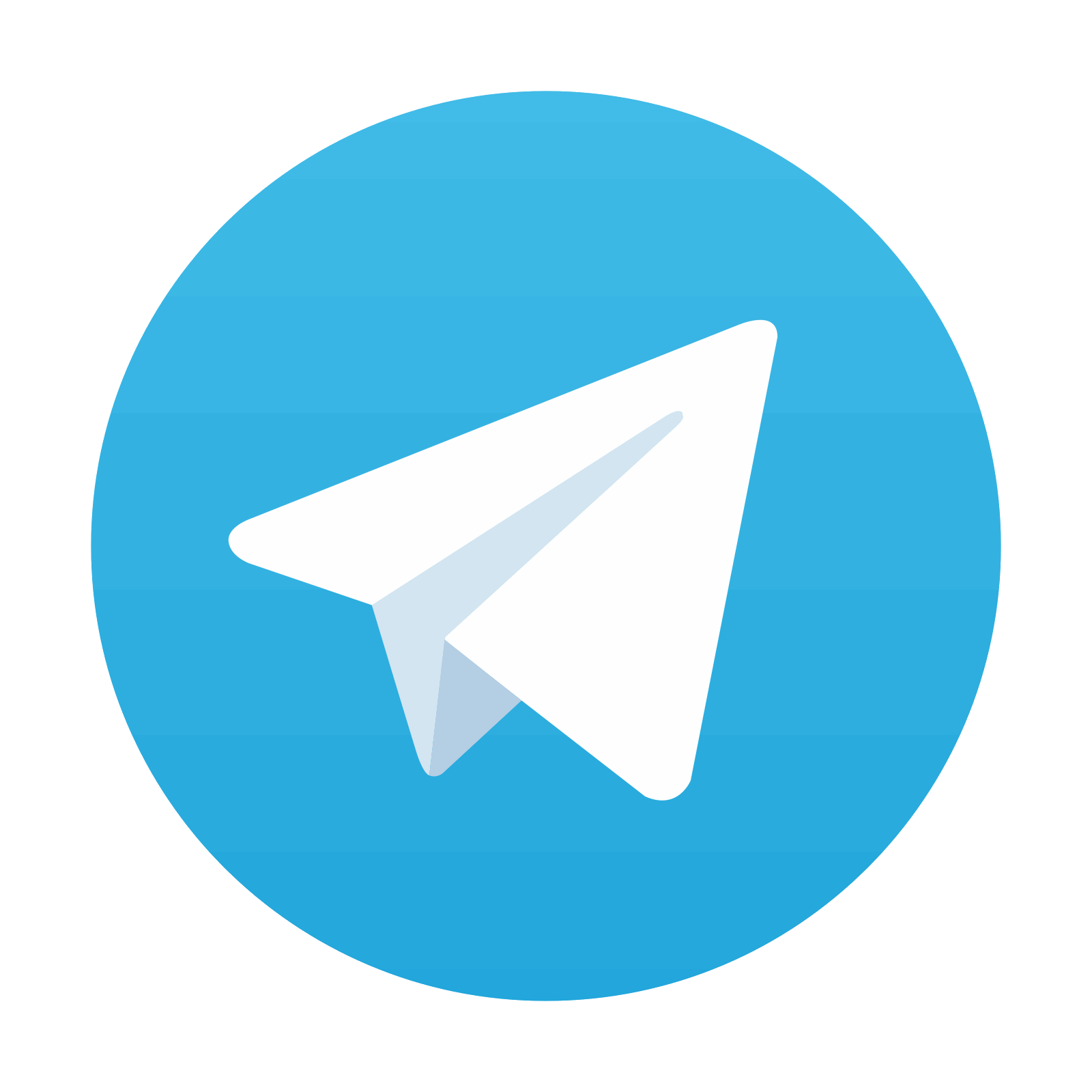
Stay updated, free articles. Join our Telegram channel

Full access? Get Clinical Tree
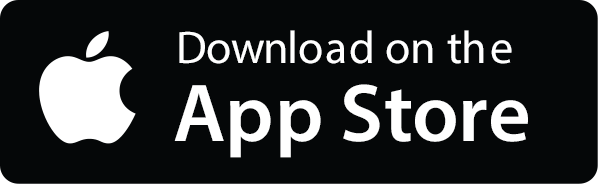
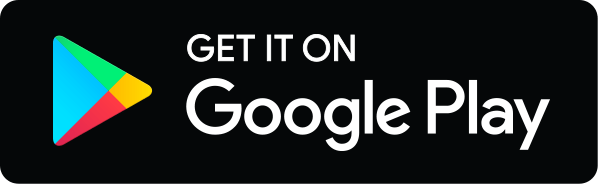