11 Christiane Müller and Andreas Bernkop-Schnürch Department of Pharmaceutical Technology, University of Innsbruck, Austria Since the term mucoadhesion appeared in the literature for the first time in 1977, the scientific research of mucoadhesive auxiliary agents has increased greatly, resulting in numerous promising ideas and strategies to achieve a more efficient delivery of therapeutic agents via noninvasive routes [1]. Mucoadhesive polymers are synthetic or natural macromolecules that are able to adhere to mucosal surfaces for a prolonged period of time due to their physical and chemical interactions with mucin molecules [2]. Most common applied polymers form noncovalent bonds (e.g. hydrogen bonds, van der Waals forces and ionic interactions) with mucoglycoproteins, leading to comparatively weak adhesion to the mucus [3,4]. Mucoadhesiveness could be significantly enhanced through covalent attachment of thiol-bearing moieties on the backbone of well-established polymeric materials. These thiolated polymers, or so called thiomers, have evolved as potential drug delivery system based on their ability to form covalent bonds within the mucus gel layer. Based on thiol/disulfide exchange reactions and simple oxidation process, disulfide bridges are formed between the cysteine-rich subdomains of the mucus and the polymer. Hence, thiomers mimic the natural behaviour of secreted mucins that are also covalently anchored in the mucus by disulfide bonds (Figure 11.1). As a result of improved adhesiveness, thiomer-based formulations remain longer on the site of absorption and improve the overall bioavailability. The use of thiolated polymers may lead to lower administration frequency, lower drug concentrations for disease treatment, targeting of particular tissues and avoidance of the first-pass metabolism [5]. Figure 11.1 Schematic representation of thiol group immobilization and mechanism of disulfide bond formation between thiomer and mucoglycoproteins. Besides the favourable mucoadhesiveness, thiomers show also improved enzyme inhibiting, efflux pump inhibiting and permeation enhancing properties as well as in situ gelling properties. Because of the multifunctional qualities of thiolated biomaterials, they are used in various fields of pharmaceutical technology, including dosage forms such as matrix tablets, gels, micro- and nanoparticles and liquid formulations [6,7]. The amended concept of mucoadhesion caused by immobilization of sulfhydryl compounds has already been demonstrated in different in vivo studies. Thiolated polymers have emerged as highly effective for the development of carrier systems for oral, nasal, ocular and buccal delivery [8,9]. This chapter provides an overview of the different thiolated polymers that have been generated so far and their synthesis techniques. Furthermore, the properties of thiomers are explained in detail, as is their biopharmaceutical use in different application forms. Since the concept of thiol group immobilization on polymers has been introduced into the pharmaceutical area, numerous derivatives have been developed and characterized. So far, a broad spectrum of polymers has been functionalized with different sulfhydryl bearing ligands via carbodiimide chemistry, periodate diformylation followed by reductive amination or via use of reactive ligands. Figure 11.2 provides an overview of the main thiolation techniques on the backbone of chitosan. The main targets for the covalent attachment of thiol compounds are functional groups on the polymeric backbone, such as amino groups on chitosan and polyallylamine or carboxylic acid moieties on poly(acrylic acid) and carboxymethylcellulose. Figure 11.2 Presumptive chemical pathways for the modification of chitosan with thioglycolic acid mediated by a carbodiimide (1-ethyl-3-(3-dimethylaminopropyl)carbodiimide hydrochloride; (a) EDAC); (b) 2-imnothiolane (B); and (c) cysteamine via periodate treatment followed by reductive amination. Reproduced with permission from [10]. Copyright © 2003 Elsevier Ltd. All rights reserved, and with permission from [17]. Copyright © 2012, Royal Society of Chemistry. In the case of chitosan, the most convenient synthesis technique is presented by usage of coupling reagents such as 2-iminothiolane (Traut’s reagent) and isopropyl-S-acetylthioacetimidate, yielding chitosan-4-thiobutylamidine and chitosan-thioethylamidine, respectively. Both chemical compounds react readily with the primary amino groups of chitosan under formation of amidine bonds via a simple one step reaction. A further advantage of this thiolation technique is based on the protection of the thiol groups based on the chemical properties of the reagents. The sulfhydryl moiety of 2-iminothiolane is located within the tetrahydrothiophene ring and the thiol group of isopropyl-S-acetylthioacetimidate is acetylated, which ensures their protection against oxidation during synthesis [10,11]. The second possibility to graft mercaptane molecules on polymeric materials is the use of carbodiimides. Compounds containing the carbodiimide functionality are applied to catalyse the formation of amide bonds by activating the carboxylic acid moiety to form an O-acylurea derivative as intermediate product that reacts with primary amino groups [12]. Hence, this method allows the immobilization of cysteine or cysteamine to activated poly(acrylic acid) as well as the attachment of activated thioglycolic acid or cysteine to chitosan [13,14]. Performing the reaction under inert conditions excludes unintended oxidation of thiol groups during the synthesis process. Alternatively, the synthesis can be conducted at a pH below five, where the concentration of reactive thiolate anions is low and the formation of disulfide bonds can be almost prevented [15]. Disulfide bonds formed during the synthesis can be cleaved by addition of reducing agents such as sodium borohydride [16] or tris-(2-carboxyethyl)-phosphine hydrochloride [17]. To improve the mucoadhesive and permeation enhancing properties and to increase the degree of thiol groups, a further thiolation technology was developed. For this purpose polysaccharides such as chitosan or hydroxyethylcellulose were oxidized by means of periodate under cleavage of their fundamental structure. The intermediate polymer was coupled with cysteamine and reduced with sodium cyanoborohydride to obtain the secondary amine moiety [18,19]. The amount of immobilized free and oxidized sulfhydryl groups can be quantified with Ellman’s reagent after reducing the entire amount of oxidized thiol groups with sodium borohydride [20]. The ratio of reduced thiols and disulfide bonds can be determined by omitting the reduction process during the Ellman’s assay [21]. The most important polymeric material for the development of cationic thiomers is chitosan. Chitosan is a natural polysaccharide derived from chitin by partial deacetylation of its acetamido groups by alkaline treatment. It has been widely used as an excipient for drug delivery systems based on its excellent mucoadhesive properties [22]. The primary amino group at the C2 position of the glucosamine subunits is readily accessible for the covalent attachment of sulfhydryl bearing ligands. Depending on the immobilized thiol compound the cationic character can be increased or lowered. In the case of chitosan–thiobutylamidine, the positive charge was improved due to the formation of the amidine group [10]. On the contrary, the grafting of chitosan with thioglycolic acid leads to an uncharged amide bond and reduces the total quantity of amino groups that can be protonated. Thiol moieties with cationic neighbour groups react to a greater extent with disulfide bonds within the mucus layer having anionic substructures [23]. According to this, chitosan–thiobutylamidine exhibits stronger adhesive properties compared to chitosan–thioglycolic acid. Besides chitosan, the cationic nonbiodegradable polymer poly(allylamine) was modified with N-acetylcysteine to develop a further positively charged thiomer [24]. Recently, a novel cationic thiolated polymer based on hydroxyethylcellulose was designed and characterized. The polysaccharide structure was altered by oxidative ring opening with periodate and reductive amination to immobilize cysteamine to hydroxyethylcellulose. This alternative thiolated cationic polymer showed similar properties to sulfhydryl tethered chitosan but was soluble in a broader pH range [18]. Anionic thiomers developed so far exhibit carboxylic acid moieties as ionic substructures, which offer the advantage that thiol compounds bearing a primary amino group can be easily immobilized to this polymer via the formation of amide bonds. Ligands as cysteamine, cysteine [13], thioaminophenol [25] and homocysteine [26] can be attached mediated by carbodiimide. The most commonly used anionic polymers are poly(acrylic acid) and polycarbophil, but pectin, alginate, carboxymethylcellulose and hyaluronic acid have also been thiolated and characterized. A disadvantage of anionic mucoadhesive polymers is their incompatibility with multivalent such as like Ca2+, Mg2+ and Fe3+, in which presence these polymers precipitate and coagulate [27], leading to a strong reduction in their bioadhesive properties. The potential of thiolated biomaterials depends markedly on the chemical and physical properties of the immobilized mercaptane bearing ligand. The pKa value of the thiol group influences the concentration of the reactive thiolate anion and determines the extent of disulfide bond formation [8]. Figure 11.3 provides an overview of chitosan and poly(acrylic acid) with aliphatic, aromatic and preactivated sulfhydryl bearing compounds. Up to now, a considerable number of thiolated polymers has been synthesized using alkyl thiol bearing compounds. Most of these aliphatic ligands, such as thioglycolic acid, N-acetylcysteine or thiobutylamidine, possess a sulfhydryl moiety with a pKa value in the range 8–10 [28]. Figure 11.3 Overview of chitosan and poly(acrylic acid) with aliphatic, aromatic and preactivated sulfhydryl-bearing compounds. Adapted from [25,28,32,55,91]. Consequently, alkyl thiolated polymers reveal the strongest mucoadhesive properties in a pH slightly above the physiological intestinal pH. Besides the pKa value, charge, lipophilicity and hydrophilicity of the attached thiol bearing ligand affect success and degree of mucoadhesive bonding. Focusing on the structure of glutathione as the aliphatic ligand, the tripeptide structure and the high negative redox potential improved the mucoadhesive features of chitosan [29]. A comparative study of chitosan with different sulfhydryl ligands showed that hydrophilic molecules with additional cationic charge increase the residence time of thiomers at mucosal surfaces [28]. Recently, the adhesive properties of thiolated polymers were improved by immobilizing aromatic thiol ligands. For this purpose, 4-mercaptobenzoic acid-functionalized chitosan was synthesized to accomplish enhanced affinity to mucin-containing surfaces. This introduced hydrophobic entity is supposed to show higher reactivity due to a low pKa (5–7) value of sulfhydryl functional groups. At intestinal pH values from 5.5–7.5 aryl thiol groups are represented in the reactive form of thiolate anions, which enhance the formation of disulfide bridges [17]. A further improvement was achieved by attachment of 6-mercaptonicotinic acid to the chitosan’s backbone. In addition to the low pH of the aromatic mercaptane, mercaptonicotinamide allows the formation of tautomeric structures, leading to a pH-independent disulfide formation [30]. Despite all the advantages of thiomers, they show comparatively low stability in solutions and gels, as they are subject of thiol oxidation at pH above six unless sealed under inert conditions. This fast oxidation of the sulfhydryl groups restricts their application in body compartments where the pH is raised [31]. Disulfide formation occurs before the polymer comes into contact with the mucus layer and diminishes the interactions between thiomer and mucus layer, thereby resulting in reduced efficacy of the dosage form. Therefore, preactivated polymers were designed and developed in order to enhance the stability, mucoadhesion and cohesive properties of thiolated biomaterials. According to this, pyridyl substructures were coupled to chitosan–thioglycolic acid mediated by disulfide bond formation. Pyridyl disulfides react very rapidly with thiol moieties over a broad pH range to form disulfide bridges. The disulfide exchange occurs between the mercaptane group and the pyridylthiol group, that is the thiomer forms disulfide bonds with cysteine-rich subdomains of mucins, with the pyridyl thiol moiety as the leaving group [32]. Mucin fibers are responsible for the structure of the mucus gel and form long flexible strings densely coated with short oligosaccharide chains. These hydrophilic and glycosylated regions are separated by hydrophobic parts of the protein that are stabilized by multiple internal disulfide bonds (cysteine-rich domains) [33]. These cystein-rich protein domains contain no potential glycosylation sites and are involved in the linking of mucin monomers with the thiol moieties of the thiomers. The resulting disulfide bond structure is supposed to be responsible for the increased mucoadhesive properties of the thiomers. The rate and the extent of disulfide formation depends on the concentration of thiolate anions representing the reactive form for thiol/disulfide exchange processes and oxidation reactions. The amount of thiolate anion is influenced by the pKa value of the thiol group, the pH of the thiomer as well as the surrounding medium. Hence, the choice of the polymeric material and the mercaptane ligand influence the reactivity of the thiomer. The properties of the thiol groups inside the polymeric network are mainly controlled by the pH of the thiomer, whereas the reactivity on the surface is more influenced by the surrounding medium. At pH values above six, a higher content of thiolate anion is available for oxidation and nucleophilic attack, which is a determining parameter in mucoadhesion [8]. Different methods have been employed to prove the existence of covalent bonds between thiolated excipient and mucoglycoproteins. Diffusion assays, gel filtration tests, rheological and mucoadhesion studies with thiomer/mucin mixtures were performed to reveal the formation of polymer–mucin conjugation. Due to the addition of disulfide breakers such as dithiothreitol the disulfide bonds could be cleaved and the immobilized thiolated polymer removed from mucin [5,34]. The second mechanism responsible for the enhanced mucoadhesion of thiolated materials is based on their in situ gelling properties. During the interpenetration process, the thiol groups oxidize at physiological pH values, which results in the formation of inter- and intramolecular disulfide bonds. These disulfide bridges are connected within the thiomer itself and lead to additional anchors by chaining up with the mucus gel layer. The mechanism can be described by penetration of the bioadhesive into the mucosal surface followed by a stabilization process of the adhesive material [8]. Rheological experiments verified the theory of the in situ gelling process. The sol–gel transition of thiolated polymers was completed after two hours, when a highly cross-linked gel was formed (Figure 11.4). Also the ascertained decrease in the free thiol group amount indicated the formation of disulfide bonds. Investigations demonstrated also a correlation between the degree of thiol groups on the polymeric backbone and the change in the viscoelastic properties of the formed gel. A high amount of mercaptane ligands leads to a significant increase in the elastic modulus [10,35]. Figure 11.4 Increase in the elastic properties (G′) of 1.5% (m/v) chitosan–TBA gel at pH 5.5 and 37 °C as a function of time. Indicated values represent the means (±SD) of at least three experiments. Reprinted with permission from [8]. Copyright (2005) Elsevier Ltd. All rights reserved. The strong mucoadhesiveness of thiomers compared to unmodified polymers has already been proven by different in vitro studies, such as those performed by the rotating cylinder and/or tensile studies. The adhesive strength of polymers is expressed either in terms of adhesion time or in terms of total work of adhesion (TWA) and maximum detachment force (MDF). In addition, the rheological behaviour of polymer/mucin mixtures can be analysed, as the increase in viscosity of polymer/mucin mixtures directly correlates with the mucoadhesion of the affected polymers [36]. Table 11.1 provides an overview of the mucoadhesiveness of the most applied anionic and cationic thiomers with their corresponding unmodified polymer. Table 11.1 Comparison of the mucoadhesive properties of various thiomer tablets and their corresponding unmodified polymer tablets. Mucoadhesion experiments were performed by tensile studies (total work of adhesion; TWA) and the rotating cylinder method (adhesion time; AT) As a result of thiol group coupling to all so far tested polymers, their mucoadhesion was significantly improved irrespective of the evaluation method. A comparative study with nineteen different, most often referred mucoadhesive excipients revealed the advantages of thiolated polymers. The rank order obtained from the adhesion time showed a prolonged residence time for the thiomers on the mucus as a result of disulfide bonds with the mucin. On the contrary, other tested polymers, such as natural polysaccharides, cellulose derivatives, polyvinylpyrrolidone and poly(ethylene glycol), showed low to almost no mucoadhesive properties. In addition, the pH of the polymer and the drying method were found to be important factors influencing the mucoadhesion of all polymers [37]. Even though thiolated polymers have shown excellent mucoadhesive qualities, the adhesion is limited due to the natural mucus turnover. Mucus is a semipermeable barrier that is continuously secreted, shed and digested, which leads also to a detachment of the dosage form [38]. One of the major obstacles for orally administered drugs is the appearance of efflux pumps in the human body. Efflux proteins located in the apical membrane may remove molecules from inside the cell back in the intestinal lumen, thus preventing their absorption. These efflux transporters are ABC (ATP-binding cassette) proteins such as P-gp and MRP2 and act as the first line of defence by limiting the absorption of toxic compounds. Although their physiological role ensures protection via detoxification, efflux proteins also reduce the bioavailability of a variety of drugs [39]. Recently, it was demonstrated by in vitro and in vivo studies that thiomers can inhibit efflux pumps. The transmucosal transport of the P-gp substrate rhodamine-123 was strongly improved in the presence of thiolated polymeric agents. In vivo studies in rats with chitosan–thiobutylamidine/glutathione tablets exhibited a threefold improved oral bioavailability of rhodamine-123 compared to the administration of this P-gp substrate given in solution. The results of this study are depicted in Figure 11.5 [40]. The mechanism of efflux pump inhibition is based on the interaction of the polymeric thiol moieties with the cysteine subunit located within the channel forming transmembrane region of P-gp. The theory is supported by the size-dependent activity of thiolated polymers and the observation that corresponding unthiolated polymers exhibit no or a negligible effect on the efflux pump activity [41]. Figure 11.5 Pharmacokinetic profile of orally administered rhodamine-123 given in solution (
Thiomers
11.1 Introduction
11.2 Thiolated Polymers
11.2.1 Thiolation Techniques
11.2.2 Cationic Thiomers
11.2.3 Anionic Thiomers
11.3 Sulfhydryl Group Contribution
11.3.1 Aliphatic Thiomers
11.3.2 Aromatic Thiomers
11.3.3 Preactivated Thiomers
11.4 Mechanism of Mucoadhesion
11.4.1 Formation of Disulfide Bonds with Mucoglycoproteins
11.4.2 In Situ Cross-Linking Mechanism
11.5 Mucoadhesive Properties
Polymer
Amount of thiol groups)μmol/g polymer)
TWA [μJ]
AT (h)
Reference
Chitosan
—
31
2.0
[37]
Chitosan–thiobutylamidine
243.4 ± 54.0
408
161.0
[37]
Poly(acrylic acid)
—
160
2.0
[55]
Poly(acrylic acid)–cysteine
404.1 ± 65.5
700
22.0
[55]
Hyaluronic acid
—
—
2.5
[92]
Hyaluronic acid–cysteine ethyl ester
201.3 ± 18.7
—
17.0
[92]
Pectin
—
—
2.5
[93]
Pectin–cysteine
892.27 ± 68.68
—
10.0
[93]
Alginate
25
—
[94]
Alginate–cysteine
340.4 ± 74.9
105
—
[94]
Polycarbophil
—
100
—
[5]
Polycarbophil–cysteine
142.2 ± 38.0
270
—
[5]
Carboxymethylcellulose
—
112
2.0
[37]
Carboxymethylcellulose–cysteine
1023.3 ± 36.0
65
7.3
[37]
11.6 Additional Properties of Thiolated Polymers
11.6.1 Efflux Pump Inhibition
) and rhodadamine-123 administered in chitosan–thiobutylamidine/glutathione tablets (
). Indicated values are means (±SD) of five experiments. Reprinted with permission from [40]. Copyright (2006) Elsevier Ltd. All rights reserved.
11.6.2 Permeation-Enhancing Effect
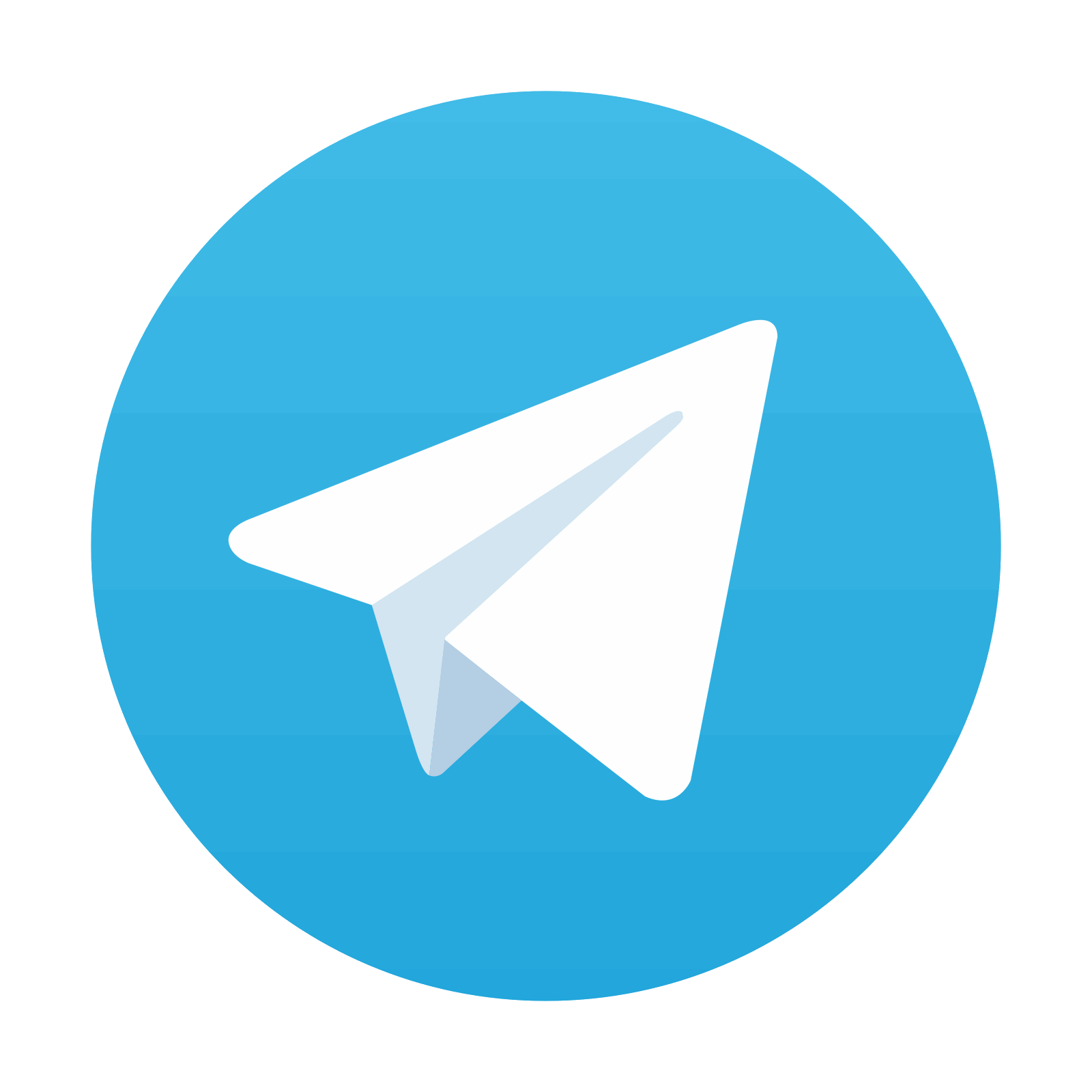
Stay updated, free articles. Join our Telegram channel

Full access? Get Clinical Tree
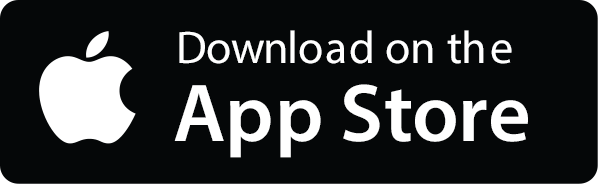
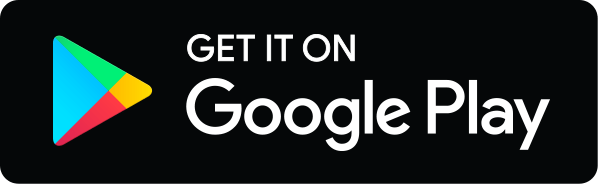