10 Joshua Boateng, Isaac Ayensu and Harshavardhan Pawar Department of Pharmaceutical, Chemical and Environmental Sciences, University of Greenwich, UK The concept of mucoadhesion has gained significant interest in pharmaceutical technology over the past two decades and might provide opportunities for novel dosage forms such as buccal delivery systems [1]. Application of mucosal drug delivery systems has increased exponentially for every conceivable route of administration because of the potential therapeutic benefits this delivery technology brings, particularly in the area of protein therapeutics. These include less frequent dosing, site-specific targeting and maintaining effective plasma concentrations without increased consumption. Bioadhesive polymers can be broadly classified into two groups, namely specific and nonspecific [2]. The specific bioadhesive polymers, such as lectins and fimbrin, have the ability to adhere to specific chemical structures within the biological molecules while the nonspecific bioadhesive polymers, such as poly(acrylic acid), polycyanoacrylates, chitosan and chitosan derivatives, have the ability to bind with both the cell surfaces and the mucosal layer. A polymer will exhibit sufficient mucoadhesive property if it can form strong intermolecular hydrogen bonds with the mucosal layer, penetrate the mucus network or tissue crevices, easily be wetted by the mucosal layer and the polymer chain has a high molecular weight. The ideal characteristics of a mucoadhesive polymer matrix include [3] rapid adherence to the mucosal layer without any change in the physical property of the delivery matrix, minimum interference to the release of the active agent, biodegradable without producing any toxic by-products, inhibit the enzymes present at the delivery site and enhance the penetration of the active agent (if the active agent is meant to be absorbed from the delivery site). Chitosan has received a great deal of attention due to its well documented degradability by human enzymes, biocompatibility and low toxicity [4]. However, unlike cellulose, the use of chitosan as an excipient in pharmaceutical formulations is a relatively new development. Chitosan is a linear aminopolysaccharide made of glucosamine and N-acetylglucosamine units obtained by the deacetylation of chitin under alkaline conditions. Chitin is a native polymer extracted from the exoskeleton of crustaceans, such as shell fish, shrimps and crabs, as well from the cell walls of some fungi; it was discovered 200 years ago [5–7]. Together with chitin, chitosan is considered to be the second most abundant polysaccharide after cellulose. Chitosan is composed mainly of (1,4)-linked 2-amino-2-deoxy-β-D-glucan [8], with the chemical name of poly[-(1,4)-2-amino-2-deoxy-D-glucopiranose]. Chitosan (Figure 10.1) differs from chitin in that a majority of the N-acetyl groups in chitosan have been deacetylated. Figure 10.1 Chemical structure of chitosan. Chitosan comes in several grades depending on the molecular weight as well as the degree of deacetylation. The degree of deacetylation has significant effects on the solubility and rheological properties of the polymer. These determine its physical and mechanical characteristics, including solubility, gel strength, mucoadhesion and sites for surface modification via reaction with the amine functional group. For example, medium molecular weight chitosan with a molecular weight of 190–310 kDa is 75–85% deacetylated. The amine functional group on the polymer has a pKa in the range 5.5–6.5, depending on the source of the polymer [9]. At low pH, the polymer is soluble, with the sol–gel transition occurring at approximately pH 7. The poor solubility of chitosan in neural or alkaline medium, however, limits its usage. The solubility in aqueous solvents of up to a pH of 6.5 is, however, attainable via the protonation of the NH2 functional group on the C-2 position of the glucosamine unit to produce soluble R-NH3+ polyelectrolyte [10]. The pH sensitivity, coupled with the reactivity of the primary amine groups, make chitosan a unique polymer for various drug delivery applications. Furthermore, its gel and matrix-forming ability make it useful for solid dosage forms, such as granules, microparticles and xerogels. Chitosan has many applications because it is the only pseudo-natural cationic polymer that is easily and readily functionalised to yield various derivatives with different functional properties. The key functional characteristics of chitosan include bioadhesion (mucoadhesion), biophysical (biomaterial), smart, permeation enhancing, hydrogel forming (swelling) and controlled (targeted) drug delivery properties. These properties make chitosan a highly versatile polymer and form the basis of its diverse applications covering pharmaceutical, agricultural, biomedical and clinical uses; these are discussed briefly here. Badawi and Rabea [11] noted that chitosan has certain major and unique characteristics that make it advantageous for various applications: ‘(i) a well defined chemical structure; (ii) easily modified chemically and enzymatically; (iii) physically and biologically functional; (iv) biodegradable and biocompatible with many organs, tissues, and cells; (v) it can be processed into several products including flakes, fine powders, beads, membranes, sponges, cottons, fibers and gels’. As noted earlier, chitosan is obtained industrially by hydrolysing the aminoacetyl groups of the natural polymer chitin. It can, therefore, be described as a natural hydrophilic polysaccharide biopolymer [12]. Chitin and its deacetylated derivative, chitosan, are nontoxic, antibacterial, biodegradable and biocompatible biopolymers. Due to these properties, they are widely used for biomedical applications such as tissue engineering scaffolds for tissue replacements, drug delivery, wound dressings, separation membranes and antibacterial coatings, stent coatings and sensors. Di Martino and co-authors [13] have noted that the interesting biomaterial characteristics of chitosan include minimal foreign body reaction, intrinsic antibacterial property and ease of fabricating into various geometries such as porous structures that lend themselves to cell growth and osteoconduction for orthopaedic purposes. They postulated that the ability to manipulate and reconstitute tissue structure and function using chitosan had significant clinical implications in cell and gene therapies in the future. Chitosan exhibits mucoadhesive properties and is often used to enhance the residence time of drugs at the mucosal membrane, thereby increasing the drugs’ bioavailability. Compared with other commonly used polymeric mucoadhesive materials of natural origin, such as cellulose, starch and xanthan, chitosan exhibits higher bioadhesive characteristics [14]. The mucoadhesion could be further improved by derivatisation of the amine functionality to form various derivatives, such as chitosan–4-thio-butyl-amidine) [15]. Different mechanisms have been proposed for the mucoadhesive attachment of chitosan to mucosal surfaces. Mucoadhesive polymers such as chitosan are generally hydrophilic networks that contain numerous polar functional groups. Such polar functional groups enable their interaction with the mucus through physical entanglements and secondary chemical bonds, with the resultant formation of weakly cross-linked networks. The key sites for mucoadhesive interactions appear to be on the carbohydrate residues, via electrostatic interaction or through hydrophobic bonding of fucose clusters [16]. It has been reported that chitosan binds via ionic interactions between primary amino functional groups and the sialic acid and sulfonic acid substructures of mucus [17,18]. It has been proposed as well that chitosan adheres by possible interaction between its hydroxyl and amino groups and mucus via hydrogen bonding. Furthermore, the linearity of chitosan molecules also allows sufficient chain flexibility for interpenetration into crevices on the mucosal surface, which results in enhanced mucoadhesive performance [19]. Various factors that can alter the interaction between the polymer and the mucosal layer can affect the mucoadhesive property of a polymer. These include polymer molecular weight [20,21], degree of derivatisation, polymer concentration [22] and method of drying. Grabovac et al. [23] reported that the method of drying is vital as it influenced the mucoadhesive potential of polymers. They showed that lyophilised thiolated chitosan produced greater mucoadhesion than others dried by precipitation in organic solvents. The addition of plasticisers to polymeric dosage forms also imparts flexibility, reduces brittleness, increases toughness and improves flow. It achieves this by interposing itself between the polymer chains and interacting with polymer functional groups to reduce interaction and intermolecular cohesive forces between the polymer chains [24]. Recently, it has been shown that the thiolation of chitosan can lead to an improvement of several properties of unmodified chitosan [25]. These thiolated chitosans have numerous advantageous features in comparison to unmodified chitosan, such as significantly improved mucoadhesive and permeation enhancing properties [26–28]. For instance, the mucoadhesive properties of a chitosan–4-thio-butyl-amidine conjugate were improved 250-fold in comparison to unmodified chitosan. The strong cohesive properties of thiolated chitosans render them highly appropriate excipients in controlled drug release dosage forms. Roldo et al. [25] also reported the effect of molecular weight and percentage thiol immobilisation on the mucoadhesive properties of chitosan and its conjugates. They concluded that mucoadhesion was increased when the level of 4-thio-butyl-amidine substructures immobilised on the polymer was greater whilst thiolated chitosan of medium molecular mass was relatively more mucoadhesive [29]. The permeation enhancing capabilities of chitosan were first shown by Illum and co-workers [30]. The use of chitosan in various studies carried out on Caco-2 cell monolayers demonstrated a significant decrease in the transepithelial electrical resistance, leading to enhanced membrane permeation [31–33]. Chitosan is able to improve the transport of hydrophilic compounds, such as therapeutic peptides and antisense oligonucleotides, across the membrane via the paracellular route. Structural reorganisation of tight junction-associated proteins, which stems from the interaction between the positive charges of the polymer and the cell membrane, results in the permeation enhancing effect [34]. However, in the presence of mucus layer, the permeation enhancing effect is comparatively low, as chitosan cannot reach the epithelium due to size exclusion and/or competitive charge interactions with mucins [35]. On the other hand, the results obtained on Caco-2 cell monolayers were confirmed by in vivo studies, showing an enhanced intestinal absorption of the peptide drug, buserelin, in rats owing to the co-administration of chitosan hydrochloride [36]. Chitosan shows strong mucoadhesive properties [37], which make it useful in drug delivery. Interaction between the protonated amino group at the C-2 position and the negatively charged sites on the cell surfaces and tight junctions allows paracellular transport of large hydrophilic compounds such as proteins by opening the tight junctions of mucosal membrane barriers [31,32,38]. This has been demonstrated by a decrease in ZO-1 proteins and the change in the cytoskeletal protein F-actin from a filamentous to a globular structure [39,40]. These observations show the potential of chitosan as a penetration enhancer of mucosal paracellular pathways, which makes it useful as a mucosal drug delivery system. It has also been found to enhance the nasal absorption of degracalcitonin and insulin in rats and sheep [41], morphine-6-glucuronide and goserelin in sheep [42]. One of the key characteristics of chitosan is its in situ gellation property [43]. In addition, chemical or physical modification (and/or combinations) allows the formation of different hydrogels with varying physicochemical properties for a wide range of applications. The swelling and gel forming behaviour of chitosan is critical, as it affects functional properties such as mucoadhesion, drug release and controlled drug release characteristics. The swelling and gelling behaviour is also dependent on the degree of acetylation (or deacetylation), pH and degree of substitution as well as cross-linking. The latter, in particular, determines its hydrogel forming ability, which ultimately affects its water holding capacity and structural integrity [44]. Chitosan hydrogels are normally obtained by use of chemicals, physical interaction or by irradiation. Rohindra et al. [45] prepared hydrogels by cross-linking chitosan with varying concentrations of glutaraldehyde and showed the swelling behaviour to be dependent on pH, temperature and the degree of cross-linking. Similarly, Singh et al. synthesised chitosan hydrogels by cross-linking with varying concentrations of formaldehyde and characterised their swelling and water absorption capacity characteristics with varying formaldehyde concentration, ionic strength, pH and temperature. The hydrogels they produced generally showed a typical pH and temperature responsive behaviour, such as low pH and high temperature has maximum swelling while high pH and low temperature showed minimum swelling. Their results showed that the hydrogels exhibited a high swelling capacity and equilibrium water content at an optimum pH of 7 and temperature of 35 °C. In addition, higher values of ionic strength resulted in decreasing the swelling of hydrogels at both low and high pH [46]. The presence of hydrophilicity, functional amino groups and a net cationic charge has made chitosan a suitable polymer for the ‘intelligent’ delivery of macromolecular compounds, such as proteins and genes. These have further been improved by derivatisation of the amine functionality, as highlighted above. The polymer is sensitive to various external stimuli, including pH, temperature, solvent, and ionic strength. The smart property of chitosan is normally achieved by various modifications to form hydrogels that respond to different external stimuli including pH, temperature and ionic strength as discussed above [47]. This is particularly significant, as it allows the versatility of chitosan and its derivatives in various biomedical, clinical and pharmaceutical applications, including tissue regeneration, controlled and targeted drug delivery, which are discussed in more detail here. Chitosan has been developed as a suitable matrix for the controlled release of small molecules and protein or peptide drugs over the last two decades [48]. Formulations with bioadhesive properties have been reported to offer certain advantages for mucosal drug delivery, including prolonged residence time, ease of application [49,50] and controlled release of loaded drug. Due to its favourable gelling properties, chitosan can deliver morphogenic factors and pharmaceutical agents in a controlled fashion. Its cationic nature allows it to complex DNA molecules, making it an ideal candidate for gene delivery strategies [51]. In most cases, controlled drug release is achieved by modifying the swelling and drug diffusion process by combining with a wide range of other synthetic, biological, ionic or nonionic polymers. For example, it is possible to combine positively charged chitosan with negatively charged equivalents, including gelatine, alginic acid and hyaluronic acid, to obtain delivery systems with properties tailored for controlled and targeted drug release [52]. The effect of chitosan properties on swelling behaviour of alginate–chitosan microcapsules was investigated by Lui and co-workers [53]. They showed that microcapsules prepared from low molecular weight and high concentration of chitosan had low swelling capacity. Microcrystalline chitosan as a gel-forming excipient for matrix-type drug granules has been studied by Sakkinen et al. [54], who observed that crystallinity, molecular weight and degree of deacetylation were the major factors that affected the release rates from the chitosan-based granules. The excipient also has promise for site-specific delivery. Tozaki et al. [55] used chitosan capsules into which was encapsulated a 5-amino salicylic acid for colon-specific delivery to treat ulcerative colitis. It was observed that chitosan capsules delivered in vivo to male Wistar rats after induction of colitis disintegrated specifically in the large intestine as compared to the control formulation (in absence of chitosan), which demonstrated absorption of the drug in the small intestines. The importance of chitosan is depicted by the wide range of applications spanning pharmaceutical, medicinal, clinical and other related industries. A number of clinical studies have reported the use of chitosan as cell scaffolds in tissue engineering, nerve regeneration tubes and cartilage regeneration [56–58]. In addition, it has been used extensively as a biomaterial, owing to its immuno-stimulatory activities, anticoagulant properties, antimicrobial and antifungal action [59] and for its action as a promoter of wound healing in the field of surgery [60]. Because of its biocompatibility and biodegradation properties [61], chitosan has been used in a variety of pharmaceutical formulations, primarily for the purpose of controlled drug delivery [62], such as mucosal [63–65], buccal [66], and ocular [67] delivery of drugs. Due to its cationic nature, chitosan is capable of opening tight junctions in a cell membrane. This property has led to a number of studies to investigate the use of chitosan as a permeation enhancer for hydrophilic drugs that may otherwise have poor oral bioavailability, such as peptides [1]. Because the absorption enhancement is caused by interactions between the cell membrane and positive charges on the polymer, the phenomenon is pH and concentration dependent. Furthermore, increasing the charge density on the polymer would lead to higher permeability. This has been studied by quaternising the amine functionality on chitosan [68]. Buccal formulations for local and systemic drug delivery have attracted significant interest due to the numerous advantages presented by the buccal route, including avoidance of gastrointestinal enzymatic degradation and hepatic first-pass metabolism. In addition, it is richly vascularised and offers good accessibility for self-medication, safety and patient compliance [3]. However, the limited absorption area, barrier properties and accidental swallowing of delivery system and salivary wash-out of dissolved drug limit the absorption of drugs via the buccal mucosa. In addition, it is necessary to use penetration enhancers due to the low permeability of the buccal mucosa to high molecular weight drugs. An ideal buccal systemic drug delivery system would, however, require intimate contact with the buccal mucosa in order to maintain its position in the mouth for a desired period of time; which can be achieved by the use of mucoadhesive polymers. Furthermore, the device itself or its components should promote the permeation of the macromolecule across the mucosa, and protect it from environmental degradation [69]. Chitosan (including the thiolated derivatives) has been identified to deliver drugs, including proteins and peptides, via the buccal route because of its mucoadhesive, penetration enhancing and peptidase inhibition properties. They have been found to enhance drug absorption through the buccal mucosa without damaging the biological system [62]. The permeability enhancing effect of chitosan was shown in an in vitro model of the human buccal epithelium [70] and in porcine buccal mucosa [71]. Chitosan possesses excellent film-forming properties [72], which may be prepared from either a solvent casting technique [73–74] or hot melt extrusion technique [75]. Due to their small size and thickness compared to tablets, films promote patient compliance and have gained significance as novel drug delivery systems in the pharmaceutical sector. They have been studied for application as mucoadhesive buccal films for local (oral candidiasis) [75] or systemic effect (insulin delivery) [76]. In their study, Abruzzo et al. [77] determined that the presence of higher chitosan amounts in chitosan/gelatine films allowed the lowest percentage water-uptake ability (235.1 ± 5.3%) and the highest in vivo residence time in the buccal cavity (240 ± 13 min). Mucoadhesive chitosan-based films, incorporated with insulin-loaded nanoparticles (NPs) made of poly(ethylene glycol)methyl ether-block-polylactide (PEG-b-PLA) have also been developed and characterised [76]. Furthermore, Cui et al. [78] have also demonstrated that chitosan films offer a unique possibility for administering insulin through the buccal mucosa route, with bioavailability reaching 17% in five hours. Another novel chitosan-based drug delivery system is freeze dried wafers (xerogels). Lyophilised wafers offer advantages over other delivery systems such as semi-solid polymer gels and solvent cast films [74,79]. Wafers can maintain their swollen gel structure for a longer period and, therefore, have longer residence time to allow for effective drug absorption. Due to their porous nature and higher surface area, wafers have a higher drug loading capacity compared to the thin and continuous solvent cast equivalent [74]. Chitosan-based drug-loaded wafers have been prepared by lyophilising aqueous gels of the polymers incorporating glycerol and d-mannitol with an annealing process to obtain elegant and mechanically strong cakes [80]. The drug-loaded chitosan-based wafers have the advantage of increased ease of hydration, mucoadhesion and, subsequently, drug release characteristics. Chitosan sponges (wafers) developed for the delivery of insulin showed that mucoadhesion properties and insulin release were related to the swelling capacity and solubility properties of different chitosan salts [70]. Attempts have also been made to formulate chitosan buccal mucoadhesive devices as tablets. Chlorhexidine diacetate buccal tablets developed with a drug-loaded chitosan and sodium alginate microsphere have been evaluated in an in vivo environment [81]. Chitosan/ethylcellulose mucoadhesive bilayered tablets for buccal drug delivery have been designed and evaluated by Remuñán-López et al. [82] The bilayered structure design loaded with nifedipine and propranolol hydrochloride as model drugs demonstrated that these devices show promising potential for use in controlled delivery of drugs through the buccal cavity. Mucoadhesive buccal patches based on interpolymer complexes of chitosan–pectin for delivery of carvedilol have been developed by Kaur and Kaur [83]. The chitosan-based mucoadhesive systems provided drug delivery in a unidirectional fashion to the mucosa that avoided loss of drug due to wash-out with saliva. Tolerability and compatibility with buccal mucosa achieved with mucoadhesive chitosan buccal formulations suggests their possible use as formulations intended for treatment of chronic diseases.
Chitosan
10.1 Introduction
10.2 Material and Physicochemical Properties of Chitosan
10.2.1 Chemistry
10.2.2 Functional Characteristics of Chitosan
10.2.2.1 Biomaterial (Biopolymer) Characteristics
10.2.2.2 Mucoadhesive and Bioadhesive Characteristics
10.2.3 Factors Affecting Mucoadhesive Performance
10.2.4 Permeation Enhancing Effect
10.2.5 Swelling and Hydrogel Behaviour
10.2.6 Smart Properties
10.2.7 Controlled and Targeted Drug Delivery
10.3 Applications
10.3.1 Chitosan-Based Mucoadhesive Matrix Formulations – Case Examples
10.3.1.1 Buccal Mucosa Systems
10.3.1.2 Wound Dressings
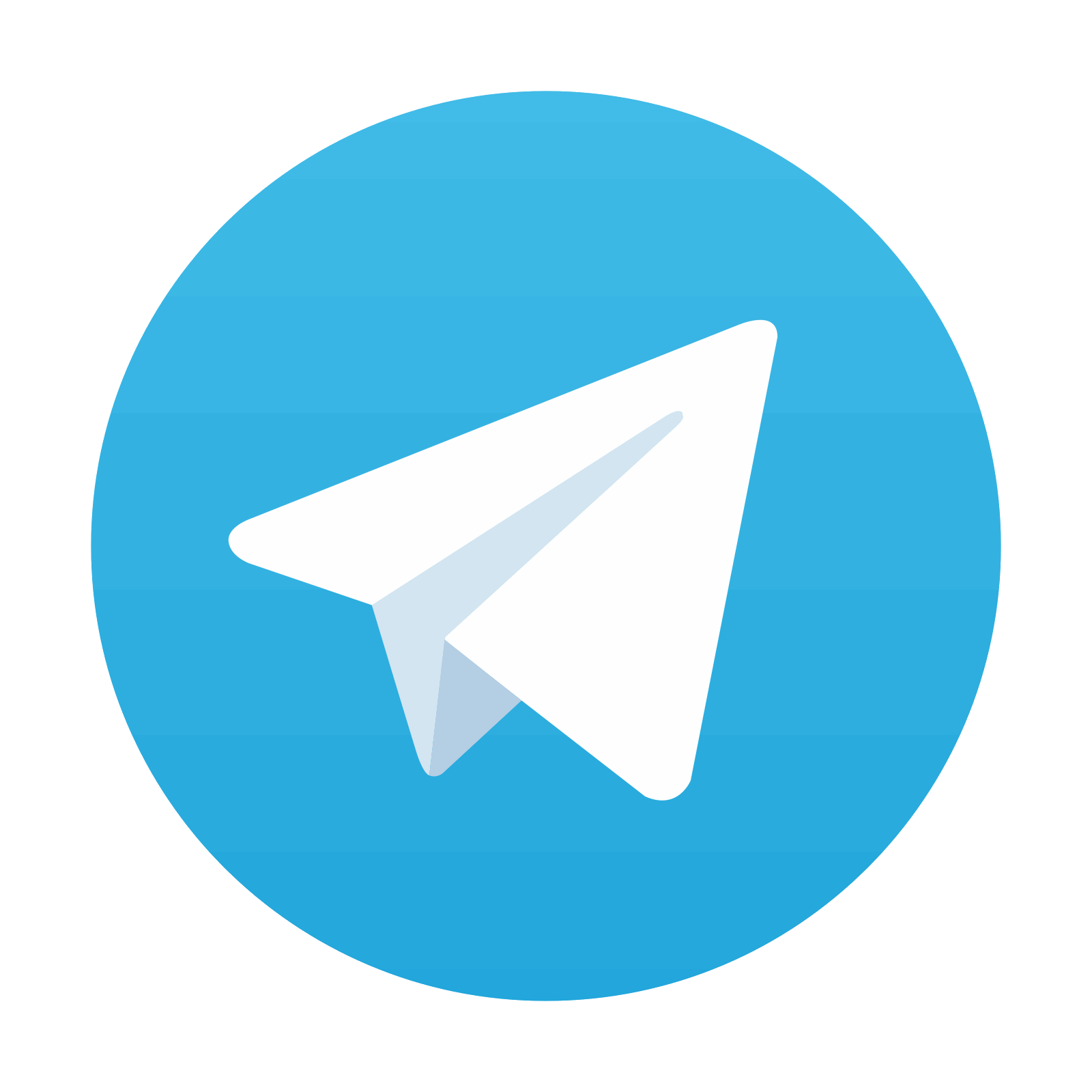
Stay updated, free articles. Join our Telegram channel

Full access? Get Clinical Tree
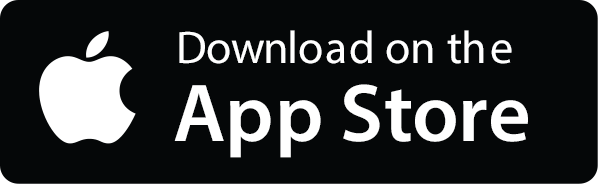
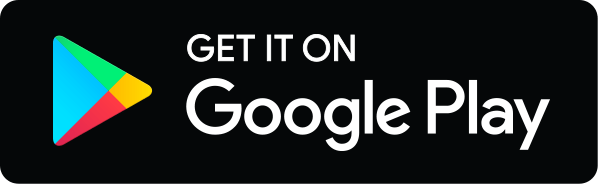