2 Peter W.J. Morrison and Vitaliy V. Khutoryanskiy Reading School of Pharmacy University of Reading UK The eye has evolved into a complex and delicate organ, the function of which is to convert light that focuses on the retina into electrical signals. The information from the retina is transmitted to the visual cortex of the brain along the optic nerve. Processed by the brain these signals are interpreted as a visual representation of the world, seen through the eye as our window into the environment that we are part of. As a whole, the eye gives us information about what is going on around us, images, a sense of distance, colour and movement. Visual perception allows a being to interact with the environment unlike any of our other senses. This function happens ‘in the background’, that is, it happens in a way that we as organisms take for granted, almost unconsciously. The various components work together and in the main function remarkably well considering the complexity and delicate nature of this visual apparatus [1,2]. Sight is considered by many to be the most feared of our senses to lose; this fear is termed ‘scotomaphobia’ [3–5]. Treating ocular disorders presents many problems for drug delivery. Firstly, topically applied drugs are subject to dilution by tears and pre-corneal loss; around 75% of the instilled dose either spills over the eyelids or is rapidly lost via naso-lacrymal drainage. Next, it is subject to nonproductive losses, where the drug is absorbed by conjunctival tissue surrounding the eye, from which it enters systemic circulation and is subsequently eliminated. Any remaining drug has to traverse the tear film and mucus barrier at the cornea surface. Finally, the cornea is a very efficient barrier with its lipophilic epithelium and hydrophilic stroma. Developing formulations with sufficient drug concentration that can remain on the eye long enough to be effective is very challenging to ocular scientists [6]. There are three main routes for administering ocular medication: topical, intraocular and systemic; each has advantages and disadvantages. The preferred means for ocular drug delivery is via the topical route, due to ease of access and patient compliance. Ocular mucosa plays an important role in drug delivery and is discussed in later sections. Recent advances in ocular drug delivery have established methods to improve ophthalmic drug retention and enhance corneal permeation as well as the development of controlled and sustained release systems [7]. The eyes are accommodated within the orbits, eye sockets deeply embedded inside the front of the skull, ensuring a high degree of physical protection. The eye is a specialised organ with individual structures that work together for the purpose of capturing visual information, transmitting this along the optic nerve to be processed by the visual cortex of the brain [8]. Figure 2.1 shows the main individual components of the eye. Some of these structures and associated tissues are described more fully in the following sections. Figure 2.1 Anatomy of the human eye. Reproduced with permission from BioGraphix (copyright 2006 BioGraphix, LLC @ www.BioGraphixMedia.com) [9]. The internal components of the eye are held within tough membranes known as ‘tunica fibrosa oculi’; these tissues are subject to internal pressure of 13–19 mm Hg, necessary in maintaining the correct shape of the body. The outer membranes encase and protect its contents and consist of the transparent cornea (anterior portion, 17%), extending forwards relative to the main body of the organ, and the highly vasculated and fibrous opaque white sclera (posterior portion, 83%) [5]. A ring of tissue where the cornea and sclera meet is known as the limbus; here the cornea thickens before making the transition to the scleral membrane [10]. The limbus is populated with stem cells responsible for regeneration of the epithelium, necessary due to its fast cell turnover rate [1]. The cornea is a transparent avascular multilayered membrane (Figure 2.2) and historically five layers are described (epithelium, Bowman’s membrane, stroma, Descemet’s membrane and endothelium), each have specific properties. More recently, Dua et al. published a report of their discovery of an additional layer between the Descemet’s membrane and the stroma, named the ‘Dua’s layer’, which is a tough membrane ∼15 μm thick that is impervious to air [11]. Figure 2.2 Micrograph showing a cross-section of the multilayered structure of bovine cornea. Scale bar = 100 μm. The cornea is nourished by the aqueous humour from inside the eye and is cleansed, lubricated and oxygenated by mucus and the tear film at the outermost surface. Further metabolic support is provided from limbal capillary blood vessels, although these vessels do not normally spread into the cornea unless it becomes starved of oxygen for an extended length of time [1,5]. The epithelium is the external physical layer, a lipophilic tissue that offers around 90% impediment to hydrophilic drug penetration and 10% to hydrophobic drug formulations. The central region of the epithelium is ∼50 μm, thickening to ∼100 μm towards the limbus [12]. The epithelial membrane consists of three types of cells: basal, polygonal and squamous. New cells are generated from limbal stem cells at the basal layer, where they have column-like shape, as they develop; older cells are pushed forwards, changing shape in the process. When intact, the epithelium is highly impermeable to aqueous solutions due to its superficial layers having a closely packed order with gap and tight junctions that serve to prevent the ingress of foreign matter [13–15]. However, this barrier is compromised when the superficial cells that make up the epithelium are disrupted, although in a healthy eye an epithelial layer is quickly regenerated. Epithelial repair occurs initially by migration of cells from nearby regions, followed by a proliferative phase resulting in the eventual regeneration of normal epithelial structure [1]. The epithelial surface is further protected by a mucus layer anchored to microvilli attached to the superficial epithelial cells. This mucosal film affords an additional barrier function against foreign matter. The epithelial surface is protected, nourished, wetted and washed by a continuously replenished tear film, which also serves to smooth out any topographic irregularities, ensuring an optically clear window to transmit incident light towards the retina [5]. An important function of the epithelium that is often overlooked is the protection it offers against ultraviolet radiation. Epithelia have high concentrations of tryptophan residues and ascorbate, which are considered important in absorbing UVR [16]. Epithelial cells absorb UV to varying degrees depending on its intensity and wavelength. Cell apoptosis follows irradiation beyond the threshold limit and a maximum irradiance of 3.0 mW cm−2, corresponding to a dose of 5.4 J cm−2 at the corneal surface, is considered safe to prevent UVR penetration to the endothelium and beyond [17–19]. Endothelial cells are continuously regenerated, ensuring maintenance of this protective barrier. Deeper structures within the eye are safeguarded from UV damage due to its absorbance at the epithelium. Ultimately, cell death follows when exposure exceeds a threshold that can be tolerated. Rapid cell turnover ensures continuous replacement of older superficial epithelial cells. It is necessary to understand the importance of this function when faced with a treatment option that compromises this protective layer, for example, when considering photorefractive surgery (laser treatment) and corneal UV ‘A’ collagen cross-linking [20]. At the very least, measures must be taken to minimise exposure to strong light sources and daylight during the healing process; effective UV absorbing eyewear should be worn during this period [16–20]. The Bowman’s membrane forms a transitional layer towards the stroma. This structure is not considered to be a barrier to drug diffusion. It is a homogenous, acellular form of ∼8–14 μm thickness, this structure does not regenerate if it is damaged [8,10]. The stromal substantia propria makes up the main portion of the corneal thickness at around 90%; it consists of a hydrophilic gel made up of collagen fibrils, proteins and mucopolysaccharides, and contains between 75 and 80% water w/w. Consisting of lamellae packed loosely and parallel to the surface, interspersed with corneal corpuscles, a cell type that maintains this layer by generation of new collagen. The stroma is an aqueous environment through which hydrophilic compounds can readily diffuse. Due to its hydrophilic gel structure, the stroma is a resistant barrier to lipophilic compounds [5,8,10,21]. Further, it is an extremely sensitive tissue owing to a high density of nerves throughout [22]. In human corneas an extra membrane has recently been discovered by Dua et al., now known as the Dua’s layer [11]. Next there is another acellular layer, at around 6 μm thickness, the Descemet’s membrane is a tough homogenous and resilient membrane that supports the endothelium, which is also responsible for secreting this membrane. Normally the Descemet’s membrane is under tension due to pressure imposed by the aqueous humour; this force maintains the curvature of the cornea [5,8,10]. The innermost layer of the cornea is the endothelium, a single loose covering of flat epithelial like cells whose function is to allow the permeation of nutrients and to maintain the hydration of the stroma via a bicarbonate dependent Na+/K+ -ATPase pump. The correct level of hydration is important to maintain transparency of the cornea and to avoid oedema (swelling due to excess fluid) [8,10]. Maurice and Giardini measured the average thickness of human cornea and found it to be 0.5070 ± 0.0042 mm, determined from both eyes of 44 volunteers [23]. Whilst the mean value quoted by Bahr is given as 0.5650 ± 0.0042 mm, this study was based on a larger data set from 125 subjects [24]. Further protection is offered by a mucosal membrane, the conjunctiva. At the anterior surface of the eye is the bulbar. Here the membrane is transparent and avascular in the corneal region and loosely attached to the sclera beyond this. The vascularised palpebral conjunctiva lines the posterior surface of the eyelids. Between the bulbar and palpebral membrane there are loose bridges of tissue, known as the superior and inferior fornices, forming the conjunctival sac, which provides a convenient depository that can be exploited to act as a reservoir for instilled medication or the placement of drug loaded ocular insert [10,25]. Figure 2.3 shows conjunctival tissue of a human subject, clearly demonstrating the loose nature of tissue in this region, which allows the organ to move within the eye socket, and for the eyelids to close over the eye. Figure 2.3 Conjunctival tissue showing the superior and inferior fornices. Around 60% of the eye’s refractive power is provided by the cornea; however, this refraction is fixed and the main focusing action of the eye is provided by the crystalline lens. The shape, hence focusing power of the lens, is controlled by the ciliary muscles. The lens hardens, becomes less elastic and takes on a yellow hue with advancing age; these effects result in a reduction of colour perception and ability of the lens to accommodate between near and far vision [5,26]. Immediately behind the cornea is found the anterior chamber; this structure houses the iris and aqueous humour, a clear aqueous fluid similar to blood plasma. With less protein, aqueous humour contains higher concentrations of ascorbate, pyruvate and lactate, and lower levels of glucose and urea than blood plasma. The aqueous humour maintains the pressure within the eye and is secreted into the posterior chamber by the ciliary body. Aqueous humour is continuously replenished, leaving the chamber via the trabecular meshwork and is drained through the canal of Schlemm [5,27]. Slight resistance offered by the drainage mechanisms ensures normal intraocular pressure is maintained at ∼16 mm Hg in a healthy subject; pressures greater than 21 mm Hg are associated with ocular hypertension. A hypertensive state can be painful and often leads to glaucoma, which can lead to permanent damage to the retina if left untreated [8]. Therapeutic treatments for ocular hypertension are mostly targeted at reducing production of aqueous humour, which subsequently leads to a reduction of pressure from within the eye [5]. Situated between the anterior chamber and the posterior chamber is the iris, which acts as a control to the intensity of light entering the eye; the iris also responds to our emotional state and vigilance. This component is part of the uveal tract, which also encompasses the choroid and ciliary body. These structures reside between the external ocular membranes and the retina in the posterior chamber, although it extends into the anterior chamber and can be seen as the coloured part of the eye [5]. The uveal tract is highly vasculated and is responsible for nourishment and metabolic processes. One of the more important functions of the uvea is to offer a ‘systemic to ocular barrier’ known as the blood–eye barrier (BEB), important in keeping the light sensitive pathways transparent [10]. The main body of the organ holds the vitreous humour occupying ∼80% of the internal volume of the eye at around 4 ml. An avascular and optically clear structure consisting of collagen, hyaluronic acid, polysaccharide and vitrosin, the largest component being water at ∼98%. Behaving similar to a gel-like body, molecular movement throughout this body is driven by diffusion rather than fluid movement. However, the vitreous humour becomes less viscous as a person ages and behaves more like a liquid than a gel at this stage. The vitreous humour keeps the retina in place against the choroid, a vascular membrane between the retina and the sclera [5,10,28]. Positioned between the aqueous chamber and vitreous body is the crystalline lens, which allows for accommodation between near and far vision. For distance vision the ciliary muscles relax and the lens becomes less convex. Conversely, for near vision the ciliary muscles ‘tense’ and the lens becomes more convex. The optical clarity of the lens is due to the precise alignment of a single cell type consisting of soluble protein fibres. It is contained within the capsular bag, which itself is attached to the ciliary body. Lens fibres are not regenerated if damaged and are, therefore, subject to age or disease-related degeneration; the structure becomes less elastic and takes on a yellow hue as a person becomes older, leading to less perception of colour and a gradual loss of the ability to accommodate between near and far vision. The most common cause of ‘loss of visual quality’ is disease causing the formation of cataracts [5,8,26,29]. Known as the ‘tunica vasculosa oculi’, the choroid forms a vascular network around the back of the eye that includes the ‘iris’, ‘ciliary body’, covering the inside of the sclera between the sclera and retina. The main function is to provide nourishment to the retina, ciliary body and iris [27]. Membranes within the vascular network are special in that they offer a ‘blood–eye barrier’ to prevent ingress of substances that can compromise the clarity of the light transmitting pathways [10]. Adjacent to the choroid and kept in place by pressure from the vitreous body lies the ‘retina’, a membrane of highly specialised nerve tissue continuous to the optic nerve. It forms a link to the visual cortex, a specialised region of the brain dedicated to visual processing. The purpose of the retina is to convert light falling upon it into visual signals interpreted by the brain as images of the outside world [2]. The retina is a complex multilayer structure ∼0.5 mm thick lining the posterior inner surface of the eye, extending towards the ciliary body; the extent of coverage is shown in Figure 2.1. Specialised photoreceptor cells are embedded throughout the retina consisting of cones and rods. Cone cells are associated with colour perception, whilst rod cells detect light monochromatically and are sensitive even at low light levels; these are of particular importance in peripheral vision. There are three types of cone cell type acting as photoreceptive transducers employing the photosensitive molecule, rhodopsin, which respond to the primary colours, red, blue and green. Colour perception is mostly concentrated at or near the macula, whilst monochromatically sensitive rod cells are dispersed throughout the retina. Retinal nourishment and metabolic maintenance are regulated by the retinal pigmented epithelium (RPE), which itself is part of the blood–retina barrier (BRB); the RPE and BRB serve to regulate solute movement to the retina and into the body of the eye [5,28]. The ocular surface includes mucosal tissue, the conjunctiva; this membrane contains various glands responsible for secreting fluids important in maintaining optimal conditions at the ocular surface. These secretory bodies and their function are discussed in more detail in this section. The lacrimal glands are located behind the superior tarsus (upper eyelid) at the upper and outer orbital quadrant. They are responsible for secreting the major portion of pre-corneal tear fluid, an aqueous fluid of low viscosity which continuously bathes the anterior surface of the eye and drains via the nasolacrimal ducts [21]. Tears are important to the healthy functioning of the eyes and have several purposes: The ‘tearing’ (lacrimation) function is autonomous and continuous action. Tearing also responds to stimuli, for example, an irritation or foreign body, whereby tear flow often overwhelms drainage through the nasolacrimal duct and overflows the eyelids in an attempt to wash out the irritating substance [5,10,21]. The tear film consists of three layers ∼7 μm thick in total (Figure 2.4). It has a superficial outer layer, ∼0.1 μm thick, that is lipid rich and helps to prevent evaporation of the aqueous content [21]. This layer is composed of waxes, cholesterol esters, triacylglycerols, free sterols, sterol esters and free fatty acids; these lipids are secreted by the Meibomian glands, glands of Zeis and glands of Moll of the upper eyelids [10,30]. The main bulk of the tear fluid is aqueous with free mucin throughout. Aqueous secretions are serviced by the lacrimal glands of the upper fornix [5]. The layer in contact with the surface of the eye consists of mucus adsorbed with the aid of microvilli. Mucus secretions emanate from the goblet cells of the upper and lower tarsus. Mucus aids the adhesion of the aqueous fraction to the surface of the conjunctiva [10]. Figure 2.4 Three-layer tear film model showing mucin, aqueous and lipid fractions. Average tear volume is ∼7 μl, with ∼1 μl occupying the pre-corneal tear film and the remainder occupying tear region margins. A maximum tear volume of ∼30 μl can accumulate prior to blinking and the upper and lower fornices can accommodate ∼10 μl of fluid. The pH of tear fluid fluctuates between 7.0 and 7.4, mostly influenced by the bicarbonate–carbon dioxide buffer system, with pH increasing through loss of carbon dioxide when the eyes are open, and acidic interactions operating when closed [5,10]. Tear, mucin and lipid producing glands are abundant in the ocular mucosa (Figure 2.5); most are situated in the superior tarsus (upper eyelid) and inferior tarsus (lower eyelid), with the highest density associated with the palpebral conjunctiva. Aqueous components of tears are supplied by lacrimal glands, glands of Krause and glands of Wolfring, whilst mucin is generated by Goblet cells, glands of Manz and Crypts of Henle. Glands responsible for secreting lipid are at the terminal boundaries of the superior and inferior tarsus, namely, Meibomian glands, glands of Moll and glands of Zeis [10]. Figure 2.5 Location of aqueous, mucin and lipid secreting glands of ocular mucosa. Tears and mucin are spread across the eye surface by the action of blinking, offering a means to keep the ocular surface smoothed and hydrated. Elimination is mostly via the nasolacrimal tract aided by a ‘pumping action’ due to pressure changes upon opening and closing the eyelids; however, in the event of severe irritation or intrusion by a foreign body, a reflex tearing action overwhelms lacrimal drainage and tears overspill the eyelids in their attempt to wash out the foreign matter [21,31].
Anatomy of the Eye and the Role of Ocular Mucosa in Drug Delivery
2.1 Introduction
2.2 Anatomy of the Eye
2.2.1 Outer Membranes; Conjunctiva, Cornea and Sclera
2.2.2 Aqueous Chamber, Lens and Vitreous Body
2.2.3 Choroid and Retina
2.3 Introduction to Ocular Mucosa
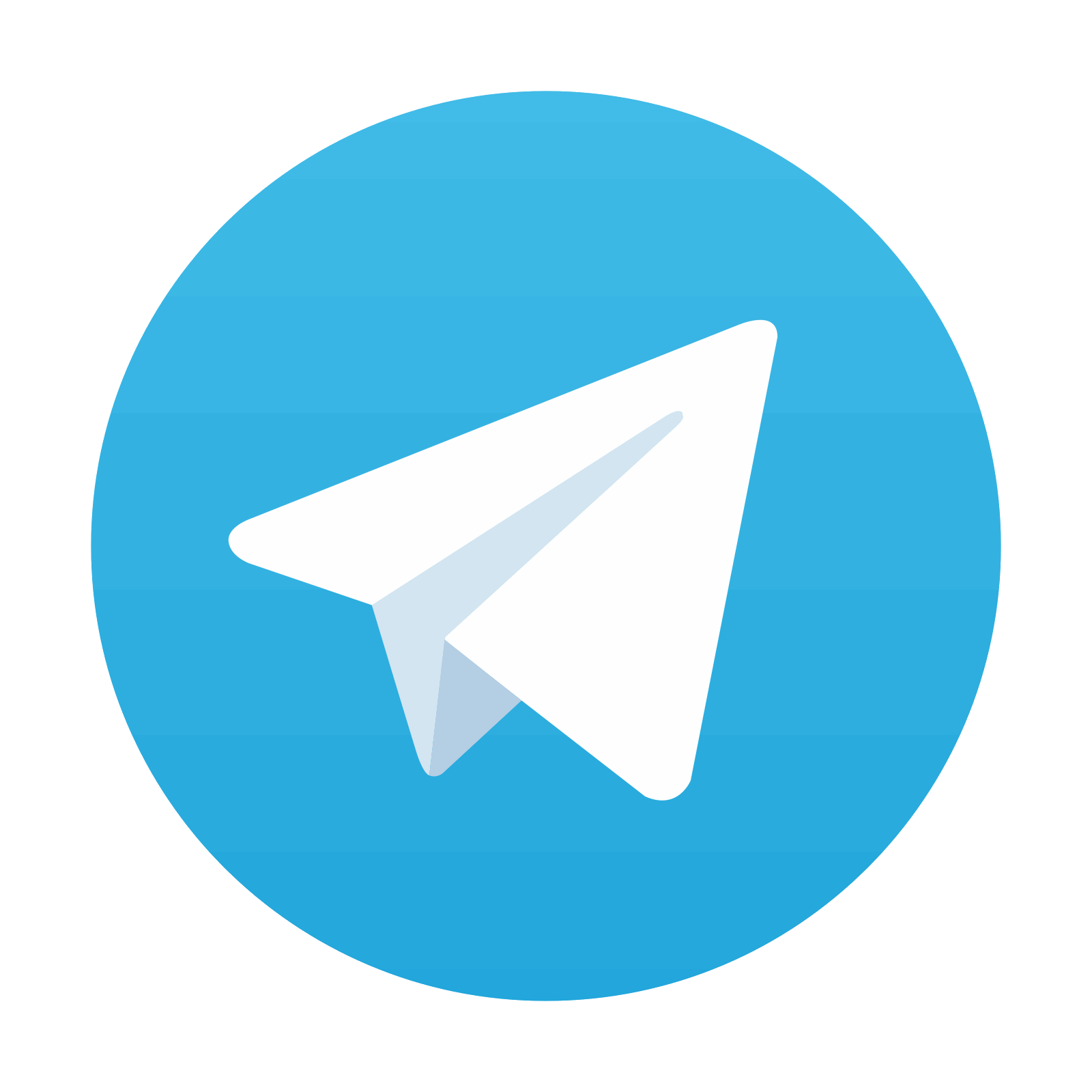
Stay updated, free articles. Join our Telegram channel

Full access? Get Clinical Tree
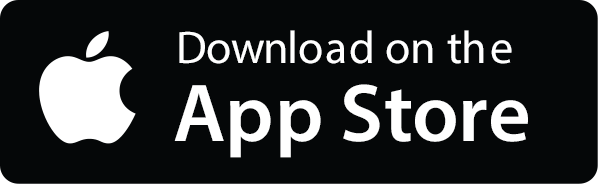
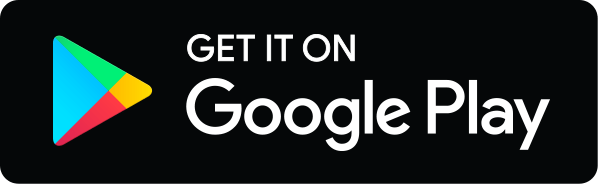