Author
Method
Results
Property of tantalum demonstrated
Bobyn et al.
Implantation of 22 acetabular porous tantalum components in 11 dogs
Thin section histology, high-resolution radiography, and backscattered scanning electron microscopy revealed that all 22 implants had stable bone-implant interfaces at the end of 6 months
Bone ingrowth
Hacking et al.
Eight dorsal subcutaneous tantalum implantation in two dogs evaluated at 4, 8, and 16 weeks
Analysis of retrieved specimens revealed fibrous tissue ingrowth and blood vessels in porous tantalum implants
Fibrous ingrowth
Rahbek et al.
Implantation of tantalum and glass bead-blasted titanium alloy into the knee joints of eight mongrel dogs in randomized paired design. Weekly polyethylene particle injection into the knees starting at three weeks postimplantation
Superior bone ingrowth and less polyethylene particle migration into porous tantalum compared with glass bead-blasted implants at 8 weeks
Resistance to migration of polyethylene particles
Reach et al.
Supraspinatus tendons from forty skeletally mature dogs were reattached to the greater tuberosity between two porous tantalum washers, and the reattachment evaluated postoperatively for strength, stiffness, and tendon function
Excellent biologic ingrowth of the tendon into porous tantalum was observed. Histomorphologic evaluation revealed Sharpey-like fibers inserting onto the surface of the porous tantalum washers
Direct healing potential of tendons to porous tantalum biomaterial
The same team studied patterns of bone ingrowth into porous tantalum subjected to normal loading. They implanted acetabular monoblock tantalum components in a canine model and examined the bone-implant interface radiologically and histologically after 6 months. Stable implant fixation and bone ingrowth were found in all cases. The depth of bone ingrowth varied from 0.2 to 2 mm, comparable to titanium fiber mesh [22, 23]. The bone ingrowth was intense and pronounced at the periphery of the implant due to uniform bone-implant contact. Interestingly, fibrous tissue formation was noted in pores devoid of bone. This phenomenon may serve as a barrier to wear debris and potentially avoid or minimize wear particle-induced osteolysis. Tantalum serves as a good substrate for growth and differentiation of fibroblasts as well. Hacking et al. evaluated eight dorsal subcutaneous tantalum implants in two dogs at 4, 8, and 16 weeks [19]. The retrieved specimens revealed reliable fibrous and vascular tissue ingrowth into the porous tantalum implants.
The bone ingrowth potential of porous tantalum can be enhanced with application of various surface coatings. Garbuz et al. compared bone formation into porous tantalum implants coated with calcium phosphate and alendronate with uncoated implants using an electron microscopy in eighteen rabbit femora [24]. They observed significantly enhanced bone ingrowth and filling of the bone-implant gap with the coated porous tantalum implants, thus illustrating that chemical surface treatment of porous tantalum may further improve its biological performance.
10.4 Bone Ingrowth in Retrieval Studies
There has been encouraging data from studies of retrieved tantalum implants (Table 10.2). D’Angelo et al. studied an acetabular porous tantalum component removed for recurrent hip dislocation 3 years after index surgery [25]. Bone ingrowth was assessed with light microscopy, and a geometrical model was used to obtain quantitative evaluation of bone ingrowth. Their analysis revealed that more than 95 % of the voids on the implant were filled with bone. The histological findings were consistent with the radiological findings of complete osseointegration of the implant. In a recent multicenter study, Hanzlik et al. investigated the amount of bone ingrowth in retrieved porous tantalum components [26]. They analyzed bone volume fraction, extent of ingrowth, and maximum depth of ingrowth in 76 acetabular shells, 5 femoral stems, 7 patellas, and 36 tibial trays. They noted consistent full depth bone penetration into the porous tantalum layer in the acetabular shells and femoral stems. In another study, Sambaziotis et al. reported a histologic retrieval analysis of a porous tantalum tibial component in an infected total knee arthroplasty [27]. They observed bone ingrowth along tibial baseplate and the post, which was suggestive of viable bone tissue and healthy marrow, osteocytes, and lamella despite of the presence of infection. However, the bone ingrowth potential of porous tantalum may vary in different clinical scenarios. Tanzer et al. reported histopathologic retrieval analysis of failed porous tantalum rods used for treatment of hip osteonecrosis [28]. The retrieved implants were associated with little bone ingrowth and insufficient mechanical support of the subchondral bone. They suggested that the failures were probably multifactorial. They recommended close monitoring of implant design, surgical technique, and appropriate patient selection to avoid failures. Similarly, Breer et al. investigated the osseointegration pattern into porous tantalum augmentations harvested during re-revision after hip and knee replacement procedures [29]. They noted limited to no growth in 5/7 specimens. They attributed suboptimal bone ingrowth to poor direct implant to host bone contact secondary to the use of interposing cement or bone graft. Although porous tantalum has demonstrated excellent bone ingrowth potential, an adverse biological environment may influence the clinical performance of these implants.
Table 10.2
Retrieval studies of porous tantalum following hip and knee reconstructive surgery
Author | Components removed | Indication for removal | Characteristics of bone ingrowth |
---|---|---|---|
D’Angelo F et al. | One acetabular component | Recurrent hip dislocation | 95 % bone ingrowth |
Hanzlik et al. | 76 acetabular shells, five femoral stems, seven patellas, and 36 tibial trays | Infection [tibial trays], instability [acetabular], and loosening [two acetabular shells, one tibial tray] | Full depth penetration of bone into the porous tantalum layer for the acetabular shells and femoral stems |
Sambaziotis et al. | One tibial component | Infection | Bone ingrowth present in the tibial posts (36.7 %) and 4.9 % into the tibial baseplate |
Tanzer et al. | 15 tantalum rods | Progression of osteonecrosis | Bone ingrowth in thirteen (87 %), extent of bone ingrowth was 1.9 % |
Breer et al. | Four hip and three knee tantalum augments | Two fractures, two loosening, and three infections | No bone ingrowth 5/7, 2.6 mm bone ingrowth in 2/7 specimens |
10.5 Clinical Applications
10.5.1 Hip Reconstructive Surgery
The unique material properties of porous tantalum make it an ideal biomaterial for complex primary and revision total hip replacement (THR) especially in the presence of substantial bone loss and poor bone quality (Fig. 10.1). Porous tantalum has shown excellent fibrous tissue ingrowth throughout the porous structure in areas devoid of bone ingrowth in preclinical studies. This characteristic offers the potential to act as a mechanical barrier to access of fluid and particulate wear debris and implant by-products to the bone-implant interface to mitigate osteolysis. Currently, tantalum acetabular components are available in three versions for hip reconstructive surgeries – a monoblock acetabular component with direct compression molded polyethylene, a monoblock acetabular cup with peripheral screw fixation, and a modular acetabular component coated with porous tantalum framework. Several clinical studies have demonstrated good early clinical and radiographic with monoblock tantalum devices used for acetabular fixation (Table 10.3) [28–37]. Gruen et al. reported the outcome of tantalum monoblock components in 414 primary total hip replacements at an average follow-up of 3 years (2–5 years) [28]. There was no evidence of radiographic osteolysis or component loosening, and the average Harris hip score [HHS] was 90. Moen et al. reviewed 51 THRs using tantalum monoblock components [34]. The authors reported no evidence of osteolysis or loosening on computed tomography (CT) scan at an average follow-up of 10.3 years. In another recent study, Noiseux et al. reported clinical outcomes of tantalum acetabular components in a large group of patients (613) who underwent primary THR [36]. They noted excellent bone ingrowth with no failures related to component loosening at 2–10-year follow-up. However, the monoblock acetabular component has some theoretical disadvantages. The rough external surface of the implant may adhere to surrounding tissue and prevent complete seating of the component resulting in central radiolucency. Additionally monoblock components do not provide the option of isolated exchange of the bearing surface should polyethylene wear and osteolysis occur.
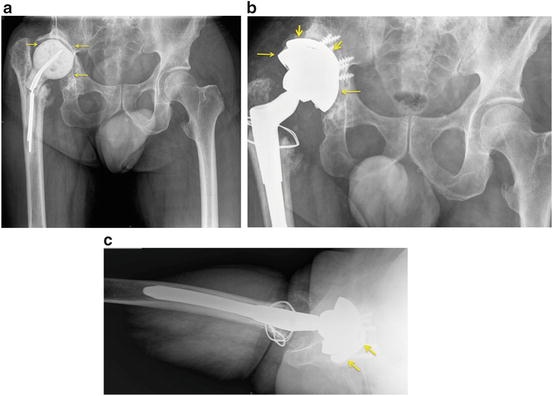
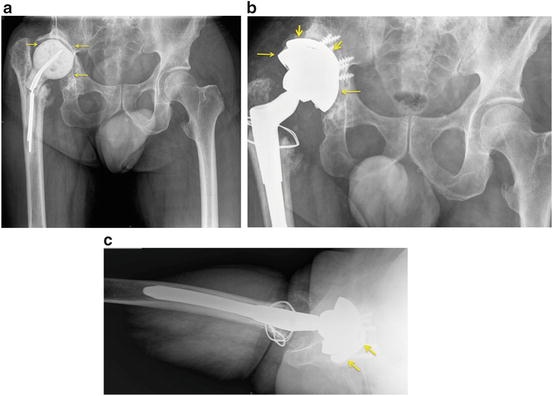
Fig. 10.1
Revision total hip replacement with severe acetabular bone loss. (a) anteroposterior radiograph of the pelvis showing an antibiotic-laden cement spacer and metal rods in the right hip and severe acetabular bone loss (arrows) compared to the contralateral left side. The original right hip replacement was excised due to infection, and a cement spacer was placed temporarily. (b) anteroposterior radiograph after revision total hip replacement of the case seen in (a). The tantalum acetabular shell (thin arrows) and screws are surmounted by a tantalum augment and screws (short thick arrows) to make up some of the lost acetabular bone and place the new cup in a more anatomical socket. (c) cross-table lateral view showing the hemispherical tantalum cup and surrounding tantalum augment (arrows)
Table 10.3
Clinical results of porous tantalum in primary total hip replacement
Author | Number of hips | Average F/U | Clinical outcome [hip scores] | Complications | Reoperations |
---|---|---|---|---|---|
Gruen et al. | 414 | 33 months | N/A | Six femoral and two acetabular intraoperative fractures, one traumatic cup loosening, six recurrent dislocations, and three infections | 10 |
Komaraswamy et al. | 112 | 32 months | Oxford hip score [average – 45] | Three periprosthetic femoral fractures, two DVT, and two trochanteric bursitis | 3 |
Bargiotas et al. | 102 [90 primary and 12 secondary] THRs | 50 months | Harris hip score [94] | No cases of revision, osteolysis, or loosening | 1 |
Macheris et al. | 86 | 7.3 years | N/A | No cases of osteolysis or migration | Nil |
Mullier et al. | 40 | 46 months | 65 % excellent Harris hip score [\90] | One dislocation, one radiographic cup migration without clinical failure | 1 |
Lewis et al. | 245 | 2–5 years | Harris hip score [improved from an average of 49–84] | Nine dislocations, two sepses, two acetabular fractures | Not documented |
Noiseux et al. | 613 | 2–10 years | N/A | Acetabular cup removal 6 [ 1.2 %], femoral and other revisions 19 | 25 |
Macheras et al. | 27 | 10.2 years | Harris hip score 89.5 | N/A | None |
Porous tantalum components appear an attractive option in hip replacement procedures following radiation therapy. The pelvic bone often is comprised of islands of avascular bone interspersed within areas of viable vascularized bone postirradiation. The potentially superior biological and mechanical fixation due to rapid tissue ingrowth from viable host bone may lend itself to better clinical outcome compared to conventional porous coated acetabular components in these complex clinical situations. Rose et al. reported encouraging early clinical outcome in 12 THRs performed after radiation therapy for malignancy using porous tantalum acetabular components [38]. There were no clinical failures or radiographic loosening at an average follow-up of 31 months with average postoperative HHS of 88 points.
Acetabular revision procedures present challenging clinical scenarios with variable amount of bone loss ranging from small contained defects to extensive segmental defects or pelvic discontinuity. The clinical outcomes with reconstruction rings, cages, and bulk allografts used for reconstruction of large acetabular bone defects have been inconsistent and sometimes suboptimal [39–41]. Tantalum implants offer a wide array of options ranging from non-modular to modular acetabular components and augments of various sizes and shapes. The non-modular tantalum components offer the flexibility of placing the screws through the metal itself rather than through preexisting holes to obtain purchase in the best available bone stock. The tantalum augments have almost eliminated the use of bulk allograft which was associated with nonunion and graft collapse leading to compromised acetabular fixation. Historically, reconstruction cages and rings were used for management of extensive segmental acetabular defects and pelvic discontinuity with less than 50 % host bone contact [42]. These constructs do not provide biological fixation and are at risk for late loosening. Owing to enhanced biological and mechanical fixation, tantalum implants offer a good alternative reconstruction option in the presence of such a compromised biological and mechanical environment (Figs. 10.1 and 10.2) [43]. Several clinical studies have demonstrated consistently good clinical outcomes with tantalum implants in revision acetabular surgeries (Table 10.4) [44–52]. Recently, Del Gaizo et al. retrospectively reviewed 37 acetabular revisions at a mean follow-up of 60 months [47]. All patients had Paprosky type IIIa acetabular defects which were treated with porous tantalum acetabular components and augments. One patient developed aseptic loosening of the acetabular component requiring revision surgery. The majority of the patients with these reconstructions had good pain relief and reasonable function with low rates of loosening at midterm follow-up. Similar encouraging results were reported by Lachieweiz et al. in 39 revision acetabular reconstructions using tantalum implants at mean follow-up of 3.3 years [52]. They had 26 hips with Paprosky type III acetabular defects, 11 with type II, and two hips with type I defects. Only one patient developed mechanical failure at 6 months. Radiographic evaluation revealed that bone ingrowth and stable implants were present in all the other hips. They concluded that tantalum acetabular components provide stable fixation in difficult acetabular revision surgeries.
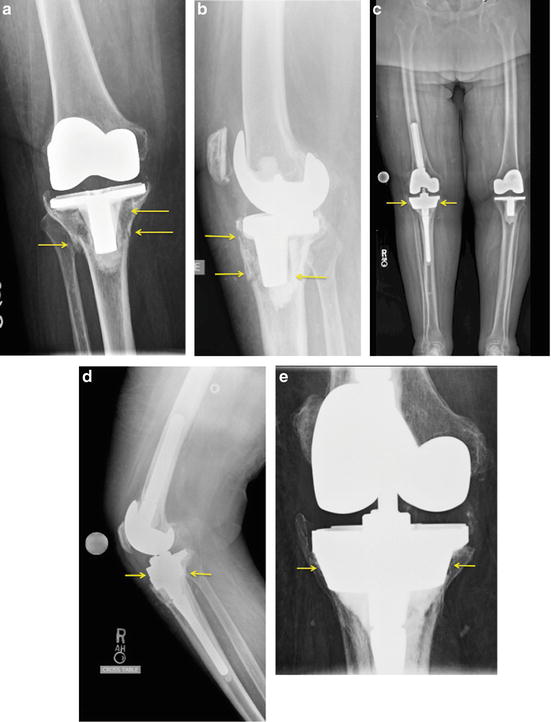
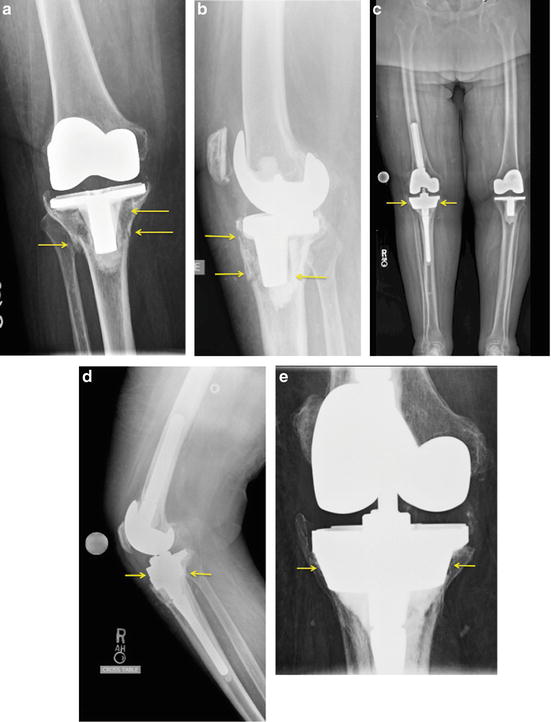
Fig. 10.2
Revision total knee replacement with severe tibial bone loss. Preoperative anteroposterior (a) and lateral (b) radiographs of the knee after cemented total knee replacement. There is loosening and marked bone loss around the tibial component with a fracture of the bone (arrows). Three-foot standing anteroposterior view (c), lateral view (d), and notch view showing the revision total knee replacement. The contralateral left knee is replaced with a conventional primary knee replacement. The right knee has been revised with stemmed more constrained components. The deficient tibial bone near the joint has a tantalum tibial cone (arrows) that replaces the lost metaphyseal cancellous bone in the area of the previous fracture
Table 10.4
Clinical results of porous tantalum in revision hip arthroplasty
Author | Number of revision hips | Follow-up | Complications | Clinical outcome |
---|---|---|---|---|
Unger et al. | 60 | 42 months [14–68] | One revision for aseptic loosening, seven dislocations | Harris hip score 94.4 |
Mardones et al. | 114 | 24 months | Four dislocations, six reoperations | 97.3 % with complete incorporation with host bone |
Paprosky et al. | 12 [pelvic discontinuity] | 2.1 years [1–3] years | One case of possible acetabular loosening secondary to screw breakage | Eleven patients had no or mild pain; one patient had moderate pain |
Nehme et al. | 16 | 31.9 months [24–39] | One revision for pelvic discontinuity, one dislocation, and one sciatic nerve palsy | Harris hip score [56–92] postoperatively |
Boscainos et al. | 14 [cup cage construct] | 32 months [6–45] | Two dislocations, one thigh seroma | Oxford hip score 45 [33–53] |
Del Gaizo et al. | 37 [augments] | 60 months [26–106] | One aseptic loosening, two fractures, three acute infections, and two recurrent dislocations | Harris hip scores 81.5 postoperatively |
Davies et al. | 46 | 50 months | One infection, two dislocations, and one arterial bleeding | Harris hip score 78.2 |
Lachiewicz et al. | 37 | 39 months | One component loosening, three recurrent dislocations, two infections, and 1 fracture | Harris hip score 86 |
Sporer et al. | 28 | 4.4 years | One aseptic loosening, one infection, one vascular injury, and one bowel injury | Merle d’Aubigné-Postel score was 6.6 |
Pelvic discontinuity is characterized by separation of the superior and inferior hemi-pelvis due to either loss of bone or a fracture through the acetabular columns. Paprosky et al. described a surgical method to manage pelvic discontinuity with tantalum implants [46]. They recommended the use of a tantalum augment to span the discontinuity and particulate bone graft to fill any residual cavities before placement of the acetabular component. They reported satisfactory clinical and radiographic outcome with this technique in 20 patients at a mean follow-up of 4.5 years [53]. One patient required revision for aseptic loosening of acetabular component. Implantation of tantalum acetabular components in revision surgery requires great care because of compromised bone stock. Springer et al. reported seven acetabular fractures following implantation of tantalum components during revision acetabular surgeries [54]. They attributed the fractures to potential weakening of the acetabular walls during reaming for revision procedures compounded further by physiological stresses of daily activities. They recommended conservative acetabular reaming and protected weight-bearing protocol to avoid this complication.
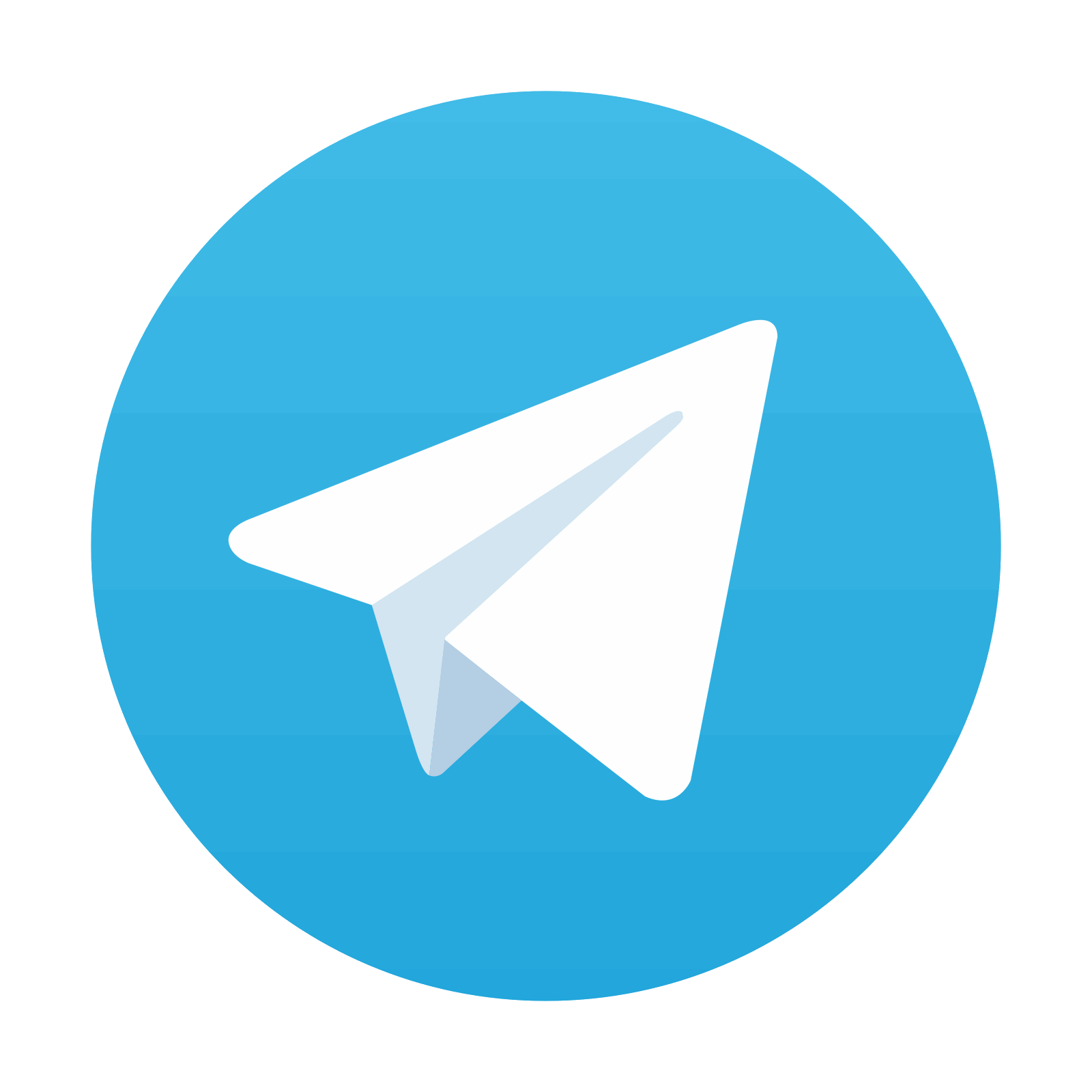
Stay updated, free articles. Join our Telegram channel

Full access? Get Clinical Tree
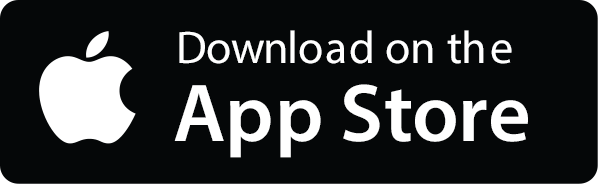
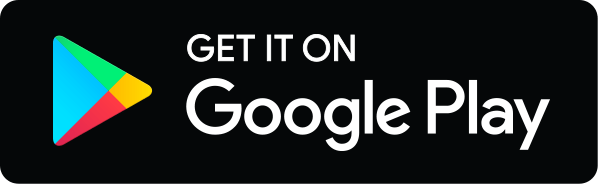