The Placenta
Raymond W. Redline, M.D.
INTRODUCTION
Perinatal pathology, the subdiscipline of pediatric pathology devoted to the study of abnormal pregnancy outcomes, is a dynamic specialty interfacing with obstetrician-gynecologists, neonatologists, and clinical geneticists. A central tenet of this field is that analysis of adverse pregnancy outcome begins with study of the placenta and its adnexa. The fetus is entirely dependent on the placenta for sustenance and protection throughout gestation. Indeed, the placenta has been called a “diary of intrauterine life.” Artificial barriers are often placed between the study of so-called products of conception resulting from early pregnancy loss and placentas submitted to pathology following complications of later pregnancy. Such a separation has no anatomic or functional basis and has probably hindered a complete and holistic understanding of the underlying biologic factors responsible for adverse outcomes in couples with sporadic or recurrent pregnancy loss. The first part of this chapter briefly summarizes key stages of placental development as a basis for understanding the problems of the first and early second trimester. The second part will outline the structure of the mature placenta to provide an anatomic framework for disease processes occurring in the late second and third trimester of pregnancy.
EARLY PREGNANCY
Development
The fertilized zygote undergoes a series of cleavage divisions to form a solid 16 cell morula by 5 days following ovulation (1). Between 5 and 8 days, a number of important events occur; the loose aggregate of cells become compacted, cells at the periphery develop tight junctions and begin transporting fluid into the center of the morula (blastocyst formation), and the surrounding zona pellucida is shed as the blastocyst attaches to, crosses, and invades the endometrium (Figure 9-1). The formation of tight cell-cell junctions at the periphery of the blastocyst marks the emergence of the trophectoderm lineage (trophoblast), which is the principal component of the placenta. Transport of fluid into the blastocyst and invasion of the gestational endometrium (decidua) foreshadow the two most important functions of trophoblast throughout gestation: transport of maternal substrates to the fetus and tissue remodeling of the uterus to ensure adequate delivery of these substrates. Cells within the blastocyst (inner cell mass) separate into two lineages: epiblast, which gives rise to the epithelium surrounding the amnionic cavity (day 8) and the embryonic germ layers (days 15 to 28), and hypoblast, which forms the connective tissue of the placenta (extraembryonic mesoderm) and the primary yolk sac.
Development of the maternal and fetal placental circulations occurs in parallel. The maternal circulation begins when uterine capillaries are eroded by the outer layer of primitive syncytial trophoblasts at the periphery of the blastocyst allowing blood to flow into lacunar spaces that form within the trophoblast. These lacunae gradually enlarge to form the intervillous space. Trophoblasts also migrate against flow into the arterial circulation forming cellular plugs that limit perfusion of the intervillous space (Figure 9-2A) (2). During this period, the walls of the spiral arteries are remodeled in a series of events that includes dissolution of the muscular media, dilatation of the lumen, and reconstitution of the vessel wall by extracellular matrix; all mediated by trophoblasts within the vessel lumen. By the time the trophoblastic plugs disappear at approximately 10 weeks of gestation, the structural integrity of the developing placenta is sufficient to withstand the high
oxygen tension and pressures of arterial blood flow. Extension of the remodeling process to the deeper arteries in the inner third of the myometrium occurs between 10 and 18 weeks of pregnancy. During this period and beyond, the placenta also expands laterally by attachment to and cooptation of large veins at its margins (so-called marginal sinus formation) (3). By the end of pregnancy, approximately 80 and 100 spiral arteries open into the intervillous space.
oxygen tension and pressures of arterial blood flow. Extension of the remodeling process to the deeper arteries in the inner third of the myometrium occurs between 10 and 18 weeks of pregnancy. During this period and beyond, the placenta also expands laterally by attachment to and cooptation of large veins at its margins (so-called marginal sinus formation) (3). By the end of pregnancy, approximately 80 and 100 spiral arteries open into the intervillous space.
The fetal circulation of the placenta develops in two phases (4). Extraembryonic mesoderm migrates out into the trophectoderm between the lacunar spaces to form so-called “primary villi.” A villous capillary plexus then develops within the extraembryonic mesoderm via local inductive interactions with trophoblasts (Figure 9-2B). This plexus eventually becomes connected to the embryonic circulation via anastomoses with large vessels growing out from the fetus into the extraembryonic connective tissue covering the trophoblastic portion of the placenta (chorionic plate). These fetal vessels reach the placenta via the body stalk, later to become the umbilical cord (UC). Paired arteries within the body stalk develop alongside the allantoic duct, and a vein develops alongside the omphalomesenteric duct. Vascularized extraembryonic mesoderm in the primary villi subsequently expands to form villous trees, largely driven by branching angiogenesis. As these trees increase in complexity and the placenta enlarges, the more proximal fetoplacental vessels develop a muscular media and form stem villous arteries and veins.
The final stage of early placental development is formation of the membranes. The first event occurring at about 9 to 11 weeks of gestation is disappearance of the extraembryonic coelom and primitive yolk sac resulting in attachment of the amnionic sac surrounding the fetus to the chorionic connective tissue covering the trophoblastic portion of the placenta. This is followed at 11 to 17 weeks by the gradual atrophy and collapse of the intervillous space in portions of the placenta away from the implantation site. It has been suggested that higher oxygen tension due to the lack of endovascular trophoblast plugs in arteries away from the implantation site triggers this pattern of peripheral atrophy (5). Finally, at about 17 to 20 weeks, the enlarging gestational sac makes contact with and fuses with the opposite side of the uterus forming the mature multilayered placental membrane composed of vascularized decidua vera, avascular decidua capsularis, chorionic trophoblasts (chorion laeve) and connective tissue, and amnionic connective tissue and epithelium.
Multiple Pregnancies
Background
Twins (and higher-order multiple pregnancies) develop following either fertilization of multiple eggs (dizygotic twins) or fission of a single fertilized egg (monozygotic twins). The incidence of dizygotic twinning is variable in different
populations and depends on the frequency of polyovulation, either naturally occurring due to higher endogenous FSH levels or induced by drugs used in association with assisted reproductive technology. Monozygotic twinning occurs at a constant rate in most populations and can be associated with a variety of different placental types (Figure 9-3). The classic explanation for the differences in placental morphology in monozygotic twin placentas is temporal: separation prior to blastocyst formation leads to separate placentas (dichorionic diamnionic), separation between blastocyst formation and amniogenesis to a single placenta with separate amnionic sacs (monochorionic diamnionic), and separation after amniogenesis to a single placenta with one amnionic sac (monochorionic monoamnionic). A new theory posits that monozygotic twinning is always an early postfertilization event and that placental monochorionicity and monoamnionicity depend on proximity and fusion of membrane layers rather than embryo splitting (6). This concept is supported by a recent report that on rare occasions dizygotic twins can fuse to form a monochorionic placenta (7). Secondary fusion due to proximity has also been proposed as an explanation for conjoined twins (8).
populations and depends on the frequency of polyovulation, either naturally occurring due to higher endogenous FSH levels or induced by drugs used in association with assisted reproductive technology. Monozygotic twinning occurs at a constant rate in most populations and can be associated with a variety of different placental types (Figure 9-3). The classic explanation for the differences in placental morphology in monozygotic twin placentas is temporal: separation prior to blastocyst formation leads to separate placentas (dichorionic diamnionic), separation between blastocyst formation and amniogenesis to a single placenta with separate amnionic sacs (monochorionic diamnionic), and separation after amniogenesis to a single placenta with one amnionic sac (monochorionic monoamnionic). A new theory posits that monozygotic twinning is always an early postfertilization event and that placental monochorionicity and monoamnionicity depend on proximity and fusion of membrane layers rather than embryo splitting (6). This concept is supported by a recent report that on rare occasions dizygotic twins can fuse to form a monochorionic placenta (7). Secondary fusion due to proximity has also been proposed as an explanation for conjoined twins (8).
Essentially, all monochorionic twin placentas have vascular connections. Most are surface anastomoses involving chorionic arteries or veins (artery to artery and vein to vein) that lead to sharing of blood (chimerism) but do not cause circulatory imbalance (9). One unusual exception is when large arteries with closely apposed UC insertion sites are connected. In this situation, an artery from one twin may develop sufficient pressure to reverse the circulatory flow in the second twin (twin reversed arterial perfusion {TRAP} sequence) leading to secondary atrophy of the heart and other rostral structures and resulting in the development of an acardiac fetus (10). A second more common exception occurs during the period when the villous circulation connects with the embryonic circulation. Crossed intertwin connections at that time can lead to shared zones that are perfused by the arterial circulation of one twin and drained by the venous system of the other (deep arteriovenous anastomoses).
Pathology
The main task for the pathologist in multiple pregnancies is to determine the number of chorions and amnions in each placenta, usually by direct inspection followed by confirmatory histologic sections from the dividing membrane. This examination is not required when the placental discs are completely separate. If a single placenta is noted and the dividing membrane is opaque, then two amnions flanking a fused central chorion is most likely (dichorionic diamnionic placentas). This is confirmed at gross examination by peeling the three layers, and the placentas should be separated for weighing and further examination. If the dividing membrane is translucent, only two amnions are most likely (monochorionic diamnionic placenta). The chorionic layer is absent because it surrounds, but does not divide, the two fetal sacs. This anatomy can again be confirmed by peeling only two layers. A monochorionic placenta is, as the name suggests, a single organ that should be weighed without further separation. Inspection of the chorionic plate for surface anastomoses should then be performed followed by injection studies to look for deep arteriovenous anastomoses. Air injection studies are a quick and easy method for demonstrating clinically significant connections (1). More complete injection studies using colored or radio-opaque dyes are usually only conducted in a research context. Discordant twin growth, usually defined as a greater than 25% difference in body weights, is a significant complication of both dichorionic and monochorionic gestations. Pathologic lesions other than transfusion syndrome (see below) that are significantly associated with discordant growth include peripheral cord insertion, avascular villi, and indicators of maternal vascular malperfusion (11,12).
Clinical Correlation
Twin gestations of all types are at increased risk for premature delivery, intrauterine growth restriction (IUGR), preeclampsia, and cerebral palsy. Many of these complications are more frequent in the smaller and/or the nonpresenting (second) twin. Twin-to-twin transfusion syndrome (TTTS),
affecting monochorionic twins, is a specific form of discordant twin growth caused by large deep arteriovenous anastomoses without accompanying superficial arterial anastomoses (9). TTTS is characterized by growth restriction and oligohydramnios in the donor twin and macrosomia, congestive heart failure, and polyhydramnios in the recipient. Twin anemia polycythemia sequence (TAPS) is another clinical syndrome characterized by differences in hemoglobin concentrations in twins developing secondary to chronic net transfusion across smaller arteriovenous anastomoses. Finally, acute antenatal or intrapartum transfusion occurs when previously balanced anastomoses become unbalanced due to changes in fetal blood pressures or secondary occlusion of bridging fetal vessels. The most dramatic example of acute transfusion occurs after fetal demise of one twin. In this case, sudden shifts of blood from the survivor to the decedent result in a very high risk of perinatal brain damage.
affecting monochorionic twins, is a specific form of discordant twin growth caused by large deep arteriovenous anastomoses without accompanying superficial arterial anastomoses (9). TTTS is characterized by growth restriction and oligohydramnios in the donor twin and macrosomia, congestive heart failure, and polyhydramnios in the recipient. Twin anemia polycythemia sequence (TAPS) is another clinical syndrome characterized by differences in hemoglobin concentrations in twins developing secondary to chronic net transfusion across smaller arteriovenous anastomoses. Finally, acute antenatal or intrapartum transfusion occurs when previously balanced anastomoses become unbalanced due to changes in fetal blood pressures or secondary occlusion of bridging fetal vessels. The most dramatic example of acute transfusion occurs after fetal demise of one twin. In this case, sudden shifts of blood from the survivor to the decedent result in a very high risk of perinatal brain damage.
Gestational Trophoblastic Disease
Background
Trophoblast may be separated into two major subtypes, villous and extravillous (13). Neoplasia involving villous trophoblast leads to choriocarcinoma while that of extravillous trophoblasts results in either placental site trophoblastic tumor (PSTT) or epithelioid trophoblastic tumor (ETT) (14). More than half of choriocarcinomas are preceded by molar pregnancies (complete or partial hydatidiform moles). All molar pregnancies have an overrepresentation of paternal chromosomes. Complete moles form after fertilization of an empty ovum and contain only paternal chromosomes while partial moles usually follow double fertilization of a single egg and thus have a 2:1 ratio of paternal to maternal chromosomes. Studies in mice have shown that trophoblast proliferation is largely driven by growth-promoting factors that are transcribed exclusively from paternally inherited genes while antiproliferative genes are often expressed only from maternal alleles (15). An increased frequency of molar pregnancies explains the increased incidence of choriocarcinoma in Asian populations. Although occasionally developing after molar pregnancy, PSTT and ETT most commonly follow term pregnancies, often years after delivery. It is well documented that some extravillous trophoblast is left behind after delivery and can persist for many years. Although not proven, these persistent rests of trophoblasts, known as placental site nodules, might be precursors for these rare tumors.
Pathology
Molar pregnancies are characterized by villous edema and trophoblastic hyperplasia (16). Edema is often extreme leading to cavitation of the villous stroma. In complete moles, the edema and hyperplasia affect the entire conceptus while in partial moles, they affect only a subgroup of villi (Figure 9-4A). Partial moles also show irregularly shaped
dysmorphic villi (Figure 9-4B). The classic complete mole is easily diagnosed based on clusters of fluid-filled vesicles (hydatidiform or “grape-like” change) and marked trophoblastic hyperplasia. However, with the current use of early ultrasound approximately one-third of complete moles are evacuated at a stage before the development of edema or diffuse trophoblastic hyperplasia. These early complete moles can be difficult to recognize but manifest a number of helpful diagnostic features including a cauliflower-like growth pattern, densely cellular myxoid villous stroma, focal trophoblastic hyperplasia, and atypia of implantation site trophoblasts (Figure 9-4C). Occasionally, early pregnancy specimens show nonspecific trophoblastic hyperplasia in the absence of molar villi. Cytogenetic study of these specimens has shown a high prevalence of the two relatively uncommon karyotypes, trisomies 7 and 15 (17). Whether these cases have an increased risk of later choriocarcinoma is not known.
dysmorphic villi (Figure 9-4B). The classic complete mole is easily diagnosed based on clusters of fluid-filled vesicles (hydatidiform or “grape-like” change) and marked trophoblastic hyperplasia. However, with the current use of early ultrasound approximately one-third of complete moles are evacuated at a stage before the development of edema or diffuse trophoblastic hyperplasia. These early complete moles can be difficult to recognize but manifest a number of helpful diagnostic features including a cauliflower-like growth pattern, densely cellular myxoid villous stroma, focal trophoblastic hyperplasia, and atypia of implantation site trophoblasts (Figure 9-4C). Occasionally, early pregnancy specimens show nonspecific trophoblastic hyperplasia in the absence of molar villi. Cytogenetic study of these specimens has shown a high prevalence of the two relatively uncommon karyotypes, trisomies 7 and 15 (17). Whether these cases have an increased risk of later choriocarcinoma is not known.
Choriocarcinomas often present with large areas of uterine hemorrhage and necrosis. The cellular areas show a typical pattern characterized by clusters of 10 to 50 mononuclear cytotrophoblasts surrounded by a wreath-like garland of syncytial trophoblasts (Figure 9-5A). The mononuclear cytotrophoblastic cells show mild-to-moderate nuclear atypia with prominent nucleoli and a watery clear cytoplasm. The multinucleate syncytial trophoblastic cells have enlarged hyperchromatic nuclei and a deeply eosinophilic-purple cytoplasm. Syncytial trophoblasts stains intensely for human chorionic gonadotropin (hCG), human placental lactogen (hPL), and inhibin, while the cytotrophoblasts lacks these hormones but expresses p63. Both cell types are cytokeratin positive (13,14).
PSTT is derived from mature extravillous trophoblasts and are characterized by large mononuclear cells with significant nuclear atypia and abundant intensely eosinophilic cytoplasm (Figure 9-5B). Binucleation is occasionally seen, but larger numbers of nuclei, as seen in syncytial trophoblasts, are rare. Unlike normal intermediate trophoblasts, tumor cells invade in large cohesive sheets and are associated with tissue necrosis. Tumor cells stain positively for cytokeratin, inhibin, and hPL, but are only weakly or focally positive for hCG and p63 (13,14).
ETT are derived from so-called transitional extravillous trophoblasts and are composed of nests of vacuolated or eosinophilic cells often in a hyaline matrix, bearing a striking resemblance by both light microscopy and immunostaining to the cells of the membranous chorion laeve (Figure 9-5C). They tend to grow in a nodular expansile pattern in the lower uterine segment or cervix where they can mimic squamous carcinomas. Their immunohistochemical profile includes diffuse expression of cytokeratin and p63 plus focal/variable expression of inhibin, hCG, and hPL (13,14).
Clinical Correlation
All preneoplastic and neoplastic lesions of trophoblasts are combined under the rubric gestational trophoblastic disease. While molar pregnancies are usually confirmed by tissue diagnosis, postmolar choriocarcinomas are often managed based solely on serum monitoring of the tumor marker, hCG, and radiographic imaging. Persistence or elevation of hCG levels after evacuation of a molar pregnancy is treated empirically with single agent chemotherapy. Tumors with extremely high hCG levels or metastasis to organs other than the lung are treated with multiple agent chemotherapy. Bulky uterine disease may necessitate hysterectomy. PSTT and ETT usually present with vaginal bleeding. Curettage is suspicious for a neoplasm, and hCG levels are usually positive, but often at low levels. Radiographic studies confirm a mass lesion, and hysterectomy is performed. Unlike choriocarcinoma, PSTT and ETT are relatively indolent and only rarely metastasize. However, they respond poorly to chemotherapy, so local control is paramount. Clinical management of early pregnancy specimens suspicious for, but not diagnostic of, gestational trophoblastic disease (avillous trophoblasts or villi with nonspecific trophoblastic hyperplasia) should include at least one follow-up hCG titer to ensure return to baseline.
Missed Abortion/Anembryonic Pregnancy
Background
The term missed abortion refers to a pregnancy in which a nonviable chorionic sac is retained in the uterus requiring curettage for evacuation. Early gestational sacs lacking sonographic and/or histologic evidence of embryonic development (anembryonic pregnancy) are most common. A large percentage of such specimens have embryonic lethal chromosomal abnormalities (18). Chromosomally normal anembryonic pregnancies probably represent major disruptions of early embryogenesis due to teratogens, sporadic mutations in major developmental genes, or random errors in morphogenesis (see chapter 3).
Pathology
Anembryonic pregnancies show a typical histopathologic profile. Typical features include a relatively thin chorionic membrane, and uniformly edematous (hydropic) villi (Figure 9-6A). Amnion, yolk sac, UC, and embryonic tissue are usually absent, and no fetal blood vessels are apparent. The uniformly hydropic nature of the villi is caused by continuing trophoblast transport of fluid into villi without egress to a fetal circulation. With prolonged retention, the hydropic villi may undergo secondary fibrosis (hyalinization). Gestational endometrium and implantation site in these cases usually lack hemorrhagic/ischemic degeneration or other abnormalities.
Clinical Correlation
The management of women with early pregnancy losses, particularly when recurrent, is highly dependent on the nature of the loss. A careful pathologic examination can often guide clinical management in cases where cytogenetic analysis either has not been obtained or is unsuccessful (18). Recognition of anembryonic gestation (sometimes referred to as “blighted ovum” or hydropic abortus) by early ultrasound or pathologic examination is clinically useful in that it identifies a cohort with a high rate of aneuploidy and hence a low recurrence rate. Early and late missed abortions with normally vascularized villi, thromboinflammatory lesions, or endometrial pathology are much more likely to recur in subsequent pregnancies.
Miscarriages (Threatened, Incomplete, and Complete Abortions)
Background
The term miscarriage refers to an early pregnancy in varying stages of being spontaneously expelled from the mother (threatened, incomplete, and complete abortions). Many occur following previous fetal demise. These cases represent a heterogenous mixture of chromosomally normal and abnormal gestations, some with fetal malformations, deformations, or disruptions. An uncommon, but clinically important, subgroup is miscarriage with evidence of an underlying thromboinflammatory process.
These processes are more frequent in patients with recurrent miscarriages and may represent aberrant maternal responses to pregnancy (18). One specific maternal condition, antiphospholipid antibody syndrome, has been associated with vascular thrombosis, hemorrhage, and complement-mediated damage to maternal endothelial cells and/or trophoblasts.
Pathology
The most common phenotype in spontaneous miscarriage is a well-developed chorionic sac with an adherent amnion, hyalinized villi with partially involuted fetal vasculature, and a combination of hemorrhage and necrosis in the implantation site and gestational endometrium (Figure 9-6B). Another common subtype contains a normally formed, viable or recently deceased embryo or fetus plus a large amount of fresh intervillous hemorrhage (so-called Breus mole). In addition to intervillous hemorrhage, these specimens show normally vascularized chorionic villi with early stromal-vascular karyorrhexis and, in some cases, thrombosis or congestion of spiral arteries with an absence of endovascular trophoblastic plugs (Figure 9-6C, D). Specimens with evidence for a thromboinflammatory process are much less common. Three variants have been described: (a) maternal floor infarction/massive perivillous fibrin deposition (discussed later), (b) chronic histiocytic intervillositis, characterized by extensive infiltration of the intervillous space by a
monomorphic infiltrate of monocyte-macrophages (Figure 9-6E), and (c) decidual arteriopathy, often accompanying antiphospholipid syndrome or other autoimmune diseases, and characterized by vasculitis, mural hypertrophy, perivascular decidual fibrin deposition, and/or plasma cell infiltration (Figure 9-6F) (18,19).
monomorphic infiltrate of monocyte-macrophages (Figure 9-6E), and (c) decidual arteriopathy, often accompanying antiphospholipid syndrome or other autoimmune diseases, and characterized by vasculitis, mural hypertrophy, perivascular decidual fibrin deposition, and/or plasma cell infiltration (Figure 9-6F) (18,19).
Clinical Correlation
Patients with recurrent, chromosomally normal miscarriages, particularly in association with thromboinflammatory lesions and/or the antiphospholipid antibody syndrome, are often treated with low-dose heparin and/or aspirin. Less commonly, more aggressive anticoagulation, intravenous gamma globulin, or corticosteroids have been utilized. Previous therapeutic options that have largely been abandoned include other types of immunosuppressive drugs, diethyl stilbestrol, progesterone, and immunization with paternal leukocytes.
Congenital Infections
Background
Although ascending infections involving organisms from the cervicovaginal tract do occur in the first half of pregnancy (discussed later), the majority of early infections are acquired hematogenously (1,20). Most occur after primary maternal infections, since previous exposure generally elicits protective antibodies. Organisms infecting both placenta and fetus include spirochetes (Treponema pallidum), parasites (Toxoplasma gondii, Trypanosoma cruzi), and viruses (cytomegalovirus, varicella zoster virus, herpes simplex virus, rubella virus, poxviruses). Those that usually infect only the placenta include Plasmodium falciparum, Borrelia burgdorferi, and Schistosoma hematobium. Other organisms cross the placenta to infect the fetus without eliciting placental inflammation including Parvovirus B19, HIV, hepatitis B, and the enteroviruses. The majority of congenital infections begin in the second trimester. First-trimester infections are less common, but more severe, and those occurring in the earliest stages of pregnancy often spare the products of conception entirely.
Pathology
Organisms restricted to the placenta usually involve either the decidua or the intervillous space. Histologic findings include local accumulations of fibrin and a mixed acute and chronic inflammatory infiltrate. Infections involving both fetus and placenta generally cause a diffuse placentitis with chronic inflammation of the chorion, decidua, and villous stroma. Two overlapping patterns are seen. The first, characterized by edematous villi with increased Hofbauer cells, is typical of syphilis (Figure 9-7A). The second, characterized by fibrotic villi with evidence of remote hemorrhage and villous plasma cells, is typical of CMV (Figure 9-7B). Some infections have unique features allowing a specific histopathologic diagnosis. These include the presence of organisms or viral inclusions in the villous stroma (CMV, herpes simplex virus, varicella zoster virus, T. cruzi), UC (T. pallidum, T. gondii), or intervillous space (P. falciparum, S. hematobium).
Clinical Correlation
A common clinical mnemonic for congenital infection is the acronym TORCH, standing for Toxoplasma, Other agents, Rubella virus, Cytomegalovirus, and Herpes simplex virus. In the United States, CMV and syphilis account for more than 90% of cases. Infants with any TORCH infection share common features including IUGR, pancytopenia, hepatosplenomegaly, and coagulopathy. Each infection also has specific features, a description of which is beyond the scope of this chapter. A standard serologic screen known as the “TORCH titer” tests for maternal IgG specific for the common TORCH agents and is part of the routine workup for IUGR or suspected antenatal maternal infection. Specific testing for IgM is required to distinguish recent from remote infection. Many infections are diagnosed by PCR testing of fetal blood or amniotic fluid obtained by amniocentesis.
LATE PREGNANCY
Anatomy
The mature placenta is composed of four distinct compartments of structure-function:
Fetoplacental vasculature: UC, chorionic plate and vascularized villous stroma
Interhemal membrane: villous trophoblasts and adjacent maternal intervillous space
Basal plate: anchoring villi, decidua, and underlying maternal uterine vasculature
Placental membranes: amnion, chorion, and adjacent maternal decidua.
The fetoplacental vasculature begins at the UC, a squamous epithelial-covered conduit usually measuring between 40 and 70 cm in length at term that conducts fetal blood from the umbilicus to some location on the chorionic plate (or occasionally the adjacent placental membranes). The UC contains paired arteries that spiral around a central vein; all surrounded by a hyaluronate-rich matrix (Wharton jelly), which provides considerable protection from external compression. The two arteries are connected at or just before their insertion site into the chorionic plate by an anastomosis (Hyrtl’s anastomosis). The chorionic plate (also known as the fetal surface) is composed of fibroconnective tissue supporting large muscular arteries and veins that distribute fetal blood flow from the UC to a family of 20 and 30 large villous trees (4). These villous trees protrude from the underside of the chorionic plate, branch several times, and conduct fetal blood through a succession of ever smaller arteries and veins, terminating in capillaries that interface with the trophoblastic interhemal membrane. The larger (proximal) villi are separated into stem and intermediate villi with the latter representing the arteriolar level at which blood flow into the gas exchanging terminal (distal) villi is regulated. Maturation of the villous tree with advancing gestational age in the third trimester is characterized by an increase in the number of distal relative to proximal villi, decreased distal villous connective tissue, peripheralization of villous capillaries, and the formation of specialized vasculosyncytial membranes that optimize gas exchange. There is, however, considerable regional variation in maturity such that well-perfused villi overlying the opening of the maternal spiral artery at the center of a cotyledon may appear considerably less mature than those at the “watershed” between spiral arteries at the periphery of the cotyledon (Figure 9-8A, B). The two critical anatomic features to be maintained in this compartment are patency of large proximal villous vessels and a short diffusion distance between fetal capillaries and distal villous trophoblasts.
The interhemal membrane consists of multinucleate syncytiotrophoblastic cells, a few scattered, basally located cytotrophoblastic stem cells, and an underlying basement membrane. Each cytotrophoblastic stem cell is the progenitor for 80 to 100 differentiated syncytiotrophoblastic cells that fuse into large syncytial sheets forming a mosaic covering the entire villous tree (21). Turnover of syncytiotrophoblasts occur via clustering of nuclei into syncytial knots that are later shed into the maternal circulation (22). Critical features at the interhemal membrane are cellular viability; appropriate maturation in terms of endocrine, transport, anticoagulant, and immunoprotective function; and accessibility to maternal blood flow—meaning absence of adherent fibrin or inflammatory exudate in the intervillous space.
The basal plate consists of so-called anchoring villi embedded in maternal endometrium, ectatic maternal spiral arteries, and tangentially oriented maternal veins. Extravillous trophoblasts, differentiating from villous cytotrophoblastic stem cells on the underside of the anchoring villi diffusely, infiltrate the maternal endometrium and elaborate large amounts of a fibronectin-rich extracellular matrix forming the Nitabuch layer, which provides structural integrity and unites the diverse components of the basal plate into a coherent anatomic structure. Basal plate arteries normally lack a smooth muscle layer, having been remodeled by endovascular extravillous trophoblasts. Adequate arterial remodeling is a critical feature of mature placentas allowing adequate blood flow into the intervillous space. Lateral villous trees grow into maternal veins at the periphery of the placenta resulting in the formation of large sinusoidally dilated veins that surround the disc (Figure 9-8C) (3). These structures were previously misinterpreted as a continuous marginal venous sinus.
The placental membranes appear to be a distinct structure, but this appearance is deceptive. Membranes form by secondary involution of early placental tissue not included in the final placental disc, and their basic anatomic architecture is the same. The amnion and chorionic connective tissue overly a trophoblastic region containing scattered involuted chorionic villi. This trophoblast coalesces as the intervillous space is obliterated to form a distinct zone known as the chorion leave, composed largely of transitional extravillous trophoblast) (13). The chorion laeve is supported by the underlying maternal decidua. Critical features in this compartment are maintenance of integrity and contiguity of all layers. In particular, chorionic prostaglandin dehydrogenase in chorion laeve trophoblasts must be functionally active and spatially positioned to inactivate labor-inducing prostaglandins elaborated by the amnion, decidua, and myometrium. Inflammatory or ischemic damage to these cells can result in the premature onset of labor (23).
Chronic Disease Processes
Disease processes affecting the placenta in late pregnancy can be classified in a variety of ways including anatomic location, mechanism of injury, or clinical presentation. One way that is particularly useful for understanding the causal sequence of events leading to adverse pregnancy outcomes is time of onset. The rationale for such a separation is that earlier placental lesions can significantly decrease placental reserve lowering the threshold for fetal injury and resulting in an enhanced effect of comparatively minor stressors at the time of parturition (preconditioning). In the following scheme, the term chronic refers to lesions evolving over weeks, subacute to those evolving over many hours to days, and acute to those evolving over just a few hours.
Maternal Vascular Malperfusion
Background
Chronic maternal vascular malperfusion of the intervillous space can result from a variety of causes including underlying cardiac insufficiency, failure to expand intravascular volume during pregnancy, or abnormalities in uteroplacental arteries. It is currently believed that the major process leading to malperfusion is failure of extravillous trophoblasts to appropriately invade and remodel the uterine spiral arteries. While the exact mechanism remains uncertain, a likely sequence of events appears to be early hypoxia at the implantation site leading to dysregulation of renin-angiotensin, vascular endothelial growth factor, integrin, and tissue protease expression and resulting in impaired trophoblasts differentiation and inadequate tissue invasion (24,25). In the absence of normal arterial remodeling, the placenta is inadequately perfused leading to decreased fetoplacental growth and, in some cases, the release of vasoactive mediators that cause the clinical syndrome known as preeclampsia. Several of these mediators have been identified including soluble VEGF receptor 1 (sflt-1), soluble endoglin, and circulating AT1 receptor antibodies (24,26).
Pathology
Placentas affected by maternal malperfusion generally show two or more of a constellation of pathologic abnormalities (27). Important and sometimes overlooked features include decreased weight for gestational age and decreased placental weight relative to that of the infant (increased fetoplacental weight ratio). These features correlate with impairment of placental growth (distal villous hypoplasia) as the fetus sacrifices placental reserve (estimated at up to 30% of weight) in order to supply critical vascular beds such as the central nervous and cardiovascular systems (Figure 9-9A). Complete obstruction to villous blood flow secondary to spiral artery thrombosis leads to villous infarction (Figure 9-9B). Partial obstruction leads to stasis with intervillous fibrin deposition (Figure 9-9C), hypoxia with accelerated syncytiotrophoblast turnover and increased syncytial knots (Figure 9-9D), and localized ischemia with villous agglutination (Figure 9-9E). This process is now referred to as accelerated villous maturation. A quantitative approach to the diagnosis of increased syncytial knotting has been described (28). Particularly, useful
for diagnosis is an alternating pattern of villous crowding with knots, fibrin, and agglutination with intervening areas of villous paucity (hypoplasia) that can easily be recognized at scanning magnification (Figure 9-9F). Three other site-specific abnormalities may also be present: (a) Persistent muscularization of spiral arteries and aggregation of placental site giant cells in the basal plate correlating with superficial implantation. (b) Decidual arteriopathy, either medial hypertrophy (Figure 9-9G) or fibrinoid necrosis (acute atherosis) (Figure 9-9H), linked to either underlying vascular disease or the pregnancy-specific vasoactive mediators discussed above. It is most frequently detected in small arterioles in the placental membranes near the disc margin, and (c) a thin UC (<8 mm diameter) reflecting fetal extracellular volume depletion and decreased hydration of Wharton jelly.
for diagnosis is an alternating pattern of villous crowding with knots, fibrin, and agglutination with intervening areas of villous paucity (hypoplasia) that can easily be recognized at scanning magnification (Figure 9-9F). Three other site-specific abnormalities may also be present: (a) Persistent muscularization of spiral arteries and aggregation of placental site giant cells in the basal plate correlating with superficial implantation. (b) Decidual arteriopathy, either medial hypertrophy (Figure 9-9G) or fibrinoid necrosis (acute atherosis) (Figure 9-9H), linked to either underlying vascular disease or the pregnancy-specific vasoactive mediators discussed above. It is most frequently detected in small arterioles in the placental membranes near the disc margin, and (c) a thin UC (<8 mm diameter) reflecting fetal extracellular volume depletion and decreased hydration of Wharton jelly.
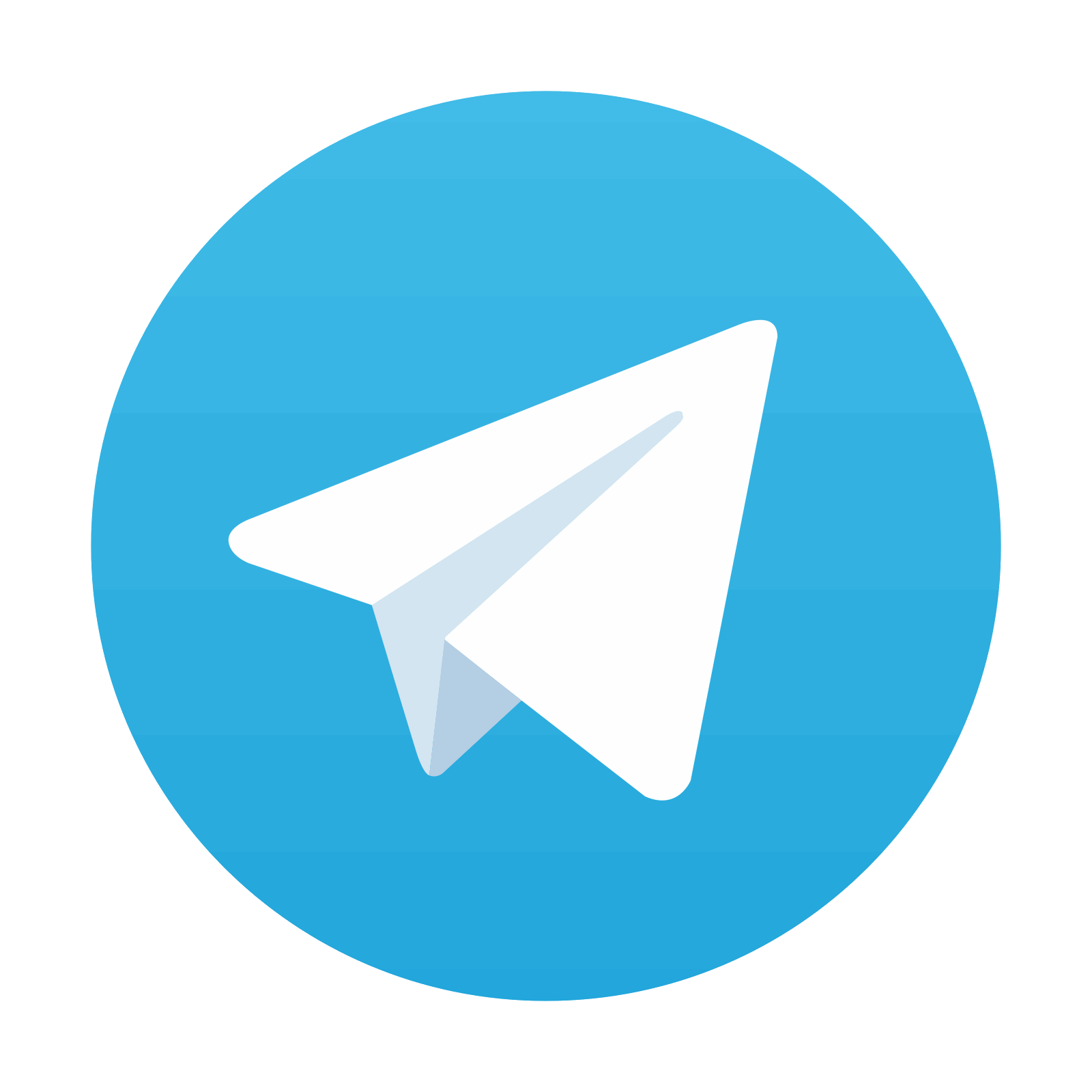
Stay updated, free articles. Join our Telegram channel

Full access? Get Clinical Tree
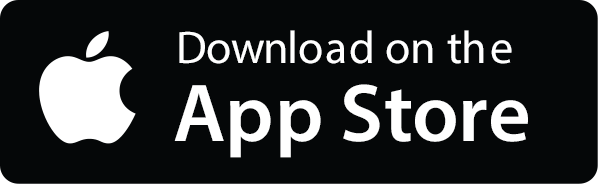
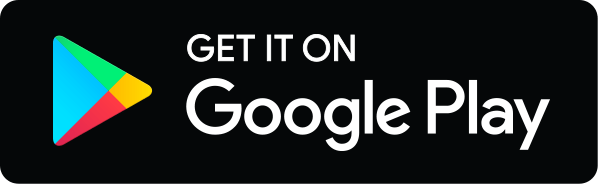
