1 Introduction
Immunological products comprise a group of pharmaceutical preparations of varied composition but with a common pharmacological purpose: the modification of the immune status of the recipient, either to provide immunity to infectious disease, or in the case of in vivo diagnostics, to provoke an indication of immune status usually signifying previous exposure to the sensitizing agent. The immunological products that are currently available are of the following types: vaccines; in vivo diagnostics; immune sera; human immunoglobulins; mono-clonal antibodies; and antibody-targeted therapeutics and diagnostics. For the purpose of this chapter, cell biology-derived immunomodifiers with a non-specific action, e.g. cytokines or chemokines, are not included.
Vaccines are by far the most important immunological products. They have enabled the control or eradication of numerous infectious diseases affecting humans and their domesticated animals. For example, the systematic application of smallpox vaccine, deployed under the aegis of the World Health Organization (WHO), achieved the eradication of one of the most devastating infections. Similarly, the universal application of poliomyelitis vaccine has brought poliomyelitis to the verge of eradication. Diphtheria, tetanus, pertussis (whooping cough), measles and rubella vaccines have been applied worldwide through national or UNICEF-sponsored healthcare programmes and have virtually eliminated these diseases in those countries in which there have been the resources and the will to deploy them effectively. Vaccines that provide protection against many other infections are available for use in appropriate circumstances. Some, such as hepatitis B and conjugate vaccines against Haemophilus influenzae b (Hib), meningococci and pneumococci, have had a huge impact on morbidity and mortality wherever they have been applied.
The range of disorders that may be prevented or treated by vaccines has enlarged considerably beyond infectious diseases. Vaccines are currently undergoing evaluation for several other purposes including therapy of cancer; prevention of allergies; desensitization of allergic patients; fertility control; and treatment of addictions.
In vivo diagnostics such as tuberculins, mallein, histoplasmin, coccidioidin and brucellin, are used to demonstrate an immune response, and hence previous exposure, to specific pathogens as an aid to diagnosis. Allergen skin test diagnostics are used to indicate sensitization to materials of biological origin that may be present in the environment or in specific products. Others, such as the Schick test (diphtheria) toxin are used to detect the presence of protective immunity. Because of their clinical and pharmaceutical limitations, the trend has been to phase out these preparations, and tuberculins (as purified protein derivative, PPD) are now by far the most important of this group.
Immune sera, which were once very widely used in the prophylaxis and treatment of many infections, have more limited use today. Vaccines and antibiotics have super-seded some and lack of proven therapeutic benefit has caused others to be relegated to immunological history. However, some still play an important role in the management of specific conditions. Thus, diphtheria and botulinum antitoxins prepared in horses remain the only specific treatments for diphtheria and botulism respectively. Equine tetanus antitoxin is still used as an effective prophylactic in some parts of the world, although largely replaced by human tetanus immunoglobulin in developed countries. Similarly, antivenins prepared in horses, sheep, goats or other animals against the venoms of snakes, spiders, scorpions and marine invertebrates still provide the only effective treatment for venomous bites and stings and are important therapeutic agents in some parts of the world.
Human immunoglobulins have important but limited uses, for example in the prophylaxis of hepatitis A, hepatitis B, tetanus and varicella zoster. Additional specific immunoglobulins against diphtheria and botulism toxins are under development and vaccinia immunoglobulinmay be reintroduced. Monoclonal antibodies to bacterial endotoxin, to cytokines involved in the pathogenesis of septic shock and to specific infectious agents have been developed and evaluated clinically but have yet to enter into general use. Monoclonal antibodies against specific cell receptors have undergone a rapid development and are employed successfully in cancer therapy and are under development for treatment of autoimmune disease. Immune sera and human immunoglobulins depend for their protective effects on their content of antibodies derived, in the case of immune sera, from immunized animals and, in the case of immunoglobulins, from humans who have been immunized or who have high antibody titres as a consequence of prior infection. The form of immunity conferred is known as passive immunity and is achieved immediately but is limited in its duration to the time that protective levels of antibodies remain in the circulation (see also Chapter 10).
Vaccines achieve their protective effects by stimulating the immune system of the recipient to produce T-cells and/or antibodies that impede the attachment of infectious agents, promote their destruction or neutralize their toxins. This form of protection, known as active immunity, develops in the course of days following infection and in the case of many vaccines develops adequately only after two or three doses of vaccine have been given at intervals of days or weeks. Once established, this immunity can last for years but it may need to be rein-forced by booster doses of vaccine given at relatively long intervals. The immunogenicity of some vaccines can be improved by formulating them with adjuvants. These are a heterogeneous group of substances which enhance the immune response. Aluminium hydroxide gel (hydrated aluminium oxide) and aluminium phosphate are the only ones currently in general use in human vaccines. A much wider range of substances including oily emulsions, saponin, immune-stimulating complexes (ISCOMS), monophosphoryl lipid A, CpG motif contained in oligo-deoxynucleotide (CpG-ODN) and others are used in veterinary vaccines, and some are under investigation for use in human vaccines.
Different types of infectious agent require preferential mobilization of different arms of the immune response. For example, toxigenic bacterial infections require the production of toxin neutralizing antibodies whereas intracellular bacterial infections such as tuberculosis require cell-mediated responses involving mixed T-lymphocytes and activated macrophages, whereas many viral infections will require neutralizing antibody and cytotoxic T-cell responses for effective protection. Achieving the appropriate response can be difficult and in the past has had to be approached empirically. This is why most successful viral vaccines have been based on live attenuated strains, which simulate natural infection. Non-living vaccines have been effective against many bacterial infections but markedly less so against those requiring sustained cell-mediated responses. The development of more selective vaccine adjuvants and delivery systems promises to put the future process of vaccine design on a more rational basis.
A property common to vaccines, immune sera and human immunoglobulins is their high specificity of action. Usually each provides immunity to only one infection, although in some cases cross-protection can occur, e.g. BCG protects against both tuberculosis and leprosy. Where it is necessary to protect against more than one type of agent, monospecific preparations can be combined. For example, botulism antitoxin usually covers types A, B and E; meningococcal polysaccharide vaccine may cover groups A, C, W125 and Y; and pneu mococcal polysaccharide vaccine usually covers 23 sero-types. Heterologous preparations may also be combined as in measles/mumps/rubella and diphtheria/tetanus/pertussis vaccines. With the increasing number of vaccines for infants and young children, the trend is to produce more complex combinations such as diphtheria/tetanus/pertussis/hepatitis B/inactivated polio/Hib vaccine, to minimize the number of injections. The possible additive or interactive effects of the various components on the immune system have raised concerns about the safety of such combinations. While some evidence of reduced responses to certain components has been obtained, there is little to support suggestions of serious adverse effects from current combinations.
In addition to the three main types of immunological products that are widely available, more specialized preparations include: synthetic peptide immune response modifiers such as those used to block T-cell responses in multiple sclerosis; monoclonal antibodies for cancer therapy or diagnosis; and hybrid toxins containing a bacterial or plant toxin subunit attached to an antibody or human cell receptor-binding protein, and also intended mainly for cancer therapy. These have rather limited applications and for the most part, are designed to suppress or exploit the specificity of immune responses rather than to stimulate them.
Principles of immunity are discussed in Chapter 9, and Chapter 10 describes a vaccination and immunization programme.
The vaccines currently used for the prevention of infectious diseases of humans are all derived, directly or indirectly, from pathogenic microorganisms. The basis of vaccine manufacture thus consists of procedures which produce from infectious agents, their components or their products, immunogenic preparations that are devoid of pathogenic properties but which, nonetheless, can still induce a protective response in their recipients. The methods that are used in vaccine manufacture are constrained by technical limitations, cost, problems of delivery to the recipient/patient, by regulatory issues and, most of all, by the biological properties of the pathogens from which vaccines are derived. Those vaccines currently in use in conventional immunization programmes are of several readily distinguishable types.
2.1 Types of vaccines
2.1.1 Live vaccines
These are preparations of live bacteria, viruses or other agents which, when administered by an appropriate route, cause subclinical or mild infections. In the course of such an infection the components of the microorganisms in the vaccine evoke an immune response which provides protection against the more serious natural disease. Live vaccines have a long history, dating from the development of smallpox vaccine. Initially, material from mild cases of smallpox was used for inoculation. This process of ‘variolation’ was hazardous and could produce fatalities and secondary smallpox cases. A much safer alternative was introduced in 1796 by the Gloucestershire physician Edward Jenner, following observations made by Benjamin Jesty, a local farmer, that an attack of the mild condition known as cowpox (probably a rodent pox) protected milkmaids from smallpox during epidemics of this dreaded disease. For many years the cowpox vaccine was propagated by serial transfer from person to person and at some point evolved into a distinctive virus, vaccinia, with some features of both cowpox and small-pox viruses but quite probably derived from a now extinct poxvirus. Vaccinia was eventually used to eradicate small-pox. Its significance was that it could stimulate a high degree of immunity to smallpox while producing only a localized infection in the recipient.
The natural occurrence of cross-protective organisms of low pathogenicity seems to be a rare event and attenuated strains have usually had to be selected by laboratory manipulation. Thus the bacille Calmette-Guérin (BCG) strain of Mycobacterium bovis used to protect against human tuberculosis caused by the related species M. tuberculosis, was produced by many sequential subcultures on ox bile medium. This process resulted in deletion of many genes present in virulent M. bovis, including some essential for pathogenicity. Similarly, treatment of a virulent strain of Salmonella enterica serovar Typhi with nitrosoguanidine, which produced multiple mutations, gave rise to the live attenuated typhoid vaccine strain Ty21A. More recently developed attenuated strains of S. enterica serovar Typhi and Vibrio cholerae have been selected by directed mutagenesis processes which can produce defined mutations in specific genes.
Perhaps surprisingly, nearly all of the most successful attenuated viral vaccine strains in current use were produced by empirical methods long before the genetic basis of pathogenesis by the specific pathogen was understood. Thus, attenuated strains of polio virus for use as a live, oral vaccine (Sabin) were selected by growth of viruses isolated from human cases under cultural conditions that did not permit replication of neuropathogenic virus. Comparable procedures were used to select the attenuated virus strains that are currently used in live measles, mumps, rubella and yellow fever vaccines. A more recent approach has been to use genetic reassortants to produce live rotavirus vaccines.
Now, attenuated strains of pathogens can be selected by deliberate selective modification of genes responsible for encoding factors determining pathogenesis, such as toxins or immunomodulators, or metabolites essential for in vivo growth. Live vaccine strains can also be genetically modified by incorporating genes that encode protective antigens of other infectious agents. Several of these are under evaluation at present e.g. for vaccination against malaria and tuberculosis.
2.1.2 Killed vaccines
Killed vaccines are suspensions of bacteria, viruses or other pathogenic agents that have been killed by heat or by disinfectants such as phenol, ethanol or formaldehyde. Killed microorganisms obviously cannot replicate and cause an infection and so it is necessary for each dose of a killed vaccine to contain sufficient antigenic material to stimulate a protective immune response. Killed vaccines therefore usually have to be relatively concentrated suspensions. Even so, such preparations are often rather poorly protective, possibly because of partial destruction of protective antigens during the killing process or inadequate expression of these during in vitro culture. At the same time, because they contain all components of the microorganism they can be somewhat toxic. It is thus often necessary to divide the total amount of vaccine that is needed to induce protection into several doses that are given at intervals of a few days or weeks. Such a course of vaccination takes advantage of the enhanced ‘secondary’ response that occurs when a vaccine is administered to an individual person whose immune system has been sensitized (‘primed’) by a previous dose of the same vaccine. The best-known killed vaccines are whooping cough (pertussis), typhoid, cholera, plague, inactivated polio vaccine (Salk type) and rabies vaccine. The trend now is for these rather crude preparations to be phased out and replaced by better-defined subunit vaccines containing only relevant protective antigens, e.g. acellular pertussis and typhoid Vi polysaccharide vaccines.
2.1.3 Toxoid vaccines
Toxoid vaccines are preparations derived from the toxins that are secreted by certain species of bacteria. In the manufacture of such vaccines, the toxin is separated from the bacteria and treated chemically to eliminate toxicity without eliminating immunogenicity, a process termed ‘toxoiding’.
A variety of reagents have been used for toxoiding, but by far the most widely employed and generally successful has been formaldehyde. Under carefully controlled conditions this reacts preferentially with the amino groups of proteins although many other functional groups potentially may be affected. Ideally the toxoided protein will be rendered non-toxic but retain its immunogenicity.
The treated toxins are sometimes referred to as formol toxoids. Toxoid vaccines are very effective in the prevention of those diseases such as diphtheria, tetanus, botulism and clostridial infections of farm animals, in which the infecting bacteria produce disease through the toxic effects of secreted proteins which enzymically modify essential cellular components. Many of the clostridial toxins are lytic enzymes with very specific substrates such as neural proteins. Detoxification is also required for the pertussis toxin component of acellular pertussis vaccines.
Anthrax adsorbed vaccine is not toxoided but relies on the use of cultural conditions that favour production of the protective antigen (binding and internalization factor) rather than the lethal factor (protease) and oedema factor (adenyl cyclase) components of the toxin. Selective adsorption to aluminium hydroxide or phosphate also slows release of residual toxin.
2.1.4 Bacterial cell component vaccines
Rather than use whole cells, which may contain undesirable and potentially reactogenic components such as lipopolysaccharide endotoxins, a more precise strategy is to prepare vaccines from purified protective components. These are of two main types, proteins and capsular polysaccharides. Often more than one component may be needed to ensure protection against the full range of prevalent serotypes. The potential advantage of such vaccines is that they evoke an immune response only to the component, or components, in the vaccine and thus induce a response that is more specific and effective. At the same time, the amount of unnecessary material in the vaccine is reduced and with it the likelihood of adverse reaction. Vaccines that have been based on one or more capsular polysaccharides include Hib vaccine; the Neisseria meningitidis ACWY vaccines; the 23-valent pneumococcal polysaccharide vaccine; and the typhoid Vi vaccine. These have the disadvantage that they are T-cell-independent antigens and thus do not evoke immunological memory or effective protective responses in the very young. This problem can be overcome by chemically coupling the polysaccharides to T-cell-dependent protein carriers.
The pertussis vaccine is another example where, traditionally, whole bacterial cells have been used, but recent developments have led to an acellular pertussis vaccine that may contain detoxified toxin, either alone or combined with several other bacterial antigens.
2.1.5 Conjugate vaccines
The performance of certain types of antigen that give weak or inappropriate immune responses can often be improved by chemically conjugating them to more immunogenic carriers. Among others, polysaccharide-protein, peptide-protein, protein-protein, lipid-protein and alkaloid-protein conjugate vaccines may be prepared in this way. These have a wide range of applications, including prevention of infection, tumour therapy, fertility control and treatment of addictions. This approach has been very successful against infections caused by bacteria that produce polysaccharide capsules. The latter are T-independent antigens and induce weak responses without immunological memory. They are particularly ineffective in the very young.
2.1.6 Viral subunit vaccines
Three viral subunit vaccines are widely available, two influenza vaccines and a hepatitis B vaccine. The influenza vaccines are prepared by treating intact influenza virus particles from embryonated hens’ eggs infected with influenza virus with a surface-active agent such as a noni-onic detergent. This disrupts the virus particles, releasing the virus subunits. The two types that are required in the vaccine, haemagglutinin and neuraminidase, can be recovered and concentrated by centrifugation methods. The hepatitis B vaccine was, at one time, prepared from hepatitis B surface antigen (HbsAg) obtained from the blood of carriers of hepatitis B virus. This very constrained source of antigen has been replaced by production in yeast or mammalian cells that have been genetically engineered to express HbsAg during fermentation. The human papillomavirus (HPV) vaccines for prevention of genital warts and cervical cancer also contain recombinant viral proteins.
For further information see Ada (1994), Ellis (1999), Mizrahi (1990), Pastoret et al.(1997),Perlmann & Wigzell (1999), Plotkin, Orenstein & Offit (2008) and Powell & Newman (1995).
2.2 The seed lot system
The starting point for the production of all microbial vaccines is the isolation of the appropriate infectious agent. Such isolates have usually been derived from human infections and in some cases have yielded strains suitable for vaccine production very readily; in other instances a great deal of manipulation and selection in the laboratory have been needed before a suitable strain has been obtained. For example, bacterial strains may need to be selected for high toxin yield or production of abundant capsular polysaccharide; viral strains may need to be selected for stable attenuation or good growth in cell cultures.
Once a suitable strain is available, the practice is to grow, often from a single viable unit, a substantial volume of culture which is distributed in small amounts in a large number of ampoules and then stored at −70°C or below, or freeze-dried. This is the original seed lot. From this seed lot, one or more ampoules are used to generate the working seed from which a limited number of batches of vaccine are generated. These are first examined exhaustively in laboratory and animal tests and then, if found to be satisfactory, tested for safety and efficacy in clinical trials. Satisfactory results in the clinical trials validate the seed lot as the material from which batches of vaccine for routine use can subsequently be produced.
It is important that the full history of the seed is known, including the nature of the culture media used to propagate the strain since isolation. If at all possible, media prepared from animal products should be avoided. If this is not practicable, media components must be from sources certified free of transmissible spongiform encephalopathy (TSE) agents.
2.3 Production of the bacteria and the cellular components of bacterial vaccines
The bacteria and cellular components needed for the manufacture of most bacterial vaccines are prepared in laboratory media by well-established fermentation methods. The end-product of the fermentation, the harvest, is processed to provide a concentrated and purified bulk lot of vaccine component that may be conveniently stored for long periods or even sold to other manufacturers prior to further processing. It is important that the materials, equipment, facilities and working practices are of a standard acceptable for the manufacture of pharmaceutical products. The requirements for this are defined as Good Manufacturing Practice (GMP). Guidelines on the basic requirements have been published by WHO (1999a, 1999b).
2.4 Fermentation
The production of a bacterial vaccine batch begins with the recovery of the bacterial seed contained in an ampoule of the seed lot stored at −70°C or below, or freeze-dried. The resuscitated bacteria are first cultivated through one or more passages in preproduction media. Then, when the bacteria have multiplied sufficiently, they are used to inoculate a batch of production medium. Again, all media used must be from sources certified free of TSEs. Wherever possible, medium components of animal origin, especially human and ruminant, should be avoided.
The production medium is usually contained in a large fermenter, the contents of which are continuously stirred. Usually the pH and the oxidation/reduction potential of the medium are monitored and adjusted throughout the growth period to provide conditions that will ensure the greatest bacterial yield. In the case of rapidly growing bacteria the maximum yield is obtained after about a day but in the case of bacteria that grow slowly, e.g. M. bovis BCG, the maximum yield may not be reached before 2 weeks. At the end of the growth period the contents of the fermenter, which are known as the harvest, are ready for the next stage in the production of the vaccine
2.4.1 Processing of bacterial harvests
The harvest is a very complex mixture of bacterial cells, metabolic products and exhausted medium. In the case of a live attenuated vaccine it should be innocuous, and all that is necessary is for the bacteria to be separated and resuspended under aseptic conditions in an appropriate diluent, possibly for freeze-drying. In a vaccine made from a virulent strain of pathogen the harvest may be intensely dangerous and great care is necessary in the subsequent processing. Adequate containment will be required and for class 3 pathogens such as S. enterica serovar Typhi or Yersinia pestis or bulk production of bacterial toxins, dedicated facilities that will provide complete protection for the operators and the environment are essential.
• Killing. This is the process by which heat and disinfectants are used to render the live bacteria in the culture non-viable and harmless. Heat and/or formalin or thiomersal are used to kill the cells of Bordetella pertussis used to make whole-cell pertussis vaccines, whereas phenol was used to kill the V. cholerae and the S. enterica serovar Typhi cells used in the now obsolete whole-cell cholera and typhoid vaccines.
• Separation. The process by which the bacterial cells are separated from the culture fluid and soluble products. Centrifugation using either a batch or continuous flow process, or ultrafiltration, is commonly used. Precipitation of the cells by reducing the pH has been used as an alternative. In the case of vaccines prepared from cells, the supernatant fluid is discarded and the cells are resuspended in a saline diluent; where vaccines are made from a constituent of the supernatant fluid, the cells are discarded.
• Fractionation. This is the process by which components are extracted from bacterial cells or from the medium in which the bacteria are grown and obtained in a purified form. The polysaccharide antigens of N.meningitidis are usually separated from the bacterial cells by treatment with hexadecyltrimethylammonium bromide followed by extraction with calcium chloride and selective precipitation with ethanol. Those of Streptococcus pneumoniae are usually extracted with sodium deoxycholate, deproteinized and then fractionally precipitated with ethanol. The purity of an extracted material may be improved by resolubilization in a suitable solvent and reprecipitation. These procedures are often supplemented with filtration through membranes or ultrafilters with specific molecular size cut-off points. After purification, a component may be freeze-dried, stored indefinitely at low temperature and, as required, incorporated into a vaccine in precisely weighed amount at the blending stage.
• Detoxification. The process by which bacterial toxins are converted to harmless toxoids. Formaldehyde is used to detoxify the toxins of Corynebacterium diphtheriae, Clostridium botulinum and Cl. tetani. The detoxification may be performed either on the whole culture in the fermenter or on the purified toxin after fractionation. Traditionally the former approach has been adopted, as it is much safer for the operator. However, the latter gives a purer product. The pertussis toxin used in acellular vaccines may be detoxified with formaldehyde, glutaraldehyde, or both, hydrogen peroxide or tetranitromethane. In the case of genetically detoxified pertussis toxin, a treatment with a low concentration of formaldehyde is still performed to stabilize the protein.
• Further processing. This may include physical or chemical treatments to modify the product. For example polysaccharides may be further fractionated to produce material of a narrow molecular size specification. They may then be activated and conjugated to carrier proteins to produce glycoconjugate vaccines. Further purification may be required to eliminate unwanted reactants and by-products. These processes must be done under conditions that minimize extraneous microbial contamination. If sterility is not achievable then strict bioburden limits are imposed.
• Adsorption.This describes the adsorption of the components of a vaccine on to a mineral adjuvant or carrier (aluminium hydroxide or aluminium phosphate; rarely calcium phosphate). Their effect is to increase the immunogenicity and decrease the toxicity, local and systemic, of a vaccine. Diphtheria vaccine, tetanus vaccine, diphtheria/tetanus vaccine and diphtheria/tetanus/pertussis (whole-cell or acellular) vaccine, are generally prepared as adsorbed vaccines.
• Conjugation. The linking of a vaccine component that induces an inadequate immune response with a vaccine component that induces a good immune response. For example, the immunogenicity for infants of the capsular polysaccharide of H. influenzae type b is greatly enhanced by the conjugation of the polysaccharide with diphtheria or tetanus toxoid, or with the outer-membrane protein of N. meningitidis. More recently, in attempts to improve efficacy, protein carriers that themselves induce a protective immune response against the pathogen have been conjugated to the capsular polysaccharide e.g. Panton-Valentine leucocidin conjugated to Staph. aureus capsular polysaccharide.
2.5 Production of the viruses and the components of viral vaccines
Viruses replicate only in living cells, so the first viral vaccines were necessarily made in animals: smallpox vaccine in the dermis of calves and sheep, and rabies vaccines in the spinal cords of rabbits and brains of mice. Such methods are no longer used in advanced vaccine production; the only intact animal hosts that are still used are embryonated hens’ eggs. Almost all the virus that is needed for viral vaccine production is obtained from cell cultures infected with virus of the appropriate strain.
2.5.1 Growth of viruses
Embryonated hens’ eggs are still the most convenient hosts for growth of the viruses that are needed for influenza and yellow fever vaccines. Influenza viruses accumu-late in high titre in the allantoic fluid of the eggs, and yellow fever virus accumulates in the nervous system of the embryos. A few bacterial vaccines, e.g. against rickettsial and chlamydial agents, are also prepared in embryonated eggs. It is important to use eggs from disease-free flocks and emphasis is placed on screening the latter for various avian viruses. The allantoic fluid or embryos must be harvested under conditions that minimize extraneous microbial contamination.
Where cell cultures are used for virus production, they must be of known origin, obtained from validated sourcesand shown to be free of extraneous agents. The mediaused in their production should not contain componentsof human or animal origin, unless the latter are from TSE-free sources.
2.5.2 Processing of viral harvests
The processing of the virus-containing material from infected embryonated eggs may take one of several forms. In the case of influenza vaccines the allantoic fluid is centrifuged to provide a concentrated and partially purified suspension of virus. This concentrate is treated with organic solvent or detergent to split the virus into its components when split virion or surface antigen vaccines are prepared. The chick embryos used in the production of yellow fever vaccine are homogenized in sterile water to provide a virus-containing pulp. Centrifugation then precipitates most of the embryonic debris and leaves much of the yellow fever virus in an aqueous suspension. Further purification can then be performed as required.
Cell cultures provide infected fluids that contain little debris and can generally be satisfactorily clarified by filtration. Because most viral vaccines made from cell cultures consist of live attenuated virus, there is no inactivation stage in their manufacture. There are, however, two important exceptions: inactivated poliomyelitis virus vaccine is inactivated with dilute formaldehyde or β-propiolactone and rabies vaccine is inactivated with β-propiolactone. The preparation of these inactivated vaccines also involves a concentration stage—by adsorption and elution of the virus in the case of poliomyelitis vaccine and by ultrafiltration in the case of rabies vaccine. When processing is complete the bulk materials may be stored until needed for blending into final vaccine. Because of the lability of many viruses, however, it is necessary to store most purified materials at temperatures of −70°C.
2.6 Blending
Blending is the process in which the various components of a vaccine are mixed to form a final bulk. It is undertaken in a large, closed vessel fitted with a stirrer and ports for the addition of constituents and withdrawal of the final blend. When bacterial vaccines are blended, the active constituents usually need to be greatly diluted and the vessel is first charged with the diluents, usually containing a preservative. Thiomersal has been widely used in the past but is now being phased out and replaced by phenoxyethanol or alternatives. A single-component final bulk is made by adding bacterial suspension, bacterial component or concentrated toxoid in such quantity that it is at the required concentration in the final product. A multiple-component final bulk of a combined vaccine is made by adding each required component in sequence. When viral vaccines are blended, the need to maintain adequate antigenicity or infectivity may preclude dilution, and tissue culture fluids, or concentrates made from them, are often used undiluted or, in the case of multicomponent vaccines, merely diluted one with another. After thorough mixing, a final bulk may be divided into a number of moderate-sized volumes to facilitate handling.
2.7 Filling and drying
As vaccine is required to meet orders, bulk vaccine is distributed into single-dose ampoules or into multidose vials as necessary. Vaccines that are filled as liquids are sealed and capped in their containers, whereas vaccines that are provided as dried preparations are freeze-dried before sealing.
The single-component bacterial vaccines are listed in Table 24.1. For each vaccine, notes are provided of the basic material from which the vaccine is made, the salient production processes and tests for potency and for safety. The multicomponent vaccines that are made by blending together two or more of the single-component vaccines are required to meet the potency and safety requirements for each of the single components that they contain. The best-known of the combined bacterial vaccines is the adsorbed diphtheria, tetanus and pertussis vaccine (DTPer/Vac/Ads) that is used to immunize infants, and the adsorbed diphtheria and tetanus vaccine (DT/Vac/Ads) that is used to reinforce the immunity of school entrants. The trend is to produce increasingly complex combinations, and hepta-and octavalent preparations are now available.
Table 24.1 Bacterial vaccines used for the prevention of infectious disease in humans
Diphtheria and pertussis vaccines are seldom used as single-component vaccines but as components of diphtheria/tetanus vaccines and diphtheria/tetanus/pertussis vaccines. Combined diphtheria/tetanus/pertussis/Hib and diphtheria/tetanus/pertussis/Hep B vaccines with or without inactivated polio vaccine are available.
Bacterial vaccines of restricted availability include anthrax, botulism, cholera, plague, Q fever, typhus and tularaemia vaccines.
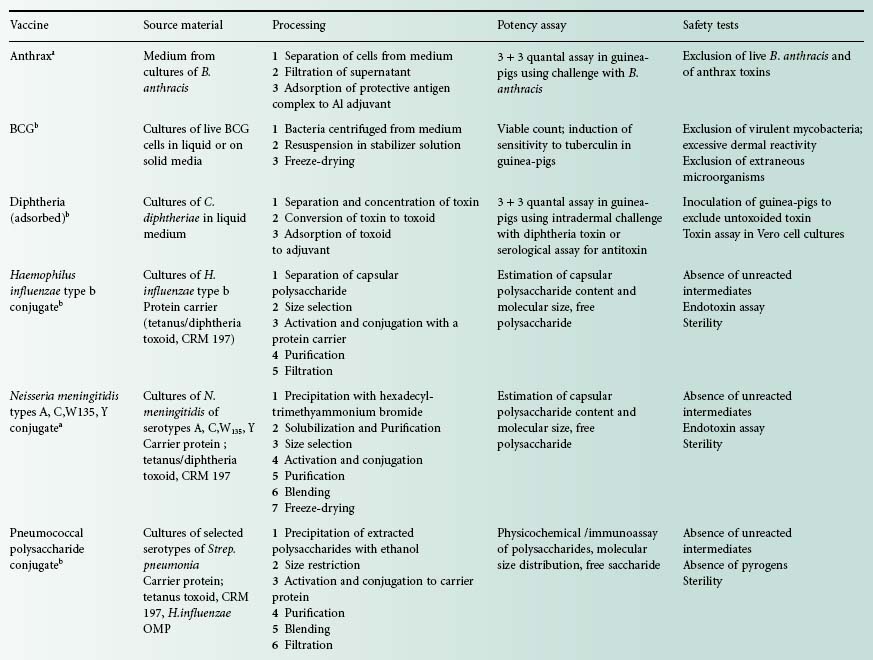
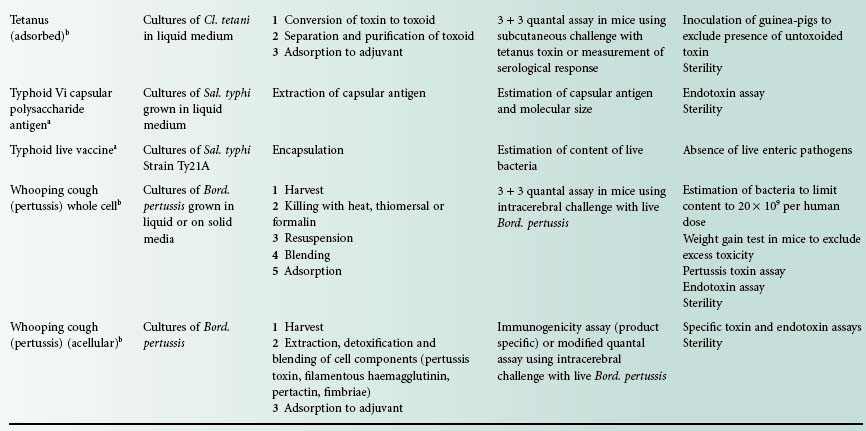
aVaccines used to provide additional protection when circumstances indicate a need.
bVaccines used in conventional immunization schedules.
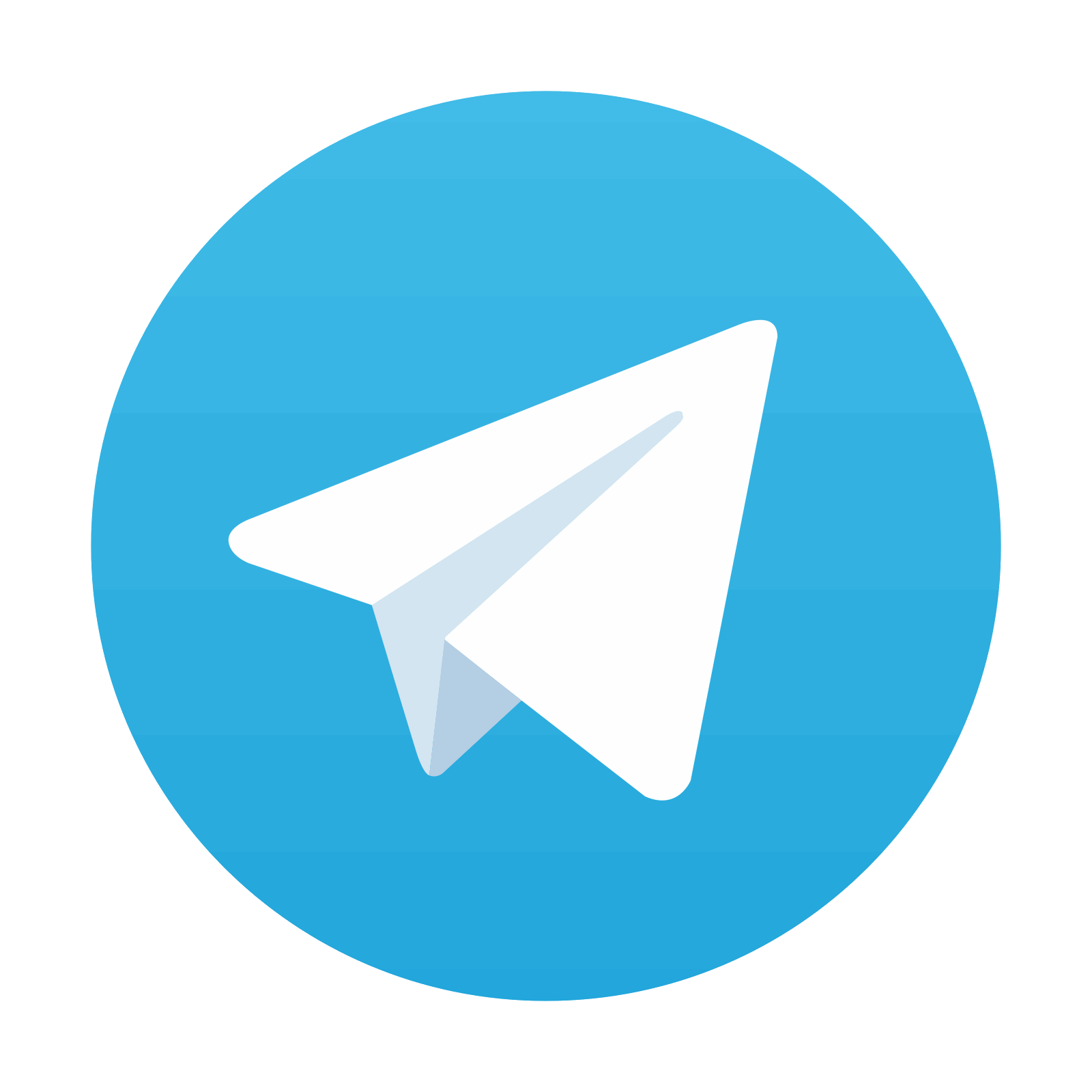