9 The innate defences of the body
The body has both ‘innate’ and ‘adaptive’ immune defences
When an organism infects the body, the defence systems already in place may well be adequate to prevent replication and spread of the infectious agent, thereby preventing development of disease. These established mechanisms are referred to as constituting the ‘innate’ immune system. However, should innate immunity be insufficient to parry the invasion by the infectious agent, the so-called ‘adaptive’ immune system then comes into action, although it takes time to reach its maximum efficiency (Fig. 9.1). When it does take effect, it generally eliminates the infective organism, allowing recovery from disease.
The contrasts between these two systems are set out in Table 9.1. On the one hand, the soluble factors such as lysozyme and complement, together with the phagocytic cells, contribute to the innate system, while on the other the lymphocyte-based mechanisms that produce antibody and T lymphocytes are the main elements of the adaptive immune system. Not only do these lymphocytes provide improved resistance by repeated contact with a given infectious agent, but the memory with which they become endowed shows very considerable specificity to that infection. For instance, infection with measles virus will induce a memory to that microorganism alone and not to another virus such as rubella.
Table 9.1 Comparison of innate and adaptive effector immune systems
Innate immune system | Adaptive immune system | |
---|---|---|
Major elements | ||
Soluble factors | Lysozyme, complement, acute phase proteins, e.g. C-reactive protein, interferon | Antibody |
Cells | Phagocytes Natural killer cells | T lymphocytes |
Response to microbial infection | ||
First contact | + | + + |
Second contact | + | + + + + |
Non-specific; no memory Resistance not improved by repeated contact | Specific; memory Resistance improved by repeated contact |
Innate immunity is sometimes referred to as ‘natural’, and adaptive as ‘acquired’. There is considerable interaction between the two systems. ‘Humoral’ immunity due to soluble factors contrasts with immunity mediated by cells. Primary contact with antigen produces both adaptive and innate responses, but if the same antigen persists or is encountered a second time the specific adaptive response to that antigen is much enhanced.
Defences against entry into the body
A variety of biochemical and physical barriers operate at the body surfaces
Before an infectious agent can penetrate the body, it must overcome biochemical and physical barriers that operate at the body surfaces. One of the most important of these is the skin, which is normally impermeable to the majority of infectious agents. Many bacteria fail to survive for long on the skin because of the direct inhibitory effects of lactic acid and fatty acids present in sweat and sebaceous secretions and the lower pH to which they give rise (Fig. 9.2). However, should there be skin loss, as can occur in burns, for example, infection becomes a major problem.
Defences once the microorganism penetrates the body
• the mechanism of phagocytosis, involving engulfment and killing of microorganisms by specialized cells, the ‘professional phagocytes’
• the destructive effect of soluble chemical factors, such as bactericidal enzymes.
Two types of professional phagocyte
Perhaps because of the belief that professionals do a better job than amateurs, the cells that shoulder the main burden of our phagocytic defences have been labelled ‘professional phagocytes’. These consist of two major cell families, as originally defined by Elie Metchnikoff, the Russian zoologist (Box 9.1; Fig. 9.3):
• the smaller polymorphonuclear granulocytes, which are generally referred to as polymorphs or neutrophils because their cytoplasmic granules do not stain with haematoxylin and eosin.
Macrophages are widespread throughout the tissues
Macrophages originate as bone marrow promonocytes, which develop into circulating blood monocytes (Fig. 9.4) and finally become the mature macrophages, which are widespread throughout the tissues and collectively termed the ‘mononuclear phagocyte system’ (Fig. 9.5). These macrophages are present throughout the connective tissue and are associated with the basement membrane of small blood vessels. They are particularly concentrated in the lung (alveolar macrophages), liver (Kupffer cells) and the lining of lymph node medullary sinuses and splenic sinusoids (Fig. 9.6), where they are well placed to filter off foreign material (Fig. 9.7). Other examples are the brain microglia, kidney mesangial cells, synovial A cells and osteoclasts in bone. In general, these are long-lived cells that depend upon mitochondria for their metabolic energy and show elements of rough-surfaced endoplasmic reticulum (Fig. 9.8) related to the formidable array of different secretory proteins that these cells generate.

Figure 9.5 The mononuclear phagocyte system. Tissue macrophages are derived from blood monocytes, which are manufactured in the bone marrow. (The numbers relate to those in Fig. 9.6.)
Polymorphs possess a variety of enzyme-containing granules
The polymorph is the dominant white cell in the bloodstream and, like the macrophage, shares a common haemopoietic stem cell precursor with the other formed elements of the blood. It has no mitochondria, but uses its abundant cytoplasmic glycogen stores for its energy requirements; therefore, glycolysis enables these cells to function under anaerobic conditions, such as those in an inflammatory focus. The polymorph is a non-dividing, short-lived cell, with a segmented nucleus; the cytoplasm is characterized by an array of granules, which are illustrated in Figure 9.9.
Azurophil granules | Specific granules |
---|---|
0.5 μm | 0.2 μm |
1500/cell | 3000/cell |
Lysozyme | Lysozyme |
Myeloperoxidase | Cytochrome b558 |
Elastase | OH phosphatase |
Cathepsin G | Lactoferrin |
H+ hydrolases | |
Defensins | Vitamin B12 binding protein |
BPI (bactericidal permeability increasing protein) |
Phagocytosis and killing
Phagocytes recognize pathogen-associated molecular patterns (PAMPs)
The first event in the uptake and digestion of a microorganism by the professional phagocyte involves the attachment of the microbe to the surface of the cell through the recognition of repeating pathogen-associated molecular patterns (PAMPs) on the microbe by pattern recognition receptors (PRRs) on the phagocyte surface (Fig. 9.10). A major subset of these PRRs belongs to the class of so-called ‘Toll-like receptors’ (TLRs) because of their similarity to the Toll receptor in the fruit fly, Drosophila, which, in the adult, triggers an intracellular cascade generating the expression of antimicrobial peptides in response to microbial infection. A series of cell surface TLRs acting as sensors for extracellular infections have been identified (Fig. 9.11) which are activated by microbial elements such as peptidoglycan, lipoproteins, mycobacterial lipoarabinomannan, yeast zymosan and flagellin. Other PRRs displayed by phagocytes include the cell bound ‘C-type (calcium-dependent) lectins’, of which the macrophage mannose receptor is an example, and ‘scavenger receptors’, which recognize a variety of anionic polymers and acetylated low density proteins. Examples of intracellular PAMPs are the unmethylated guanosine-cytosine (CpG) sequences of bacterial DNA and double-stranded RNA from RNA viruses.
The phagocyte is activated through PAMP recognition
The attached microbe may then signal through the phagocyte receptors to initiate the ingestion phase by activating an actin-myosin contractile system, which sends arms of cytoplasm around the particle until it is completely enclosed within a vacuole (phagosome; Fig. 9.12; see Fig. 9.11). Shortly afterwards, the cytoplasmic granules fuse with a phagosome and discharge their contents around the incarcerated microorganism.
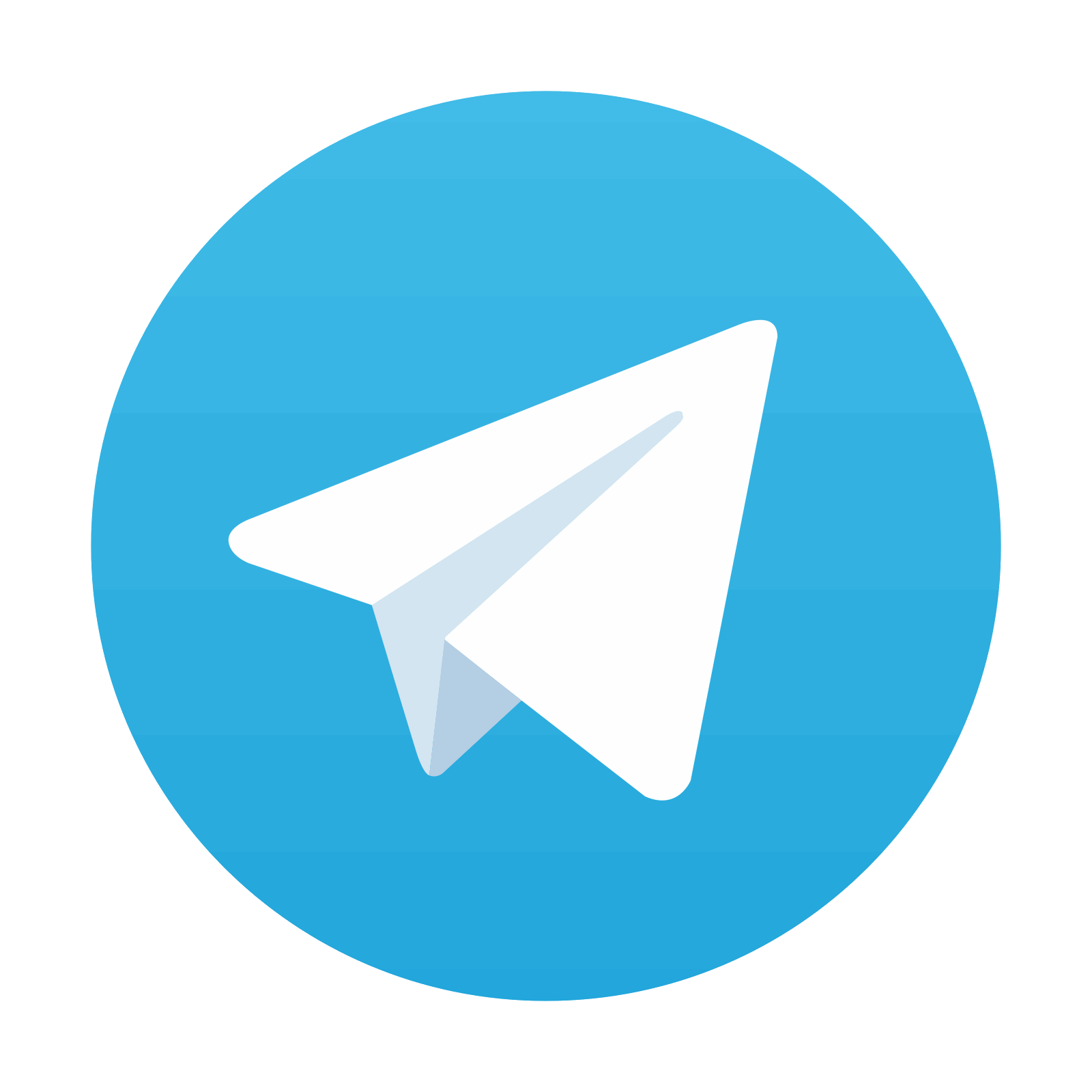
Stay updated, free articles. Join our Telegram channel

Full access? Get Clinical Tree
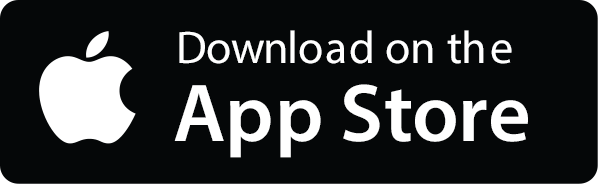
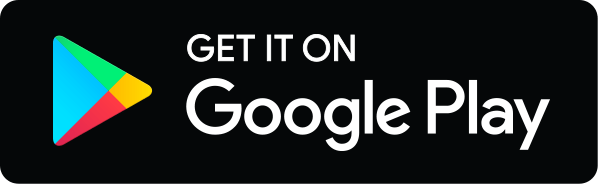