Many of the cells in tissues are embedded in an extracellular matrix that fills the spaces between cells and binds cells and tissue together. In so doing, the extracellular matrix aids in determining the shape of tissues as well as the nature of the partitioning between tissue types. In the skin, loose connective tissue beneath epithelial cell layers consists of an extracellular matrix in which fibroblasts, blood vessels, and other components are distributed (Fig. 47.1). Other types of connective tissue, such as tendon and cartilage, consist largely of extracellular matrix, which is principally responsible for their structure and function. This matrix also forms the sheetlike basal laminae, or basement membranes, on which layers of epithelial cells rest, and which act as supportive tissue for muscle cells, adipose cells, and peripheral nerves.
FIGURE 47.1 An overview of connective tissue extracellular matrix. Supporting the epithelial cell layer is a basal lamina, beneath which are collagen, elastic fibers, and proteoglycans. The cell types present in connective tissue, such as fibroblasts and macrophages, have been removed from the diagram for clarity.
Basic components of the extracellular matrix include fibrous structural proteins, such as collagens, proteoglycans containing long glycosaminoglycan chains attached to a protein backbone, and adhesion proteins linking components of the matrix to each other and to cells.
These fibrous structural proteins are composed of repeating elements that form a linear structure. Collagens, elastin, and laminin are the principal structural proteins of connective tissue.
Proteoglycans consist of a core protein covalently attached to many long, linear chains of glycosaminoglycans, which contain repeating disaccharide units. The repeating disaccharides usually contain a hexosamine and a uronic acid, and these sugars are frequently sulfated. Synthesis of the proteoglycans starts with the attachment of a sugar to a serine, threonine, or asparagine residue of the protein. Additional sugars, donated by UDP-sugar precursors, add sequentially to the nonreducing end of the molecule.
Proteoglycans, as is the case for glycoproteins and glycolipids, are synthesized in the endoplasmic reticulum (ER) and the Golgi complex. The glycosaminoglycan chains of proteoglycans are degraded by lysosomal enzymes that cleave one sugar at a time from the nonreducing end of the chain. An inability to degrade proteoglycans leads to a set of diseases known as the mucopolysaccharidoses.
Adhesion proteins, such as fibronectin and laminin, are extracellular glycoproteins that contain separate distinct binding domains for proteoglycans, collagen, and fibrin. These domains allow these adhesion proteins to bind the various components of the extracellular matrix. They also contain specific binding domains for cell surface receptors known as integrins. These integrins bind to fibronectin on the external surface, span the plasma membrane of cells, and adhere to proteins, which, in turn, bind to the intracellular actin filaments of the cytoskeleton. Integrins also provide a mechanism for signaling between cells via both internal signals and through signals generated via the extracellular matrix.
Cell movement within the extracellular matrix requires remodeling of the various components of the matrix. This is accomplished by a variety of matrix metalloproteinases (MMPs) and regulators of the MMPs, tissue inhibitors of matrix metalloproteinases (TIMPs). Dysregulation of this delicate balance of the regulators of cell movement allows cancer cells to travel to other parts of the body (metastasize) as well as to spread locally to contiguous tissues.
THE WAITING ROOM 
Sarah L. (first introduced in Chapter 14) noted a moderate reduction in pain and swelling in the joints of her fingers while she was taking her immunosuppressant medication. At her next checkup, her rheumatologist described to Sarah L. the underlying inflammatory tissue changes that her systemic lupus erythematosus (SLE) was causing in the joint tissues.
Deborah S., a patient with type 2 diabetes (see Chapter 34), complained of a declining appetite for food as well as severe weakness and fatigue. The reduction in her kidneys’ ability to maintain normal daily total urinary net acid excretion contributed to a metabolic acidosis. This, plus her declining ability to excrete nitrogenous waste products, such as creatinine and urea, into her urine (“azotemia”) were responsible for many of her symptoms. Her serum creatinine level was rising steadily. As it approached a level of 4 mg/dL, she developed a litany of complaints caused by the multisystem dysfunction associated with her worsening metabolic acidosis and retention of nitrogenous waste products (“uremia”). Her physicians discussed with Deborah S. the need to consider peritoneal dialysis or hemodialysis.
I. Composition of the Extracellular Matrix
A. Fibrous Proteins
1. Collagen
Collagen, a family of fibrous proteins, is produced by a variety of cell types but principally by fibroblasts (cells found in interstitial connective tissue), muscle cells, and epithelial cells. Type I collagen, collagen(I), the most abundant protein in mammals, is a fibrous protein that is the major component of connective tissue. It is found in the extracellular matrix (ECM) of loose connective tissue, bone, tendons, skin, blood vessels, and the cornea of the eye. Collagen(I) contains approximately 33% glycine and 21% proline and hydroxyproline. Hydroxyproline is an amino acid produced by posttranslational modification of peptidyl proline residues.
Procollagen(I), the precursor of collagen(I), is a triple helix composed of three polypeptide (pro-α) chains that are twisted around each other, forming a ropelike structure. Polymerization of collagen(I) molecules forms collagen fibrils, which provide great tensile strength to connective tissues (see Fig. 7.22). The individual polypeptide chains each contain approximately 1,000 amino acid residues. The three polypeptide chains of the triple helix are linked by interchain hydrogen bonds. Each turn of the triple helix contains three amino acid residues, such that every third amino acid is in close contact with the other two strands in the center of the structure. Only glycine, which lacks a side chain, can fit in this position, and indeed, every third amino acid residue of collagen is glycine. Thus, collagen is a polymer of (Gly-X-Y) repeats, where Y is frequently proline or hydroxyproline and X is any other amino acid found in collagen.
Procollagen(I) is an example of a protein that undergoes extensive posttranslational modifications. Hydroxylation reactions produce hydroxyproline residues from proline residues and hydroxylysine from lysine residues. These reactions occur after the protein has been synthesized (Fig. 47.2) and require vitamin C (ascorbic acid) as a cofactor of the enzymes prolyl hydroxylase and lysyl hydroxylase. Hydroxyproline residues are involved in hydrogen bond formation that helps to stabilize the triple helix, whereas hydroxylysine residues are the sites of attachment of disaccharide moieties (galactose-glucose). The role of carbohydrates in collagen structure is still controversial. In the absence of vitamin C (scurvy), the melting temperature of collagen drops from 42°C to 24°C because of the loss of interstrand hydrogen bond formation, which is in turn caused by the lack of hydroxyproline residues.
FIGURE 47.2 Hydroxylation of proline and lysine residues in collagen. Proline and lysine residues within the collagen chains are hydroxylated by reactions that require vitamin C.
The side chains of lysine residues also may be oxidized to form the aldehyde, allysine. These aldehyde residues produce covalent cross-links between collagen molecules (Fig. 47.3). An allysine residue on one collagen molecule reacts with the amino group of a lysine residue on another molecule, forming a covalent Schiff base that is converted to more stable covalent cross-links. Aldol condensation also may occur between two allysine residues, which forms the structure lysinonorleucine.
FIGURE 47.3 Formation of cross-links in collagen. A. Lysine residues are oxidized to allysine (an aldehyde). Allysine may react with an unmodified lysine residue to form a Schiff base (B), or two allysine residues may undergo an aldol condensation (C).
a. Types of Collagen
At least 28 different types of collagen have been characterized (Table e-47.1) . Although each type of collagen is found only in particular locations in the body, more than one type may be present in the ECM at a given location. The various types of collagen can be classified as fibril-forming (types I, II, III, V, XI, XXIV, and XXVII), network-forming (types IV, VIII, and X), those that associate with fibril surfaces (types IX, XII, XIV, XXI, and XXII), those that are transmembrane proteins (types XIII, XVII, XXIII, and XXV), endostatin-forming (types XV and XVIII), and those that form periodic beaded filaments (type VI).
TABLE 47.1 Steps in Collagen Biosynthesis
LOCATION | PROCESS |
Rough endoplasmic reticulum | Synthesis of preprocollagen; insertion of the procollagen molecule into the lumen of the ER |
Lumen of ER | Hydroxylation of proline and lysine residues; glycosylation of selected hydroxylysine residues |
Lumen of ER and Golgi apparatus | Self-assembly of the procollagen molecule, initiated by disulfide bond formation in the carboxyl-terminal extensions; triple helix formation |
Secretory vesicle | Procollagen prepared for secretion from cell |
Extracellular | Cleavage of the propeptides, removing the amino- and carboxyl-terminal extensions, and self-assembly of the tropocollagen molecules into fibrils, and then fibers |
ER, endoplasmic reticulum.
All collagens contain three polypeptide chains with at least one stretch of triple helix. The non–triple helical domains can be short (as in the fibril-forming collagens), or they can be rather large, such that the triple helix is actually a minor component of the overall structure (examples are collagen types XII and XIV). The FACIT (fibril-associated collagens with interrupted triple helices, collagen types IX, XII, and XIV) collagen types associate with fibrillar collagens, without themselves forming fibers. The endostatin-forming collagens are cleaved at their C-terminus to form endostatin, an inhibitor of angiogenesis. The network-forming collagens (type IV) form a meshlike structure, because of large (~230 amino acids) noncollagenous domains at the carboxy terminus (Fig. 47.4). And finally, a number of collagen types are actually transmembrane proteins (XIII, XVII, XXIII, and XXV) found on epithelial or epidermal cell surfaces, which play a role in a number of cellular processes, including adhesion of components of the ECM to cells embedded within it. Type XXV collagen has been associated with the neuronal plaques, which develop during Alzheimer disease.
FIGURE 47.4 Type IV collagen contains a globular carboxyl-terminal domain (A), which forms tropocollagen dimers (hexamers of collagen, B). Four dimers associate at the amino-terminal domains to form a 7S domain (C), and the tetramers form a lattice (D), which provides structural support to the basal lamina.
The types of collagen that do not form fibrils perform a series of distinct roles. Fibril-associated collagens bind to the surface of collagen fibrils and link them to other matrix-forming components. The transmembrane collagens form anchoring fibrils that link components of the extracellular matrix to underlying connective tissue. The network-forming collagens (type IV) form a flexible collagen that is part of the basement membrane and basal lamina that surround many cells.
b. Synthesis and Secretion of Collagen
Collagen is synthesized within the ER as a precursor known as preprocollagen. The presequence acts as the signal sequence for the protein and is cleaved, forming procollagen within the ER. From there, it is transported to the Golgi apparatus (Table 47.1). Three procollagen molecules associate through the formation of inter- and intrastrand disulfide bonds at the carboxy terminus; once these disulfides are formed, the three molecules can align properly to initiate the formation of the triple helix. The triple helix forms from the carboxy end toward the amino end, forming procollagen. The procollagen contains a triple-helical segment between two globular ends, the amino- and carboxyl-terminal extensions. The procollagen is secreted from the cell, the extensions are removed using extracellular proteases, and the tropocollagen takes its place within the ECM. The individual fibrils of tropocollagen line up in a highly ordered fashion to form the collagen fiber.
2. Elastin
Elastin is the major protein found in elastic fibers, which are located in the ECM of connective tissue of smooth muscle cells, endothelial and microvascular cells, chondrocytes, and fibroblasts. Elastic fibers allow tissues to expand and contract; this is of particular importance to blood vessels, which must deform and reform repeatedly in response to the changes in intravascular pressure that occur with the contraction of the left ventricle of the heart. It is also important for the lungs, which stretch each time a breath is inhaled and return to their original shape with each exhalation. In addition to elastin, the elastic fibers contain microfibrils, which are composed of a number of acidic glycoproteins, the major ones being fibrillin-1 and fibrillin-2.
a. Tropoelastin
Elastin has a highly cross-linked, insoluble, amorphous structure. Its precursor, tropoelastin, is a molecule of high solubility, which is synthesized on the rough endoplasmic reticulum (RER) for eventual secretion. Tropoelastin contains two types of alternating domains. The first domain consists of a hydrophilic sequence that is rich in lysine and alanine residues. The second domain consists of a hydrophobic sequence that is rich in valine, proline, and glycine, which frequently occur in repeats of VPGVG or VGGVG. The protein contains approximately 16 regions of each domain, alternating throughout the protein (Fig. 47.5).
FIGURE 47.5 The cDNA encoding elastin, indicating the repeating cross-linking and hydrophobic domains.
Upon secretion from the cell, the tropoelastin is aligned with the microfibrils, and lysyl oxidase initiates the reactions that cross-link elastin molecules, using lysine residues within the hydrophilic alternating domains in the proteins. This cross-linking reaction is the same as that which occurs in collagen. In this reaction, two, three, or four lysine residues are cross-linked to form a stable structure. The net result of the cross-linking is the generation of a fibrous mesh that encircles the cells.
b. Elastic Properties of Elastin
Elastic fibers have the ability to stretch and then to re-form without requiring an obvious energy source to do so. The mechanism by which this stretching and relaxing actively occurs is still controversial but does relate to the basic principles of protein folding described in Chapter 7. When the elastic fibers are stretched (such as when a breath is taken in and the lung fills up with air), the amorphous elastin structure is stretched. This stretching exposes the repeating hydrophobic regions of the molecule to the aqueous environment. This, in turn, leads to a decrease in the entropy of water, because the water molecules need to rearrange to form cages about each hydrophobic domain. When this stretching force within the lung is removed (e.g., when the subject exhales), the elastin takes on its original structure because of the increase in entropy that occurs because the water no longer needs to form cages about hydrophobic domains. Thus, the hydrophobic effect is the primary force that allows this stretched structure to reform. Elastin is inherently stable, with a half-life of up to 70 years.
3. Laminin
After type IV collagen, laminin is the most abundant protein in basal laminae. Laminin provides additional structural support for the tissues through its ability to bind to type IV collagen, to other molecules present in the ECM, and also to cell surface–associated proteins (the integrins; see Section I.D).
a. Laminin Structure
Laminin is a heterotrimeric protein that is shaped, for the most part, like a cross (Fig. 47.6). The trimer is composed of α-, β, and γ-subunits. There are five possible α-proteins (designated α1 through α5), three different versions of the β-subunit (β1 through β3), and three different γ-forms (γ1 through γ3). Thus, there is a potential for the formation of as many as 45 different combinations of these three subunits. However, only 18 have been discovered. Laminin 111, composed of α1β1γ1, is typical of this class of proteins. The major feature of the laminin structure is a coiled α-helix, which joins the three subunits together and forms a rigid rod. All three chains have extensions at the amino-terminal end. Only the α-chain has a significant carboxyl-terminal extension past the rod-like structure. It is the laminin extensions that allow laminin to bind to other components within the ECM and to provide stability for the structure. Components of the ECM that are bound by laminin include collagen, sulfated lipids, and proteoglycans.
FIGURE 47.6 The structure of laminin.
b. Laminin Biosynthesis
Like other secreted proteins, laminin is synthesized with a leader sequence that targets the three chains to the ER. Chain association occurs within the Golgi apparatus before secretion from the cell. After laminin is secreted by the cell, the amino-terminal extensions promote self-association as well as the binding to other ECM components. Disulfide linkages are formed to stabilize the trimer, but there is much less posttranslational processing of laminin than there is of collagen and elastin.
B. Proteoglycans
The fibrous structural proteins of the ECM are embedded in gels formed from proteoglycans. Proteoglycans consist of polysaccharides called glycosaminoglycans (GAGs) linked to a core protein. The GAGs are composed of repeating units of disaccharides (Fig. 47.7). One sugar of the disaccharide is either N-acetyl glucosamine or N-acetyl galactosamine, and the second is usually acidic (either glucuronic acid or iduronic acid). These sugars are modified by the addition of sulfate groups to the parent sugar. A proteoglycan may contain >100 GAG chains and consist of up to 95% carbohydrate by weight.
FIGURE 47.7 Repeating disaccharides of some glycosaminoglycans. These repeating disaccharides usually contain an N-acetylated sugar and a uronic acid, which usually is glucuronic acid or iduronic acid. Sulfate groups are often present and are included in the sugar names in this figure. Iduronic acid and glucuronic acid are epimers at position 5 of the sugar.
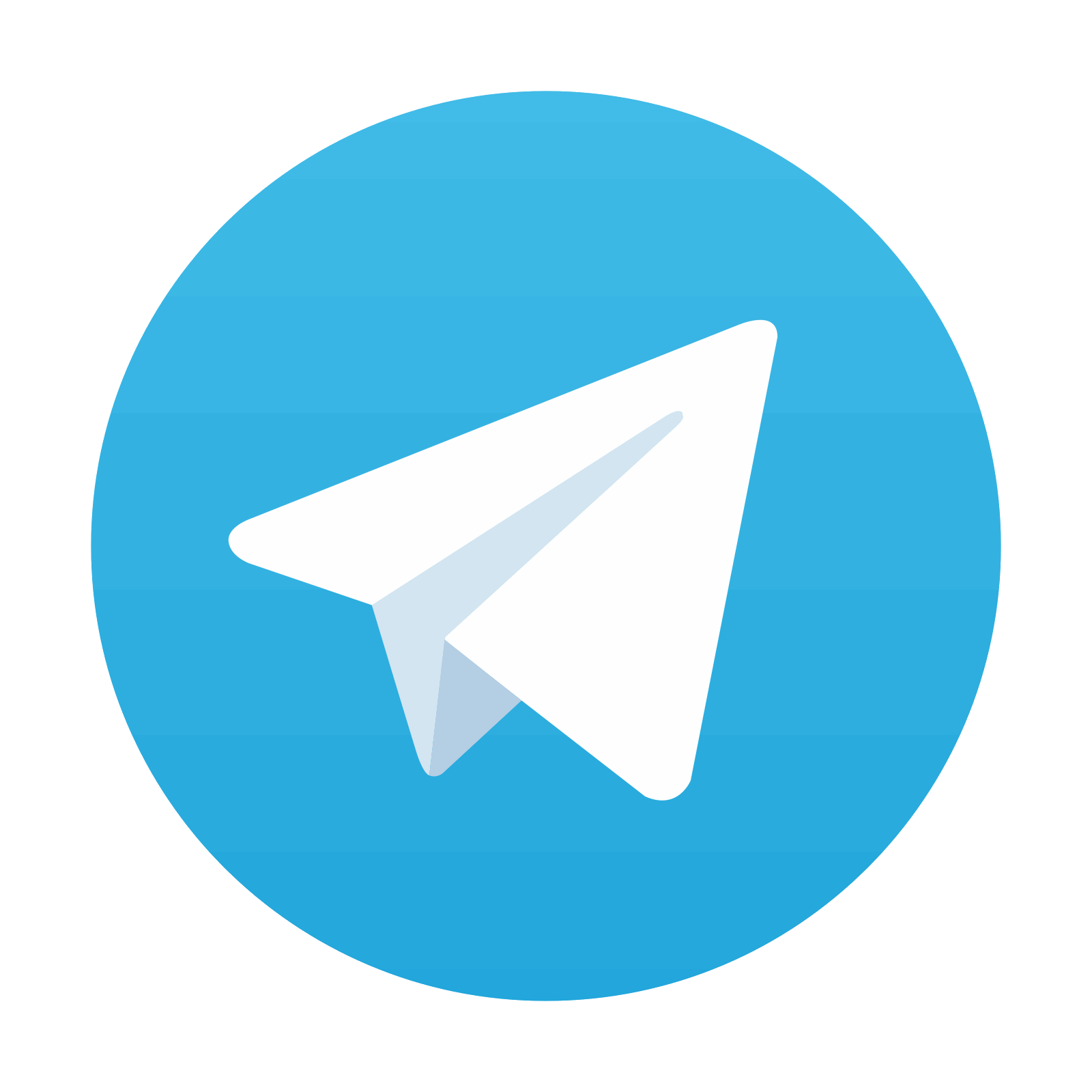
Stay updated, free articles. Join our Telegram channel

Full access? Get Clinical Tree
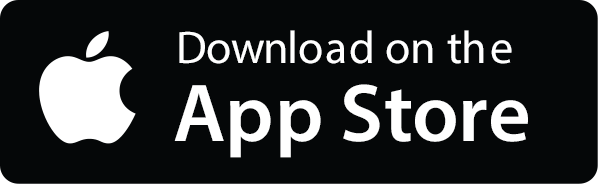
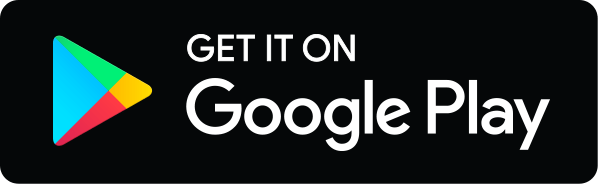