Ethanol is a dietary fuel that is metabolized to acetate principally in the liver, with the generation of reduced nicotinamide adenine dinucleotide (NADH). The principal route for metabolism of ethanol is through hepatic alcohol dehydrogenases, which oxidize ethanol to acetaldehyde in the cytosol (Fig. 33.1). Acetaldehyde is further oxidized by acetaldehyde dehydrogenases to acetate, principally in mitochondria. Acetaldehyde, which is toxic, also may enter the blood. NADH produced by these reactions is used for adenosine triphosphate (ATP) generation through oxidative phosphorylation. Most of the acetate enters the blood and is taken up by skeletal muscles and other tissues, where it is activated to acetyl coenzyme A (acetyl CoA) and is oxidized in the tricarboxylic acid (TCA) cycle.
FIGURE 33.1 The major route for metabolism of ethanol and use of acetate by the muscle. Acetyl CoA, acetyl coenzyme A; ACS, acetyl CoA synthetase; ADH, alcohol dehydrogenase; ALDH, acetaldehyde dehydrogenase; FAD, flavin adenine dinucleotide; NAD, nicotinamide adenine dinucleotide; TCA, tricarboxylic acid.
Approximately 10% to 20% of ingested ethanol is oxidized through a microsomal ethanol-oxidizing system (MEOS), comprising cytochrome P450 enzymes in the endoplasmic reticulum (especially CYP2E1). CYP2E1 has a high Km for ethanol and is inducible by ethanol. Therefore, the proportion of ethanol metabolized through this route is greater at high ethanol concentrations and greater after chronic consumption of ethanol.
Acute effects of alcohol ingestion arise principally from the generation of NADH, which greatly increases the NADH/NAD+ ratio of the liver. As a consequence, fatty acid oxidation is inhibited, and ketogenesis may occur. The elevated NADH/NAD+ ratio may also cause lactic acidosis and inhibit gluconeogenesis.
Ethanol metabolism may result in alcohol-induced liver disease, including hepatic steatosis (fatty liver), alcohol-induced hepatitis, and cirrhosis. The principal toxic products of ethanol metabolism include acetaldehyde and free radicals. Acetaldehyde forms adducts with proteins and other compounds. The hydroxyethyl radical produced by the MEOS and other radicals produced during inflammation cause irreversible damage to the liver. Many other tissues are adversely affected by ethanol, acetaldehyde, or by the consequences of hepatic dysmetabolism and injury. Genetic polymorphisms in the enzymes of ethanol metabolism may be responsible for individual variations in the development of alcohol use disorder or the development of liver cirrhosis.
THE WAITING ROOM 
A dietary history for Ivan A. showed that he had continued his habit of drinking scotch and soda each evening while watching TV, but he did not add the ethanol calories to his dietary intake. He justifies this calculation on the basis of a comment he heard on a radio program that calories from alcohol ingestion “don’t count” because they are empty calories that do not cause weight gain.
Al M. was found lying semiconscious at the bottom of the stairs by his landlady when she returned from an overnight visit with friends. His face had multiple bruises and his right forearm was grotesquely angulated. Nonbloody dried vomitus stained his clothing. Al M. was rushed by ambulance to the emergency room at the nearest hospital. In addition to multiple bruises and the compound fracture of his right forearm, he had deep and rapid (Kussmaul) respirations and was moderately dehydrated.
Initial laboratory studies showed a relatively large anion gap of 34 mmol/L (reference range, 9 to 15 mmol/L). An arterial blood gas analysis (which measures pH in addition to the levels of dissolved O2 and CO2) confirmed the presence of a metabolic acidosis. Al M.’s blood alcohol level was only slightly elevated. His serum glucose was 68 mg/dL (low normal).
Jean T., a 46-year-old commercial artist, recently lost her job because of absenteeism. Her husband of 24 years had left her 10 months earlier. She complains of loss of appetite, fatigue, muscle weakness, and emotional depression. She has had occasional pain in the area of her liver, at times accompanied by nausea and vomiting.
On physical examination, the physician notes tenderness to light percussion over her liver and her abdomen is mildly distended. There is a suggestion of mild jaundice (yellow discoloration of her skin and mucus membranes). No obvious neurological or cognitive abnormalities are present.
After detecting a hint of alcohol on Jean T.’s breath, the physician questions Jean T. about her drinking. Jean T. admits that for the last 5 or 6 years, she has been drinking gin on a daily basis (~4 to 5 drinks, or 68 to 85 g ethanol) and eating infrequently. Laboratory tests showed that her serum ethanol level on the initial office visit was 245 mg/dL (0.245%); values above 200 mg/dL (0.1%) are indicative of significant intoxication.
I. Ethanol Metabolism
Ethanol is a small molecule that is both lipid and water soluble. It is therefore readily absorbed from the intestine by passive diffusion. A small percentage of ingested ethanol (0% to 5%) enters the gastric mucosal cells of the upper gastrointestinal (GI) tract (tongue, mouth, esophagus, and stomach), where it is metabolized. The remainder enters the blood. Of this, 85% to 98% is metabolized in the liver, and only 2% to 10% is excreted through the lungs or kidneys.
The major route of ethanol metabolism in the liver is through liver alcohol dehydrogenase, a cytosolic enzyme that oxidizes ethanol to acetaldehyde with reduction of NAD+ to NADH (Fig. 33.2). Alcohol dehydrogenase is a dimer of either identical or different subunits. If acetaldehyde is not removed by metabolism, it can exert toxic actions in the liver and can enter the blood and exert toxic effects in other tissues.
FIGURE 33.2 The pathway of ethanol metabolism. ADH, alcohol dehydrogenase; ALDH, acetaldehyde dehydrogenase; NAD, nicotinamide adenine dinucleotide.
Approximately 90% of the acetaldehyde that is generated is further metabolized to acetate in the liver. The major enzyme involved is a low-Km mitochondrial acetaldehyde dehydrogenase (ALDH), which oxidizes acetaldehyde to acetate with generation of NADH (see Fig. 33.2). Acetate, which has no toxic effects, may be activated to acetyl CoA in the liver (where it can enter either the TCA cycle or the pathway for fatty acid synthesis). However, most of the acetate that is generated enters the blood and is activated to acetyl CoA in skeletal muscles and other tissues (see Fig. 33.1). Acetate is generally considered nontoxic and is a normal constituent of the diet.
The other principal route of ethanol oxidation in the liver is the microsomal ethanol-oxidizing system (MEOS), which also oxidizes ethanol to acetaldehyde (Fig. 33.3). The principal microsomal enzyme involved is a cytochrome P450 mixed-function oxidase isozyme (CYP2E1), which uses NADPH as an additional electron donor and O2 as an electron acceptor. This route accounts for only 10% to 20% of ethanol oxidation in a moderate drinker.
FIGURE 33.3 The reaction catalyzed by the microsomal ethanol-oxidizing system (MEOS) (which includes CYP2E1) in the endoplasmic reticulum (ER). NADP, nicotinamide adenine dinucleotide phosphate.
Each of the enzymes involved in ethanol metabolism (alcohol dehydrogenase, acetaldehyde dehydrogenase, and CYP2E1) exists as a family of isoenzymes. Individual variations in the quantity of these isoenzymes influence a number of factors, such as the rate of ethanol clearance from the blood, the degree of inebriation exhibited by an individual, and differences in individual susceptibility to the development of alcohol-induced liver disease.
A. Alcohol Dehydrogenase
Alcohol dehydrogenase (ADH) exists as a family of isoenzymes with varying specificity for chain length of the alcohol substrate (Table 33.1). Ethanol is a small molecule that does not exhibit much in the way of unique structural characteristics and, at high concentrations, is nonspecifically metabolized by many members of the ADH family. The alcohol dehydrogenases that exhibit the highest specificity for ethanol are members of the ADH1 family. Humans have six genes for this family of alcohol dehydrogenases, each of which exists as allelic variants (polymorphisms).
TABLE 33.1 Major Isozymes of Medium-Chain-Length Alcohol Dehydrogenases
GENE | SUBUNIT | TISSUE DISTRIBUTION | PROPERTIES |
ADH1Aa ADH1B ADH1C | α β γ | Most abundant in liver and adrenal glands. Much lower levels in kidney, lung, colon, small intestine, eye, ovary, blood vessels. None in brain or heart. | Km of 0.013–4 mM for ethanol. Active only with ethanol. High tissue capacity. |
ADH4 | π | Primarily liver, stomach, lower levels in GI tract. | Km of 11 mM for ethanol. |
ADH5 | χ | Ubiquitously expressed, but at higher levels in liver. The only isozyme present in germinal cells. | Relatively inactive toward ethanol (Km = 3,400 mM). Active mainly toward long-chain alcohols, and ω-OH fatty acids. |
ADH7 | σ | Present in highest levels in upper GI tract, gingiva, and mouth, esophagus, down to the stomach. Not present in liver. | Km of 58 mM. It is the most active of medium-chain alcohol dehydrogenases toward the substrate retinal. |
GI, gastrointestinal.
aThe ADH1A, B, and C genes are classified as Class I ADH. ADH1B can also be designated as ADH2, while ADH1C can be designated as ADH3.
A gene for ADH6 has also been identified, and mRNA corresponding to ADH6 has been detected in cells, but the ADH6 protein has not been found in any ADH dimers.
The ADH1 family members are present in high quantities in the liver, representing approximately 3% of all soluble protein. These alcohol dehydrogenases, commonly referred to collectively as liver alcohol dehydrogenase, have a low Km for ethanol between 0.013 and 4 mM (high affinities). Thus, the liver is the major site of ethanol metabolism and the major site at which the toxic metabolite acetaldehyde is generated.
The human has at least seven, and possibly more, genes that code for specific isoenzymes of medium-chain-length alcohol dehydrogenases, the major enzyme responsible for the oxidation of ethanol to acetaldehyde in the human. These different alcohol dehydrogenases have an approximately 60% to 70% identity and are assumed to have arisen from a common ancestral gene similar to the ADH3 isoenzyme many millions of years ago. The ADH1 alcohol dehydrogenases (ADH1A, ADH1B, and ADH1C) are all present in high concentration in the liver, and they have relatively high affinity and capacity for ethanol at low concentrations. (These properties are quantitatively reflected by their low Km, a parameter discussed in Chapter 9.) They have a 90% to 94% sequence identity and are able to form heterodimers among themselves. However, none of the ADH1s can form dimers with an ADH subunit from any of the other ADH genes, which function as homodimers. The three genes for class I alcohol dehydrogenases are arranged in tandem, head to tail, on chromosome 4. The genes for the other classes of alcohol dehydrogenase are also on chromosome 4 in nearby locations.
B. Acetaldehyde Dehydrogenases
Acetaldehyde is oxidized to acetate, with the generation of NADH, by acetaldehyde dehydrogenases (see Fig. 33.2). More than 80% of acetaldehyde oxidation in the human liver is normally catalyzed by mitochondrial acetaldehyde dehydrogenase (ALDH2), which has a high affinity for acetaldehyde (Km of 0.2 µM) and is highly specific. However, individuals with a common allelic variant of ALDH2 (designated as ALDH2*2) have a greatly decreased capacity for acetaldehyde metabolism, due to an increased Km (46 µM) and a decreased Vmax (0.017 units/mg vs. 0.60 units/mg).
Most of the remainder of acetaldehyde oxidation occurs through a cytosolic acetaldehyde dehydrogenase (ALDH1). Additional aldehyde dehydrogenases act on a variety of organic alcohols, toxins, and pollutants.
The accumulation of acetaldehyde causes nausea and vomiting, and, therefore, inactive acetaldehyde dehydrogenases are associated with a distaste for alcoholic beverages and protection against alcoholism. In one of the common allelic variants of ALDH2 (ALDH2*2), a single substitution increases the Km for acetaldehyde 230-fold (lowers the affinity) and decreases the Vmax 35-fold, resulting in a very inactive enzyme. Homozygosity for the ALDH2*2 allele affords absolute protection against alcoholism; no individual with this genotype has been found among individuals with alcohol use disorder. Patients with alcohol use disorder are sometimes treated with acetaldehyde dehydrogenase inhibitors (e.g., disulfiram) to help them abstain from alcohol intake. Unfortunately, patients who continue to drink while taking this drug are exposed to the toxic effects of elevated acetaldehyde levels, so it can only be used in patients who are not drinking.
C. Fate of Acetate
Metabolism of acetate requires activation to acetyl CoA by acetyl CoA synthetase in a reaction similar to that catalyzed by fatty acyl CoA synthetases (Fig. 33.4). In liver, the principal isoform of acetyl CoA synthetase (ACSI) is a cytosolic enzyme that generates acetyl CoA for the cytosolic pathways of cholesterol and fatty acid synthesis. Acetate entry into these pathways is under regulatory control by mechanisms involving cholesterol or insulin. Thus, most of the acetate generated enters the blood.
FIGURE 33.4 The activation of acetate to acetyl coenzyme A (acetyl CoA). AMP, adenosine monophosphate; ATP, adenosine triphosphate; CoA, coenzyme A; PPi, pyrophosphate.
Acetate is taken up and oxidized by other tissues, notably heart and skeletal muscle, which have a high concentration of the mitochondrial acetyl CoA synthetase isoform (ACSII). This enzyme is present in the mitochondrial matrix. It, therefore, generates acetyl CoA that can enter the TCA cycle directly and be oxidized to CO2.
D. The Microsomal Ethanol-Oxidizing System
Ethanol is also oxidized to acetaldehyde in the liver by the microsomal ethanol-oxidizing system, which comprises members of the cytochrome P450 superfamily of enzymes. Ethanol and NADPH both donate electrons in the reaction, which reduces O2 to H2O (Fig. 33.5). The cytochrome P450 enzymes all have two major catalytic protein components: an electron-donating reductase system that transfers electrons from NADPH (cytochrome P450 reductase) and a cytochrome P450. The cytochrome P450 protein contains the binding sites for O2 and the substrate (e.g., ethanol) and carries out the reaction. The enzymes are present in the endoplasmic reticulum, which on isolation from disrupted cells forms a membrane fraction after centrifugation that was formerly called “microsomes” by biochemists.
FIGURE 33.5 General structure of cytochrome P450 enzymes. O2 binds to the P450 Fe-heme in the active site and is activated to a reactive form by accepting electrons (e−). The electrons are donated by the cytochrome P450 reductase, which contains a flavin adenine dinucleotide (FAD) plus a flavin adenine mononucleotide (FMN) or Fe–S center to facilitate the transfer of single electrons from NADPH to O2. The P450 enzymes involved in steroidogenesis have a somewhat different structure. For CYP2E1, RH is ethanol (CH3CH2OH) and ROH is acetaldehyde (CH3CHO). NADP, nicotinamide adenine dinucleotide phosphate.
1. CYP2E1
The MEOS is part of the superfamily of cytochrome P450 enzymes, all of which catalyze similar oxidative reactions. Within the superfamily, at least 10 distinct gene families are found in mammals. More than 100 different cytochrome P450 isozymes exist within these 10 gene families. Each isoenzyme has a distinct classification according to its structural relationship with other isoenzymes. The isoenzyme that has the highest activity toward ethanol is called CYP2E1. CYP represents cytochrome P450. In CYP2E1, the “2” refers to the gene family (isozymes with >40% amino acid sequence identity), the “E” to the subfamily (isozymes with >55% sequence identity), and the “1” refers to the individual enzymes within this subfamily. A great deal of overlapping specificity exists among the various P450 isoenzymes, and ethanol is also oxidized by several other P450 isoenzymes. “MEOS” refers to the combined ethanol-oxidizing activity of all the P450 enzymes.
CYP2E1 has a much higher Km for ethanol than the ADH1 family members (11 mM [51 mg/dL], compared with 0.013 to 4 mM [0.06 to 18 mg/dL]). Thus, a greater proportion of ingested ethanol is metabolized through CYP2E1 at high levels of ethanol consumption than at low levels.
2. Induction of P450 Enzymes
The P450 enzymes are inducible both by their most specific substrate and by substrates for some of the other cytochrome P450 enzymes. Chronic consumption of ethanol increases hepatic CYP2E1 levels approximately 5- to 10-fold. However, it also causes a 2- to 4-fold increase in some of the other P450s from the same subfamily, from different subfamilies, and even from different gene families. The endoplasmic reticulum undergoes proliferation, with a general increase in the content of microsomal enzymes, including those that are not involved directly in ethanol metabolism.
The increase in CYP2E1 with ethanol consumption occurs through transcriptional, posttranscriptional, and posttranslational regulation. Increased levels of mRNA, resulting from induction of gene transcription or stabilization of message, are found in actively drinking patients. The protein is also stabilized against degradation. In general, the mechanism for induction of P450 enzymes by their substrates occurs through the binding of the substrate (or related compound) to an intracellular receptor protein, followed by binding of the activated receptor to a response element in the target gene. Ethanol induction of CYP2E1 appears to act via stabilization of the protein and protection against degradation (an increased half-life for the synthesized protein).
Although induction of CYP2E1 increases ethanol clearance from the blood, it has negative consequences. Acetaldehyde may be produced faster than it can be metabolized by acetaldehyde dehydrogenases, thereby increasing the risk of hepatic injury. An increased amount of acetaldehyde can enter the blood and can damage other tissues. In addition, cytochrome P450 enzymes are capable of generating free radicals, which also may lead to increased hepatic injury and cirrhosis (see Chapter 25).
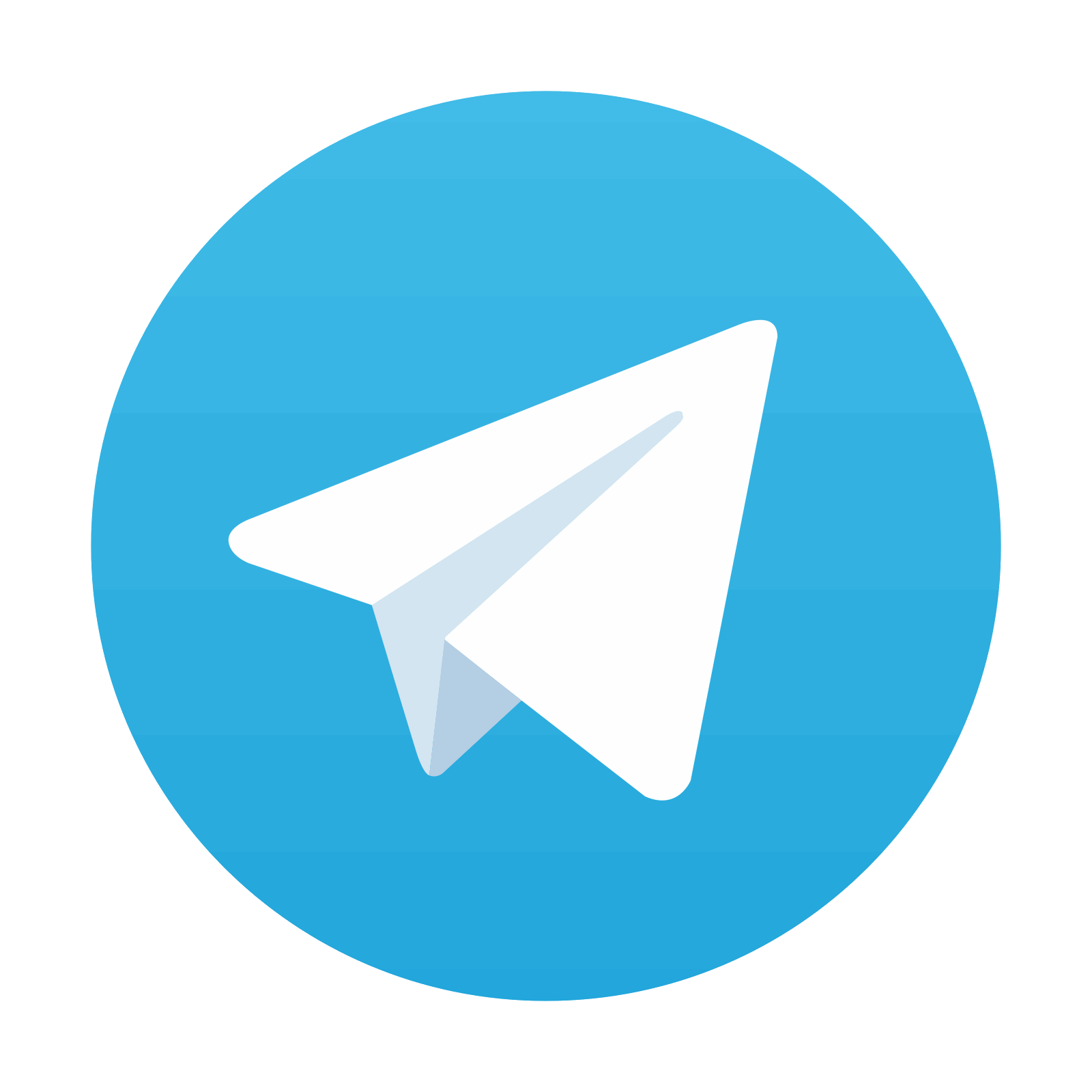
Stay updated, free articles. Join our Telegram channel

Full access? Get Clinical Tree
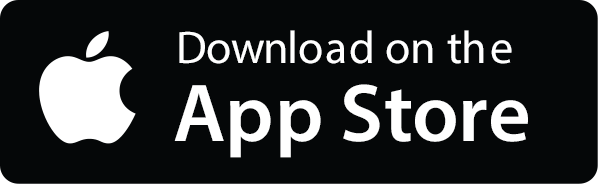
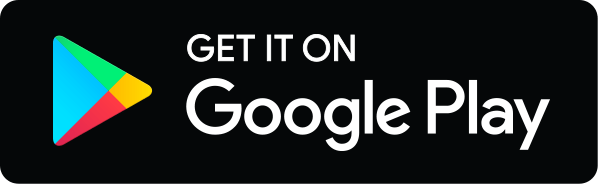