Chapter 43
Structure and Function of the Musculoskeletal System
Christy L. Crowther-Radulewicz
Structure and Function of Bones
Bone marrow is one of the sources of mesenchymal stem cells (MSCs) (Figure 43-1). These nonhematopoietic stem cells consist of a small proportion of the stromal cell population in the bone marrow, and can generate bone, cartilage, fat, cells that support the formation of blood, and fibrous connective tissue. Within certain bones, the marrow cavities also serve as storage site for the hematopoietic stem cells that form both blood and immune cells. In adults, blood cells originate exclusively in the marrow cavities of the skull, vertebrae, ribs, sternum, scapulae, and pelvis. Bones also have a crucial role in mineral homeostasis, and storing and releasing minerals (i.e., calcium, phosphate, carbonate, magnesium) that are essential for the proper working of many delicate cellular mechanisms.
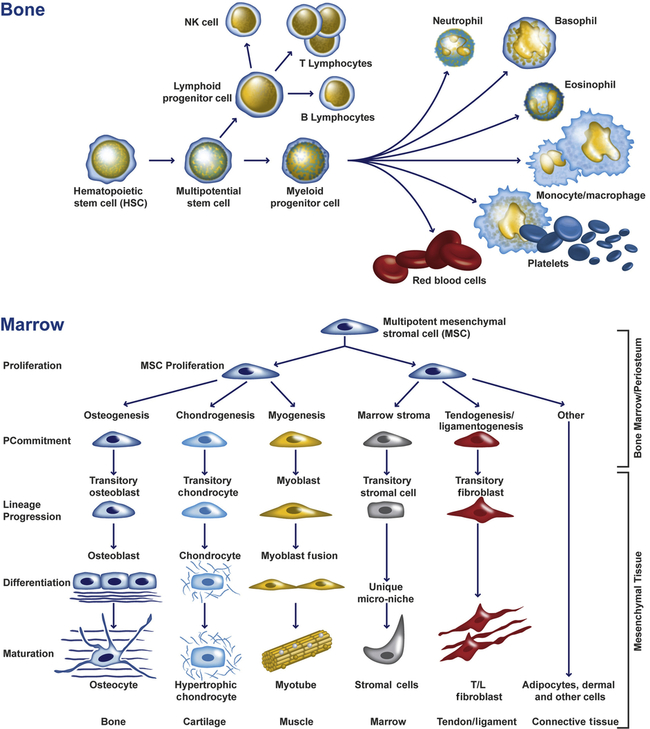
Undifferentiated hematopoietic and mesenchymal multipotential cells give rise to a variety of cell types with distinct functions. (Adapted from abcam.com.)
Elements of Bone Tissue
Mature bone is a rigid yet flexible connective tissue consisting of cells, fibers, a gelatinous material termed ground substance, and large amounts of crystallized minerals, mainly calcium, that give bone its rigidity. The structural elements of bone are summarized in Table 43-1.
TABLE 43-1
STRUCTURAL ELEMENT | FUNCTION |
Bone Cells | |
Osteoblasts | Synthesize collagen and proteoglycans; stimulate bone formation and are also involved in some osteoclast resorptive activity |
Osteocytes | Maintain bone matrix; act as mechanoreceptors; influence osteoblasts and osteoclasts |
Osteoclasts | Resorb bone; major role in mineral homeostasis |
Bone Matrix | |
Collagen fibers | Lend support and tensile strength |
Proteoglycans | Control transport of ionized materials through matrix |
Bone morphogenic proteins (BMPs)∗ | Induce cartilage, bone, tendon, and ligament formation and repair |
Glycoproteins | |
Sialoprotein | Promotes calcification |
Osteocalcin | Inhibits calcium-phosphate precipitation; promotes bone resorption |
Laminin | Stabilizes basement membranes in bones |
Osteonectin | Binds calcium in bones |
Albumin | Transports essential elements to matrix; maintains osmotic pressure of bone fluid |
α-Glycoprotein | Promotes calcification |
Minerals (Elements) | Crystallizes to lend rigidity and compressive strength |
Calcium | Regulates vitamin D and thereby promotes mineralization |
Phosphate | Balance of organic and inorganic phosphate required for proper bone mineralization; regulates vitamin D |
Alkaline phosphatase | Promotes mineralization |
Vitamins | |
Vitamin D | Assists with differentiation, mineralization of osteoblasts |
Vitamin K | Increases bone calcification; reduces serum osteocalcin |
∗See Table 43-2 for BMP functions.
Endochondral ossification occurs when mesenchymal (mesenchyme, or loose tissue found during embryonic development) stem cells begin differentiating into chondrocytes (see Figure 43-1), which in turn develop a mineralized cartilage scaffold that allows formation of osteoblasts. Most bone elements are formed this way. With the second mechanism, intramembranous bone formation, mesenchymal stem cells differentiate into a preosteoblast line that then forms osteoblasts without any cartilage framework.1,2
Multiple factors influence normal bone formation, maintenance, and remodeling. The superfamily of signaling factors, containing more than 40 members including bone morphogenic proteins (BMPs), is known as the transforming growth factor-beta (TGF-β) family. This group is primarily responsible for initiation, differentiation, and commitment of precursor cells into osteoblasts. TGF-β signals are transmitted across the plasma membrane, combine with certain proteins that act as transcription factors (Smads), and then form specific receptors known as R-Smads. These receptors, in turn, initiate intracellular signaling, interact with other transcription factors, and regulate other factors that are important in osteoblast formation, function, and maintenance.3 Crosstalk between signaling pathways (including TGF-β, BMPs, FGF, Wnt, Notch, MAPK, and Hedgehog) is critical in regulating osteoblasts.
BMPs have multiple crucial functions in the skeletal system. BMP activities are regulated at different molecular levels. Table 43-2 summarizes the role of several important BMPs.
TABLE 43-2
BONE MORPHOGENIC PROTEINS (BMPs) AND THEIR KNOWN FUNCTIONS
BMP | KNOWN FUNCTION |
BMP1 | Cartilage development; is actually a metalloprotease; key role in extracellular matrix (ECM) formation |
BMP2 | Induces bone and cartilage formation, osteoblast differentiation, bone healing |
BMP3 (osteogenin) | Induces bone formation |
BMP4 | Regulates formation of teeth, limbs, and bone |
BMP5 | Involved in cartilage development |
BMP6 | Found in osteoblasts; helps maintain adult joint integrity; accelerates bone repair |
BMP7 | Major role in osteoblast differentiation, chondrocyte formation, fracture healing; important in renal development and repair |
BMP8a | Bone and cartilage development; up-regulated in fracture nonunion |
BMP9 | Induces osteogenesis in mature osteoblasts |
BMP10 | Plays role in development of the heart |
BMP12 (cartilage-derived morphogenic protein-3; CDMP-3) | Tendon and ligament formation |
BMP13 | Cartilage development; tendon and ligament repair |
BMP14 | Assists in bone and tendon healing; cartilage formation |
Data from Caetano-Lopes J, Canhão H, Fonseca JE: Arthritis Res Ther 9(Suppl 1):S1, 2007; Hojo H et al: J Bone Miner Metab 28(5):489–502, 2010; Fajardo M, Liu C-J, Egol K: Clin Orthop Relat Res 467(12):3071–3078, 2009; Li Y et al: Zhong Nan Da Xue Xue Bao Yi Xue Ban 37(1):1–5, 2012.
Wnt genes belong to a large family of protein-signaling factors that are required for the development of body systems, including the musculoskeletal system. They play a significant role in forming bone, developing bone mass, remodeling, and fracture healing. Wnt signaling regulates production and differentiation of osteoblasts and osteoclasts, and affects bone mass and density, joint formation, fracture repair, bone remodeling, and some bone diseases. Other important elements responsible for bone formation and homeostasis are presented in Table 43-3.
TABLE 43-3
SELECTED FACTORS AFFECTING BONE FORMATION AND HOMEOSTASIS
FACTOR | FUNCTION |
Transforming growth factor-beta (TGF-β) | Superfamily of polypeptides; regulates bone formation, many other cellular processes though signaling |
Platelet-derived growth factor (PDGF) | Increases number of osteoblasts |
Fibroblast growth factor-2 (FGF) | FGF-2 increases osteoblast population, but not function; inhibits alkaline phosphatase activity, osteocalcin, type 1 collagen, and osteopontin |
Insulin-like growth factor (IGF) | |
IGF-1 | Increases peak bone mass during adolescence; decreases osteoblast apoptosis; maintains bone matrix |
IGF-2 | Increases BMP-9–induced endochondral ossification |
Smad proteins | Mediate the signaling cascade of transforming growth factor-beta (TGF-β), especially in embryonic bone development; play a role in crosstalk between BMP/TGF-β and Wnt signaling pathways |
Bone morphogenic proteins (BMPs) | Members of transforming growth factor-beta (TGF-β) superfamily of polypeptides; have many functions outside skeletal system; stimulate endochondral bone and cartilage formation and function, promote osteoblast maturation; augment bone remodeling by affecting both osteoblasts and osteoclasts |
Tumor necrosis factors (TNFs) | Superfamily of cytokines; play major role in regulating bone metabolism, especially osteoclast function |
Osteoprotegerin (OPG) | Inhibits bone remodeling/resorption; produced by several cells, including osteoblasts; is a decoy receptor for RANKL (binds to RANKL, inhibiting RANK/RANKL interactions, suppressing osteoclast formation and bone resorption) |
Receptor activator of nuclear factor κβ (RANK) | Stimulates differentiation of osteoclast precursors; activates mature osteoclasts |
Bone morphogenic protein antagonists | Prevent BMP signaling |
Noggin | Binds BMP-2 and BMP-4, reducing osteoblast function |
Gremlin | Multiple effects in and out of skeletal system, but also binds BMP-2, -4, and -7; may play a role in development of osteoporosis |
Twisted gastrulation | Acts as either a BMP agonist or an antagonist |
Activin (a BMP-related protein) | Affects both osteoblasts and osteoclasts; may promote bone formation and fracture healing; expressed by both osteoblasts and chondrocytes; helps regulate bone mass |
Inhibin | Dominant over activin and BMPs; helps regulate bone mass and strength by affecting formation of osteoblasts and osteoclasts |
Leptin | Plays role in bone formation and resorption |
Wnt (a signaling pathway) | Important in differentiating osteoblasts and bone formation; has overlapping effects with BMPs; helps regulate bone formation and remodeling |
Wnt antagonists | |
Dickkopf family (Dkk) | Disrupt Wnt signaling, leading to reduced bone mass |
Sclerostin | Protein secreted by osteocytes, osteoblasts, and osteoclasts; binds to BMP-6 and BMP-7; interferes with Wnt signaling pathway, inhibiting bone formation by osteoblasts |
Transcription Factors | |
Nuclear factor of activated κβ cells (NF-κβ) | Affects embryonic osteoclastogenesis; plays a role in certain osteoclast, osteoblast, and chondroblast functions |
Matrix metalloproteinases (MMPs) | |
Family of endopeptidases (enzymes) that includes collagenases, gelatinases, stromelysins, matrilysins | Help maintain equilibrium of extracellular matrix (ECM); break down almost all components of ECM |
A disintegrin and metalloproteinase (ADAM) | Proteolytic enzymes; also have cell-signaling functions, usually linked to cell membrane |
A disintegrin and metalloproteinase with thrombospondin motifs (ADAMTS) | Similar to ADAMs but are secreted into circulation, are found around cells; various subgroups affect multiple tissues |
Cysteine Protease | |
Cathepsin | K-expressed by osteoclasts; assists in bone remodeling by cleaving proteins, such as collagen type I, collagen type II, and osteonectin |
MMP Inhibitors | |
Tetracyclines (especially doxycycline), bisphosphonates | Block enzymatic function of MMPs |
Tissue inhibitors of metalloproteinases (TIMPs) | Balance effect of MMPs in maintaining ECM equilibrium |
From Boyce GC, Zhenqiang, Zing L: Ann N Y Acad Sci 1192:367–375, 2010; Canalis E: J Cell Biol 108(4):769–777, 2009; Kim Y-S et al: J Korean Med Sci 25:985–991, 2010; Moester MJ et al: Calcif Tissue Int 87(2):99–107, 2010; Nicks KN et al: Mol Cell Endocrinol 310(1-2):11–20, 2009; Pasternak B, Aspenberg P: Acta Orthopaedica 80(6):693–703, 2009; Stewart A, Guan H, Yang K: J Cell Physiol 223(3):658–666, 2010; Tat SK et al: Keio Med J 58(1):29–40, 2009.
In mature bone the formation of new tissue begins with the production of an organic matrix by the bone cells. This bone matrix consists of ground substances, collagen, and other proteins (see Table 43-1) that take part in bone formation and maintenance.
Bone Cells
Bone contains three types of cells: osteoblasts, osteocytes, and osteoclasts (Figure 43-2). Osteoblasts are the bone-forming cells, whose primary function is to lay down new bone. Once this function is complete, osteoblasts become osteocytes that are imbedded in bone. Comprising 90% to 95% of bone cells, osteocytes develop dendritic processes that extend to either the bone surface or the bone’s vascular space.4 They help maintain bone by signaling osteoblasts and osteoclasts to form and resorb bone.5 Osteoclasts are the major bone-resorbing cells responsible for remodeling.
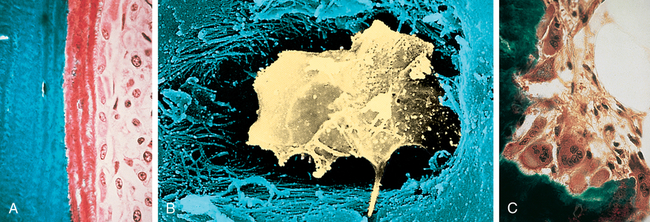
A, Osteoblasts are responsible for the production of collagenous and noncollagenous proteins that compose osteoid. Active osteoblasts are lined up on the osteoid. Note the eccentrically located nuclei. B, Osteocyte. Scanning electron micrograph showing an osteocyte within a lacuna. The cell is surrounded by collagen fibers and mineralized bone. C, Osteoclasts actively resorb mineralized tissue. The scalloped surface in which the multinucleated osteoclasts rest is termed the Howship lacuna. (A and C from Damjanov I, Linder J, editors: Anderson’s pathology, ed 10, St Louis, 1996, Mosby. B from Erlandsen S, Magney J: Color atlas of histology, St Louis, 1992, Mosby.)
Osteoblasts
Osteoblasts are cells derived from mesenchymal stem cells. Osteoblasts produce several substances, including osteocalcin, TGF-β, macrophage colony-stimulating factor, receptor activator of nuclear factor κβ ligand (RANKL), osteoprotegerin (OPG), and bone matrix6 and osteocalcin when stimulated by 1,25-dihydroxy-vitamin D. Osteoblasts are active on the outer surface of bones, where they form a single layer of cells. They initiate the formation of new bone by their synthesis of osteoid (nonmineralized bone matrix). The mechanism of osteoblast stimulation is reported to be the production of so-called coupling factors generated during the resorption process. Osteoblasts cause the formation of new bone and the orderly mineralization of bone matrix by concentrating some of the plasma proteins (growth factors) found in the bone matrix and by facilitating the deposit and exchange of calcium and other ions at the site. Osteoblasts alter levels of RANKL and OPG; the balance of these two cytokines determines overall osteoclast formation7 (see Figure 44-11). As new bone is formed, it is shaped and remodeled through TGF-β, as well as other plasma proteins (growth factors) found in the bone marrow (Table 43-4).
TABLE 43-4
EFFECTS OF SELECTED CYTOKINES (GROWTH FACTORS) ON SKELETAL TISSUES
CYTOKINE (GROWTH FACTOR) | TARGET TISSUE | FORMATION | RESORPTION |
Transforming growth factor-beta | Bone Cartilage | +, − +, − | +, − − |
Transforming growth factor-alpha or epidermal growth factor | Bone Cartilage | +, − + | + 0 |
Insulin-like growth factor | Bone Cartilage | − − | ? 0 |
Fibroblast growth factor | Bone Cartilage | +, − − | ? 0 |
Platelet-derived growth factor | Bone Cartilage | 0 | + |
Colony-stimulating factors | Bone Cartilage | ? − | ? − |
Interferon-gamma | Bone Cartilage | − − | ? + |
Tumor necrosis factor | Bone Cartilage | − +, − | + + |
Interleukins 1, 3, and 6 | Bone Cartilage | − | + |
Osteocytes
An osteocyte is a transformed osteoblast that is trapped or surrounded in osteoid as it hardens from minerals that enter during ossification (see Figure 43-2, B). It is the final differentiation stage for an osteoblast. The osteocyte is within a space in the hardened bone matrix called a lacuna. Each osteocyte has a high nucleus/cytoplasm ratio with a thin layer of nonmineralized osteoid around it, similar to the egg white surrounding an egg yolk.
Osteocytes communicate with other bone cells and instruct both osteoblasts and osteoclasts about when and where to form and resorb bone; concentrate nutrients in the matrix; regulate bone mass and minerals (especially calcium and phosphorus); and secrete other proteins, such as sclerostin and fibroblast growth factor-23 (FGF-23), that influence mineral metabolism.3,4,8,9 Sclerostin inhibits osteoblasts, thus reducing bone formation. Additionally, osteocytes seem to function as endocrine receptors in regulating bone metabolism and are prime target cells of parathyroid hormone (PTH).9–11 Through exchanges between these cells, hormone catalysts, and minerals, optimal levels of calcium, phosphorus, and other minerals are maintained in blood plasma. The osteocyte also aids in modifying bone matrix through release of enzymes to dissolve the mineralized walls of the lacunae to prepare the bone for remodeling. (Remodeling is described on p. 1519.)
Osteoclasts
Osteoclasts are large, multinucleated bone cells derived from hematopoietic stem cells in the bone marrow stroma and are the major resorptive cells of bone. Considered to be the major remodelers of bone, osteoclasts contain lysosomes (digestive vacuoles) filled with hydrolytic enzymes. Fine projections, or microvilli, fan out from the osteoclast cell’s surface and are known as ruffled borders; these projections result from extensive infoldings of the cell membrane adjacent to the resorptive surface.12 Osteoclasts in regions of bone resorption lie in pits called Howship lacunae, where the infolded, ruffled borders of the osteoclasts greatly increase the surface area of the plasma membrane. The infolds end in numerous channels and vesicles in the cell cytoplasm, permitting them to resorb the bone under their ruffled, infolded borders.
Osteoclasts bind to the bone surface of cell attachment proteins called integrins.12 In contact with bone mineral, osteoclasts use adhesive structures, called podosomes, to attach themselves to the bone surface. They then seal their cytoplasm with the underlying bone and dissolve the bone and matrix while protecting the surrounding tissue.13 They effect resorption of bone by secretion of hydrochloric acid (HCl) and cathepsin K (a protease enzyme), which help dissolve bone minerals and collagenase, thereby aiding the digestion of collagen along with the action of cytokines (see Table 43-4). Matrix metalloproteinases (MMPs), a group of proteolytic enzymes, help control osteoclast-matrix interactions necessary for bone resorption. Once resorption is completed, the osteoclast disappears by degeneration, either by reverting to its parent cell or by leaving the site through the process of cell mobility, wherein the osteoclast then becomes an inactive, or “resting,” osteoclast. Overall, bone homeostasis is the result of crosstalk between osteoclasts, osteoblasts, and osteocytes.14 Recent evidence has indicated that osteoclasts also may play a role in the body’s immune system.15–17
Bone Matrix
Bone matrix is made of the extracellular elements of bone tissue, composed of about 35% organic and 65% inorganic materials. The major organic components are collagen fibers, and the major inorganic components are the calcium and phosphate minerals. Other parts of the bone matrix are the proteins, carbohydrate-protein complexes, and ground substances. Water comprises 5% to 8% of the matrix. Within the matrix are small particles known as matrix vesicles that “bud” from chondrocytes, osteoblasts, and odontoblasts. These vesicles are the initial sites for calcification in skeletal tissues.18 Calcification begins as extracellular calcium enters the matrix vesicles and forms hydroxyapatite crystals.
Collagen Fibers
Collagen fibers are the major organic component of bone matrix. The fibers are approximately 90% type I collagen, are essential for bone strength, and are synthesized and secreted by osteoblasts. Once secreted, collagen molecules assemble into thin chains called α-chains, which combine in groups of 3 to form fibrils. The fibrils form a staggered pattern, overlapping nearby fibrils by approximately one fourth their length. This staggered, overlapping pattern creates regular gaps, called hole zones, into which mineral crystals are deposited.18 After mineral deposition, the fibrils link together and twist to form ropelike fibers. Collagen fibers then join to form a framework that gives bone its tensile strength and enables it to bear weight.
Collagen is the most abundant macromolecule in the body, accounting for approximately one third of all protein and providing the structural framework for nearly all tissues. Collagen is one of the extracellular components, along with proteoglycans and noncollagenous matrix proteins, of articular cartilage. To date, more than 20 types of collagen have been identified, though all their functions are not yet known. Cartilage-specific collagens include types II (the principal component), VI, IX, X, and XI. Type IX collagen is thought to be the “glue” that holds together the type II collagen scaffold of articular cartilage, helps maintain the structural integrity of cartilage, and resists tensile forces on the joint cartilage. Type XI regulates the fibril diameter of type II cartilage. Degradation of type IX collagen by proteolytic enzymes has been seen in the early stages of osteoarthritis and rheumatoid arthritis. Researchers have proposed that this degradation, or “unplugging,” may be the mechanism for the degenerative changes seen in osteoarthritic and rheumatoid cartilage. Table 43-5 gives the musculoskeletal distribution of other types of collagen.
TABLE 43-5
TYPES OF COLLAGEN IN MUSCULOSKELETAL TISSUES
TYPE OF COLLAGEN | DISTRIBUTION IN MUSCULOSKELETAL TISSUES |
I | Bone, tendon, ligament, intervertebral disk, muscle∗ |
II | Cartilage, intervertebral disk |
III | Skin, muscle, often with type I |
IV | Basement cell membrane, muscle |
V | Codistributed with type I muscle, most interstitial tissues |
VI | Ubiquitous, muscle |
IX | Codistributed with type II muscle |
X | Cartilage growth plate |
XI | Cartilage, muscle |
XII | Codistributed with types I and III muscle |
XIII | Molecule has not been isolated in connective tissues to date |
XIV | Codistributed with type I muscle |
XV | Muscle; contains heparin sulfate proteoglycans (HSPGs) |
XVII | Muscle; contains HSPGs |
Glycoproteins
Some of the glycoproteins in bone matrix include bone sialoprotein, osteocalcin, osteonectin, laminin, albumin, and α-glycoprotein. Other proteins found in bone matrix are the compounds currently called bone morphogenic proteins (BMPs) (see Table 43-2). Sialoprotein (osteopontin) comprises about 8% of the noncollagenous matrix of bone and easily binds with calcium.
Bone Minerals
Mineralization is the final step in bone formation, after collagen synthesis and fiber formation. Mineralization has two distinct phases: (1) formation of the initial mineral deposit (initiation), and (2) proliferation or accretion of additional mineral crystals on the initial mineral deposits (growth).12 The majority of the mineral content in the body is an analog of the naturally occurring mineral hydroxyapatite.
Table 43-6 lists the sequence in which calcium and phosphate form amorphous (fluid) calcium phosphate compounds that are converted, in stages, to solid hexagonal crystals of hydroxyapatite (HAP). As the calcium and phosphorous concentrations increase in the bone matrix, the first precipitate to form is dicalcium phosphate dihydrate (DCPD). Once DCPD precipitation begins, the remaining phases of bone crystal formation proceed until insoluble HAP is produced, with approximately 80% to 90% of the HAP being incorporated into the collagen fibers. Amorphous calcium phosphate is distributed throughout the bone matrix.
TABLE 43-6
SEQUENCE OF CALCIUM AND PHOSPHATE COMPOUND FORMATION AND CRYSTALLIZATION
FORMULA | NAME | ABBREVIATION |
Ca(HPO4) × 2H2O | Dicalcium phosphate dehydrate | DCPD |
Ca4H(PO4)3 | Octacalcium phosphate | OCP |
Ca9(PO4)6 (var.) | Amorphous calcium phosphate | ACP |
Ca3(PO4)2 | Tricalcium phosphate | TCP |
Ca5(PO4)3OH | Hydroxyapatite | HAP |
Note: Compounds are listed in order in which precipitation and crystal formation occur.
Types of Bone Tissue
Bone consists of two types of bony (osseous) tissue: compact bone (cortical bone) and spongy bone (cancellous bone) (Figure 43-3). Compact bone constitutes approximately 85% of the skeleton; spongy bone comprises the remaining 15%. Both types of bone tissue contain the same structural elements, and, with a few exceptions, both compact tissue and spongy tissue are present in every bone. The major difference between the two types of tissue is the organization of the elements.
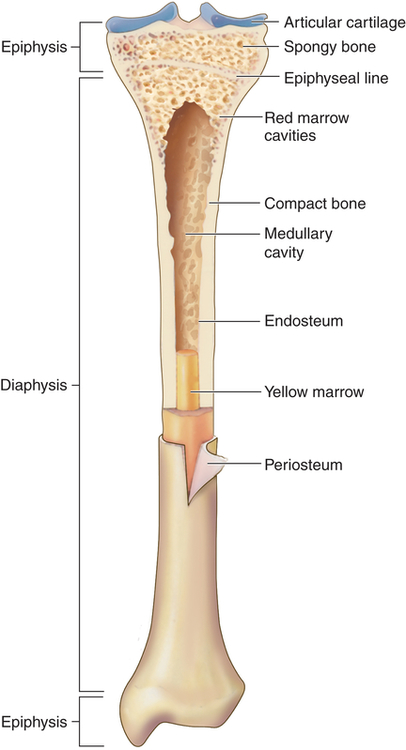
Longitudinal section of long bone (tibia) showing cancellous and compact bone. (From Patton KT, Thibodeau GA: Anatomy & physiology, ed 8, St Louis, 2013, Mosby.)
Compact bone is highly organized, solid, and extremely strong. The basic structural unit in compact bone is the haversian system (Figure 43-4). Each haversian system is made up of the following:
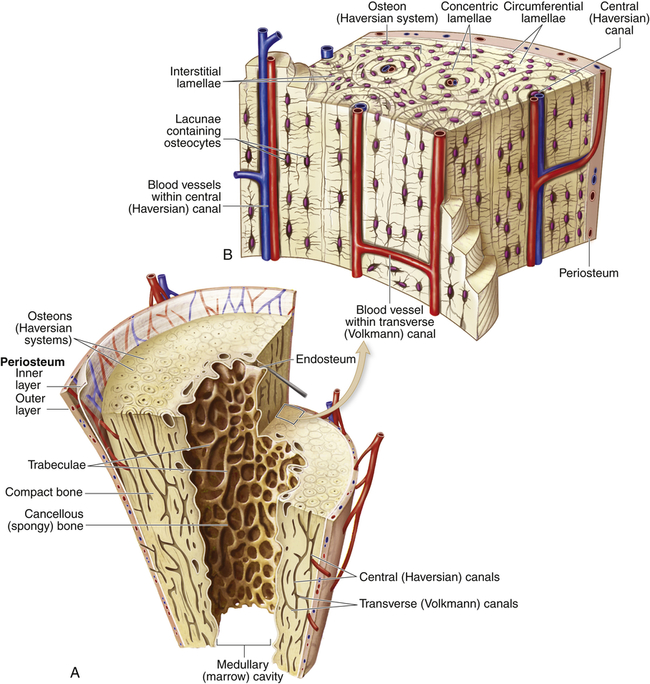
A, Longitudinal section of a long bone showing cancellous and compact bone. B, A magnified view of compact bone. (From Patton KT, Thibodeau GA: Anatomy & physiology, ed 8, St Louis, 2013, Mosby.)
1. A central canal called the haversian canal
2. Concentric layers of bone matrix called lamellae
3. Tiny spaces (lacunae) between the lamellae
4. Bone cells (osteocytes) within the lacunae
All bones are covered with a double-layered connective tissue called the periosteum. The outer layer of the periosteum contains blood vessels and nerves, some of which penetrate to the inner structures of the bone through channels called Volkmann canals (see Figure 43-4). The inner layer of the periosteum is anchored to the bone by collagenous fibers (Sharpey fibers) that penetrate the bone. Sharpey fibers also help hold or attach tendons and ligaments to the periosteum of bones.12
Characteristics of Bone
The human skeleton consists of 206 bones that constitute the axial skeleton and the appendicular skeleton (Figure 43-5). The axial skeleton consists of 80 bones that make up the skull, vertebral column, and thorax. The appendicular skeleton consists of 126 bones that make up the upper and lower extremities, the shoulder girdle (pectoral girdle), and the pelvic girdle. The skeleton contributes about 14% of the weight of the adult body.
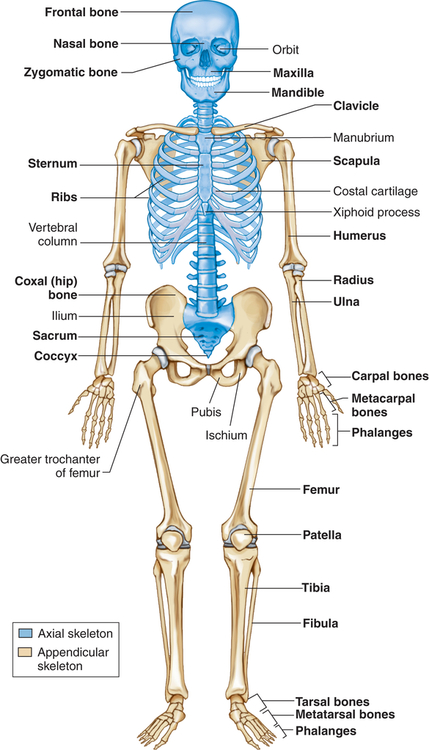
Bones can be classified by shape as long, flat, short (cuboidal), or irregular. Long bones are longer than they are wide and consist of a narrow tubular midportion (diaphysis) that merges into a broader neck (metaphysis) and a broad end (epiphysis) (see Figure 43-3).
The diaphysis consists of a shaft of thick, rigid compact bone that can tolerate bending forces. Contained within the diaphysis is the elongated marrow (medullary) cavity. The marrow cavity of the diaphysis contains primarily fatty tissue, which is referred to as yellow marrow. The yellow marrow assists red bone marrow in hematopoiesis only during times of stress. The yellow marrow cavity of the diaphysis is continuous with marrow cavities in the spongy bone of the metaphysis and diaphysis. The marrow contained within the epiphysis is red because it contains primarily blood-forming tissue (see Chapter 27). A layer of connective tissue, the endosteum, lines the outer surfaces of both types of marrow cavity.
Maintenance of Bone Integrity
Remodeling
Repair
The remodeling process can repair microscopic bone injuries, but gross injuries, such as fractures and surgical wounds (osteotomies), heal by the same stages as soft tissue injuries, except that new bone, instead of scar tissue, is the final result (see Chapter 7). In bone the stages of wound healing are as follows:
1. Hematoma formation: This process occurs if vessels have been damaged, causing hemorrhage. Fibrin and platelets within the hematoma form a meshwork that is the initial framework for healing with the help of hematopoietic growth factors such as platelet-derived growth factor and TGF-β (see Table 43-3).
2. Procallus formation: Fibroblasts, capillary buds, and osteoblasts move into the wound to produce granulation tissue called procallus. Cartilage is formed as a precursor of bone, and types I, II, and III collagen are formed. Enzymes and growth factors, such as insulin and insulin-like growth factors, plus bone morphogenic protein and osteogenin, aid in this stage of healing.
3. Callus formation: Osteoblasts in the procallus form membranous or woven bone (callus). Enzymes increase the phosphate content and permit the phosphate to join with calcium to be deposited as mineral to harden the callus.
4. Callus replacement: Osteoblasts continue to replace the callus with lamellar bone or trabecular bone (Figure 43-6).
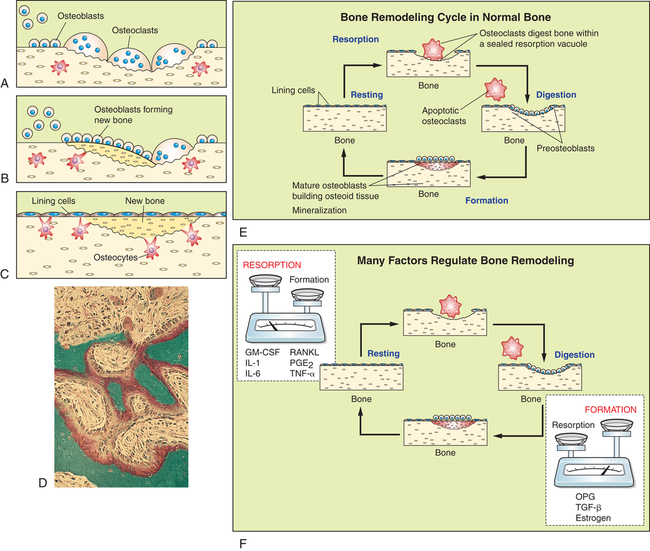
All bone cells participate in bone remodeling. In the remodeling sequence bone sections are removed by bone-resorbing cells (osteoclasts) and replaced with a new section laid down by bone-forming cells (osteoblasts). Bone remodeling is necessary because it allows the skeleton to respond to mechanical loading, maintains quality control (repair and prevent microdamage), and allows the skeleton to release growth factors and minerals (calcium and phosphate) stored in bone matrix to the circulation. The cells work in response to signals generated in the environment (see F). Only the osteoclastic cells mediate the first phase of remodeling. They are activated, scoop out bone (A), and resorb it; then the work of the osteoblasts begins (B). They form new bone that replaces bone removed by the resorption process (C). The sequence takes 4 to 6 months. D, Micrograph of active bone remodeling seen in the settings of primary or secondary hyperparathyroidism. Note the active osteoblasts surmounted on red-stained osteoid. Marrow fibrosis is present. E, Bone remodeling cycle in normal bone (F). Numerous signaling factors are necessary for remodeling. Factors most important for resorption include granulocyte macrophage-colony-stimulating factor (GM-CSF), interleukins-1 and -6 (IL-1 and IL-6), receptor activator for nuclear factor κβ ligand (RANKL), prostaglandin E2 (PGE2), and tumor necrosis factor-alpha (TNF-α). Important factors for bone formation include osteoprotegerin (OPG), transforming growth factor-beta (TGF-β), and estrogen. (Adapted from Nucleus Medical Art. D from Damjananov I, Linder J, editors: Anderson’s pathology, ed 10, St Louis, 1996, Mosby.)
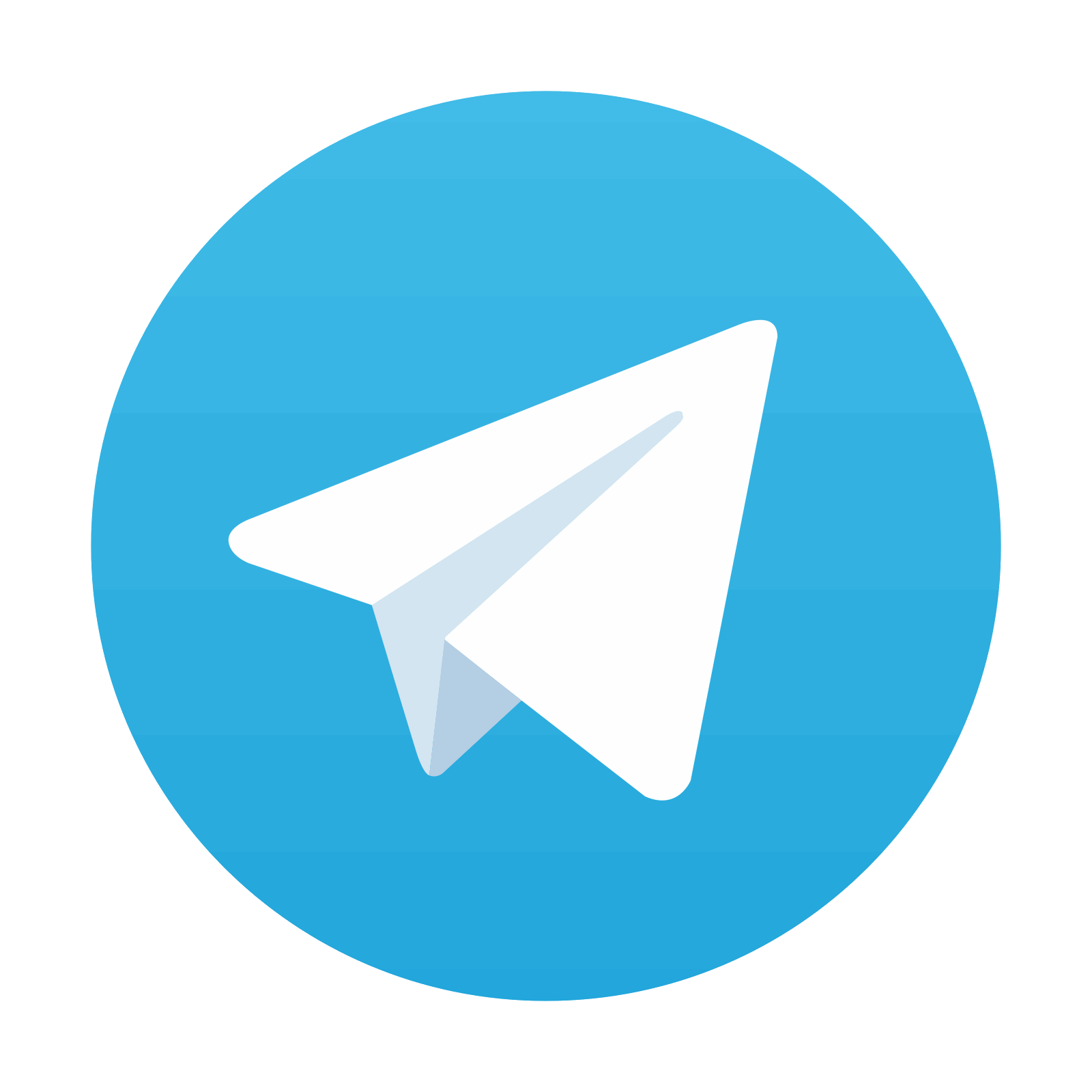
Stay updated, free articles. Join our Telegram channel

Full access? Get Clinical Tree
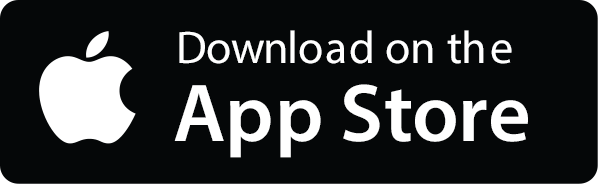
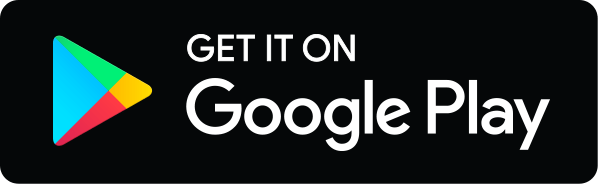
