Chapter 34
Structure and Function of the Pulmonary System
The pulmonary system consists of upper and lower airways, the chest wall, and pulmonary circulation. The primary function of the pulmonary system is the exchange of gases between the environmental air and the blood. There are three steps in this process: (1) ventilation, the movement of air into and out of the lungs; (2) diffusion, the movement of gases between air spaces in the lungs and the bloodstream; and (3) perfusion, the movement of blood into and out of the capillary beds of the lungs to body organs and tissues. The first two functions are carried out by the pulmonary system and the third by the cardiovascular system (see Chapter 31). Normally the pulmonary system functions efficiently under a variety of conditions and with little energy expenditure.
Structures of the Pulmonary System
The pulmonary system is made up of the upper airways, two lungs, the lower airways, and the blood vessels that serve them (Figure 34-1); the chest wall, or thoracic cage; and the diaphragm. The lungs are divided into lobes: three in the right lung (upper, middle, lower) and two in the left lung (upper, lower). Each lobe is further divided into segments and lobules. The space between the lungs, which contains the heart, great vessels, and esophagus, is called the mediastinum. A set of conducting airways, called bronchi, deliver air to each section of the lung. The lung tissue that surrounds the airways supports them, preventing their distortion or collapse as gas moves in and out during ventilation. The diaphragm is a dome-shaped muscle that separates the thoracic and abdominal cavities and is involved in ventilation.
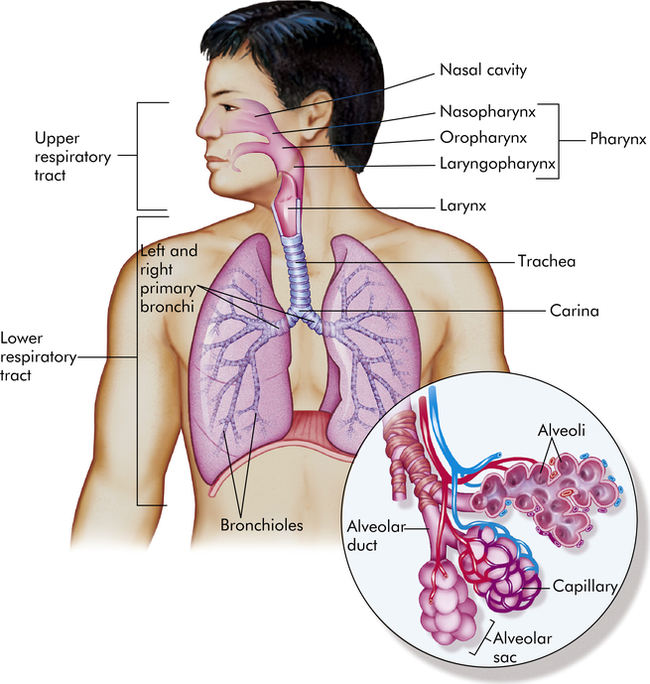
Inset shows alveolar sacs where the interchange of oxygen and carbon dioxide takes place through the walls of the grapelike alveoli. Capillaries surround the alveoli. (From Patton KT, Thibodeau GA: Anatomy & physiology, ed 8, St Louis, 2013, Mosby.)
The lungs are protected from a variety of exogenous contaminants by a series of mechanical and cellular defenses (Table 34-1). These defense mechanisms are so effective that in the healthy individual, contamination of the lung tissue itself is unusual. (Other defense mechanisms are discussed in Chapters 7 and 8.)
TABLE 34-1
STRUCTURE OR SUBSTANCE | MECHANISM OF DEFENSE |
Upper respiratory tract mucosa | Maintains constant temperature and humidification of gas entering the lungs; traps and removes foreign particles, some bacteria, and noxious gases from inspired air |
Nasal hairs and turbinates | Trap and remove foreign particles, some bacteria, and noxious gases from inspired air |
Mucous blanket | Protects trachea and bronchi from injury; traps most foreign particles and bacteria that reach the lower airways |
Cilia | Propel mucous blanket and entrapped particles toward the oropharynx, where they can be swallowed or expectorated |
Alveolar macrophages | Ingest and remove bacteria and other foreign material from alveoli by phagocytosis (see Chapter 7) |
Surfactant | Enhance phagocytosis of pathogens and allergens in alveoli; down-regulate inflammatory responses |
Irritant receptors in nares (nostrils) | Stimulation by chemical or mechanical irritants triggers sneeze reflex, which results in rapid removal of irritants from nasal passages |
Irritant receptors in trachea and large airways | Stimulation by chemical or mechanical irritants triggers cough reflex, which results in removal of irritants from the trachea and large airways |
Conducting Airways
The conducting airways are the portion of the pulmonary system that provides a passage for the movement of air into and out of the gas-exchange portions of the lung. They consist of upper and lower airways. The nasopharynx, oropharynx, and related structures often are called the upper airway (Figure 34-2). These structures are lined with a ciliated mucosa with a very rich vascular supply. The mucosal lining warms and humidifies inspired air to 100% and removes foreign particles from it as it passes into the lungs. During quiet breathing, gas usually flows through the nose, nasopharynx, and oropharynx to the lower airways. The mouth and oropharynx provide for ventilation when the nose is obstructed or when increased flow is required, such as during exercise. Filtering and humidifying are not as efficient with mouth breathing.
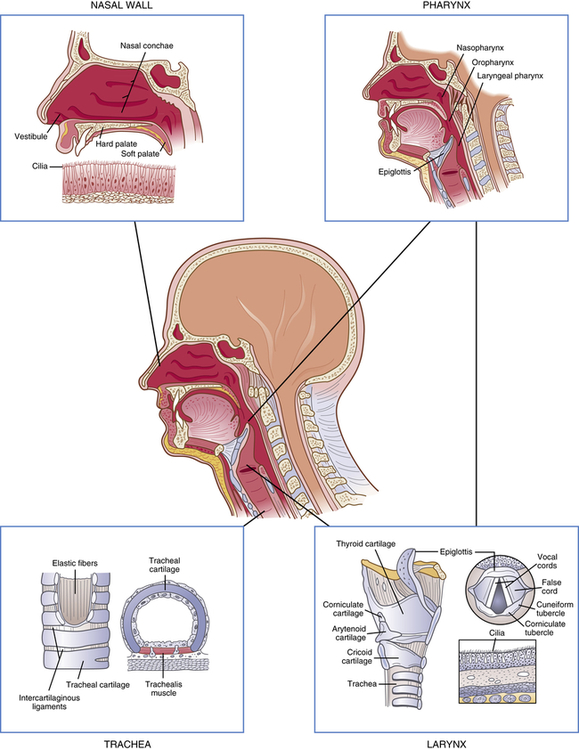
The larynx connects the upper and lower airways. The structure of the larynx consists of the endolarynx and its surrounding triangular-shaped bony and cartilaginous structures. The endolarynx is formed by two pairs of folds that form the false vocal cords (supraglottis) and the true vocal cords. The slit-shaped space between the true cords forms the glottis (see Figure 34-2). The vestibule is the space above the false vocal cords. The laryngeal box is formed by three large cartilages-the epiglottis, thyroid, and cricoid-and three smaller cartilages-the arytenoid, corniculate, and cuneiform-that are connected by ligaments. The supporting cartilages prevent collapse of the larynx during inspiration and swallowing. The internal laryngeal muscles control vocal cord length and tension, and the external laryngeal muscles move the larynx as a whole. Both sets of muscles are important to swallowing, respiration, and vocalization.1 The internal muscles contract during swallowing to prevent aspiration into the trachea and contribute to voice pitch.
The trachea, which is supported by U-shaped cartilage, connects the larynx to the bronchi, the conducting airways of the lungs. The trachea divides into the two main airways, or bronchi, at the carina (see Figure 34-1). This area is very sensitive and when stimulated can cause coughing and airway narrowing. The left mainstem bronchus branches from the trachea at about a 45° angle. The right mainstem bronchus is slightly larger and more vertical than the left (branches at about a 20° to 30° angle from the trachea). Aspirated fluids or foreign particles thus tend to enter the right lung rather than the left. The right and left main bronchi enter the lungs at the hila, or “roots” of the lungs, along with the pulmonary blood and lymphatic vessels. From the hila the main bronchi branch into lobar bronchi, then to segmental and subsegmental bronchi, and finally end at the sixteenth division in the smallest of the conducting airways, the terminal bronchioles (Figure 34-3). With these multiple divisions, the cross-sectional area of the airways increases to 20 times that of the trachea. This results in decreased velocity of airflow into the gas-exchange portion of the lung and allows for optimal gas diffusion.2
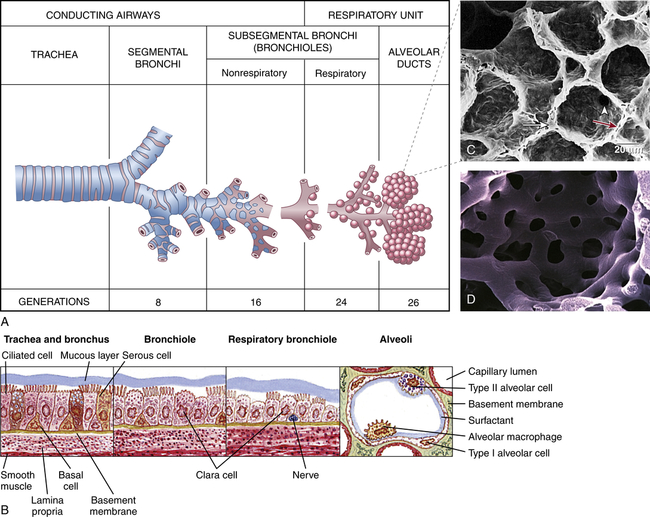
A, Structures of respiratory airways. B, Changes in bronchial wall with progressive branching. C, Electron micrograph of alveoli: long white arrow identifies type II pneumonocyte (secretes surfactant); short white arrow identifies pores of Kohn; red arrow identifies alveolar capillary. D, Plastic cast of pulmonary capillaries at high magnification. (A redrawn from Thompson JM et al: Mosby’s clinical nursing, ed 5, St Louis, 2002, Mosby; B from Wilson SF, Thompson JM: Respiratory disorders, St Louis, 1990, Mosby; C from Mason RJ et al: Murray and Nadel’s textbook of respiratory medicine, ed 5, Philadelphia, 2010, Saunders; D courtesy A. Churg, MD, and J. Wright, MD, Vancouver, Canada. From Leslie KO, Wick MR: Practical pulmonary pathology: a diagnostic approach, ed 2, Philadelphia, 2011, Saunders.)
The bronchial walls have three layers: an epithelial lining, a smooth muscle layer, and a connective tissue layer. In the large bronchi (to approximately the tenth division), the connective tissue layer contains cartilage. The epithelial lining of the bronchi contains single-celled exocrine glands-the mucus-secreting goblet cells-and ciliated cells. High columnar pseudostratified epithelium lines the larger airways, changing to columnar cuboidal epithelium in the bronchioles (types of epithelia are illustrated in Chapter 1). The submucosal glands of the bronchial lining also produce mucus, contributing to the mucous blanket that covers the bronchial epithelium. The ciliated epithelial cells rhythmically beat this mucous blanket toward the trachea and pharynx, where it can be swallowed or expectorated by coughing. Foreign particles and microorganisms that are not expelled by mucociliary clearance and coughing are attacked by cellular components of the inflammatory response and antibodies of the secretory immune system (see Chapter 8).3 The biochemical mediators released early in inflammation also play a part in antibody-mediated hypersensitivity reactions, such as asthma, because they stimulate bronchial smooth muscles to constrict. With branching, the layers of epithelium that line the bronchi become thinner (see Figure 34-3). Ciliated cells and goblet cells become more sparse, and smooth muscle and connective tissue layers thin toward the terminal bronchioles.4
Gas-Exchange Airways
The bronchioles terminate in gas-exchange airways, where oxygen (O2) enters the blood and carbon dioxide (CO2) is removed from it. The gas-exchange airways consist of respiratory bronchioles, alveolar ducts, and alveoli (see Figure 34-3). These structures together are sometimes called the acinus, and all of them participate in gas exchange.5
The alveoli are the primary gas-exchange units of the lung, where oxygen enters the blood and CO2 is removed (Figure 34-4). Tiny passages called pores of Kohn permit some air to pass through the septa from alveolus to alveolus, promoting collateral ventilation and even distribution of air among the alveoli. The lungs contain approximately 50 million alveoli at birth and about 480 million by adulthood.4,6
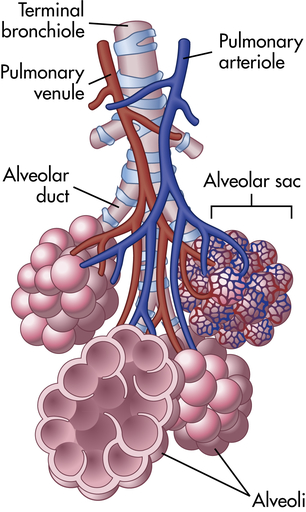
Bronchioles subdivide to form tiny tubes called alveolar ducts that end in clusters of alveoli called alveolar sacs. (From Patton KT, Thibodeau GA: Anatomy & physiology, ed 8, St Louis, 2013, Mosby.)
The alveolar septa consist of an epithelial layer and a thin, elastic basement membrane but no muscle layer (see Figure 34-3). Two major types of epithelial cells appear in the alveolus. Type I alveolar cells provide structure, and type II alveolar cells secrete surfactant, a lipoprotein that coats the inner surface of the alveolus and facilitates its expansion during inspiration, lowers alveolar surface tension at end-expiration, and, thereby, prevents lung collapse.7 Surfactant also contributes to control of lung inflammation and innate and adaptive immunity.7a
Like the bronchi, alveoli contain cellular components of inflammation and immunity, particularly the mononuclear phagocytes. The mononuclear phagocytes of the lungs are called alveolar macrophages. These cells ingest foreign material that reaches the alveolus and prepare it for removal through the lymphatics.8 (Phagocytosis and the mononuclear phagocyte system are described in Chapters 7 and 8.)
Pulmonary and Bronchial Circulation
The pulmonary circulation facilitates gas exchange, delivers nutrients to lung tissues, acts as a blood reservoir for the left ventricle, and serves as a filtering system that removes clots, air, and other debris from the circulation (Figure 34-5).
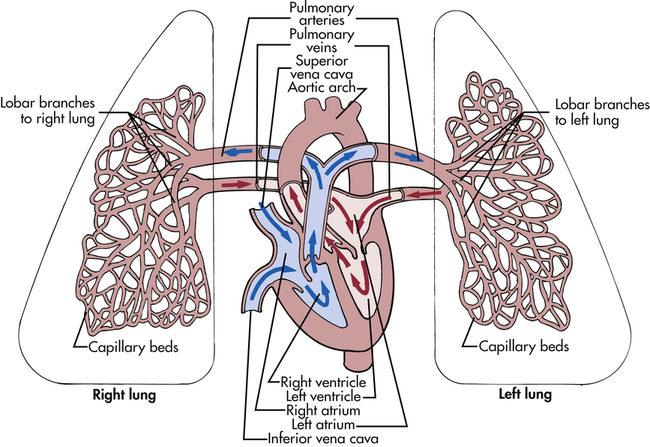
The right and left pulmonary veins and arteries and the branching capillaries are illustrated. Note the pulmonary artery carries venous blood, and the pulmonary vein carries arterial blood.
Although the entire cardiac output from the right ventricle goes into the lungs, the pulmonary circulation has a lower pressure and resistance than the systemic circulation. Pulmonary arteries are exposed to about one fifth the pressure of the systemic circulation and have a much thinner muscle layer. (Systemic vessels are described in Chapter 31.) Mean pulmonary artery pressure is 18 mmHg; mean aortic pressure is 90 mmHg. About one third of the pulmonary vessels are filled with blood (perfused) at any given time.9 More vessels become perfused when right ventricular cardiac output increases. Therefore, increased delivery of blood to the lungs does not normally increase mean pulmonary artery pressure.
The pulmonary artery divides and enters the lung at the hilus, travels with each main bronchus, and branches with the bronchus at every division so that every bronchus and bronchiole has an accompanying artery or arteriole. The arterioles, less than 1 mm in diameter, regulate blood flow through their respective capillary beds.9
The arterioles divide at the terminal bronchiole to form a network of pulmonary capillaries around the acinus. The capillaries are an integral part of the alveolar septa. Capillary walls consist of an endothelial layer and a thin basement membrane, which often fuses with the basement membrane of the alveolar septum (see Figure 34-3). This results in very little separation between blood in the capillary and gas in the alveolus.
The shared alveolar and capillary walls compose the alveolocapillary membrane, a very thin membrane made up of the alveolar epithelium, the alveolar basement membrane, an interstitial space, the capillary basement membrane, and the capillary endothelium (Figure 34-6). These extremely thin alveolar walls are easily damaged and can leak plasma and blood into the alveolar space. Gas exchange occurs across the alveolocapillary membrane. With normal perfusion approximately 100 ml of blood in the pulmonary capillary bed is spread very thinly over about 140 m2 of alveolar surface area.9 The alveolocapillary membrane efficiently exposes large quantities of blood to gas in the alveoli. Any disorder that thickens the membrane impairs gas exchange.
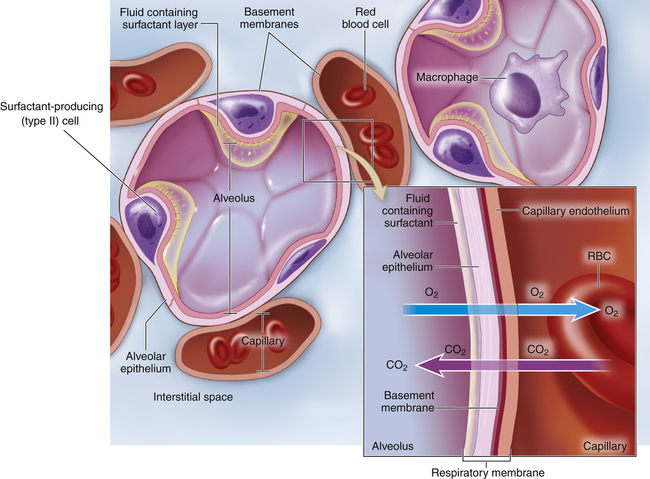
Inset shows a magnified view of the respiratory membrane composed of the alveolar wall (fluid containing surfactant, epithelial cells, basement membrane), interstitial space, and wall of a pulmonary capillary (basement membrane, endothelial cells). The gases-carbon dioxide (CO2) and oxygen (O2)-diffuse across the respiratory membrane. (From Patton KT, Thibodeau GA: Anatomy & physiology, ed 8, St Louis, 2013, Mosby.)
The bronchial circulation is part of the systemic circulation. It supplies nutrients to the conducting airways, nerves, lymph nodes, large pulmonary vessels, and membranes (pleurae) that surround the lungs and moistens inspired air.10 The bronchial circulation is unique in that not all of its capillaries drain into its own venous system. Some of the bronchial capillaries empty into the pulmonary vein and contribute to the normal venous admixture (mixing of oxygenated and deoxygenated blood) or right-to-left shunt (right-to-left shunts [ventilation-perfusion abnormalities] are described in Chapter 35). The bronchial circulation does not participate in gas exchange.
Lung vasculature also includes deep and superficial pulmonary lymphatic capillaries. The deep lymphatic capillaries begin at the level of the terminal bronchioles; there are no lymphatic structures in the acinus. Fluid and alveolar macrophages migrate from the alveoli to the terminal bronchioles, where they enter the lymphatic system. The superficial lymphatic capillaries drain the membrane that surrounds the lungs. Both deep and superficial lymphatic vessels leave the lung at the hilus through a series of mediastinal lymph nodes. The lymphatic system plays an important role in keeping the lung free of fluid and providing immune defense. (The lymphatic system is described in Chapter 31.)
Control of the Pulmonary Circulation
The most important cause of pulmonary artery constriction is a low alveolar partial pressure of oxygen (Pao2). Vasoconstriction caused by alveolar and pulmonary venous hypoxia, often termed hypoxic pulmonary vasoconstriction, can affect only one portion of the lung or the entire lung. If only one segment of the lung is involved, the arterioles to that segment constrict, shunting blood to other, well-ventilated portions of the lung. This reflex improves the lung’s efficiency by better matching ventilation and perfusion. If alveolar hypoxia affects all segments of the lung, however, vasoconstriction occurs throughout the pulmonary vasculature, and pulmonary hypertension (elevated pulmonary artery pressure) can result. The pulmonary vasoconstriction caused by low Pao2 is reversible if the Pao2 is corrected. Chronic alveolar hypoxia can result in permanent pulmonary artery hypertension, which eventually leads to cor pulmonale and heart failure (see What’s New? Update on Hypoxic Pulmonary Vasoconstriction).11
Acidemia also causes pulmonary artery constriction. If the acidemia is corrected, the vasoconstriction is reversed. (Respiratory acidosis and metabolic acidosis are described in Chapter 3.) It is important to note that an elevated Paco2 without a drop in pH does not cause pulmonary artery constriction. Other biochemical factors that affect the caliber of vessels in pulmonary circulation are histamine, prostaglandins, endothelin, serotonin, nitric oxide, and bradykinin.
Chest Wall and Pleura
The chest wall (skin, ribs, intercostal muscles) protects the lungs from injury, and its muscles, in conjunction with the diaphragm, perform the muscular work of breathing. The thoracic cavity is contained by the chest wall and encases the lungs (Figure 34-7). A serous membrane called the pleura adheres firmly to the lungs. It then folds over itself and attaches firmly to the chest wall. The membrane covering the lungs is the visceral pleura; that lining the thoracic cavity is the parietal pleura. The area between the two pleurae is called the pleural space, or pleural cavity. Normally only a thin layer of fluid secreted by the pleura (pleural fluid) fills the pleural space. About 18 ml of fluid is in the pleural space with a pH of about 7.6, a few cells, about 1 g/dl protein, and glucose and electrolyte concentrations that approximate those of the plasma.9 This lubricates the pleural surfaces, allowing the two layers to slide over each other without separating. Pressure in the pleural space is usually negative or subatmospheric (−4 to −10 mmHg).
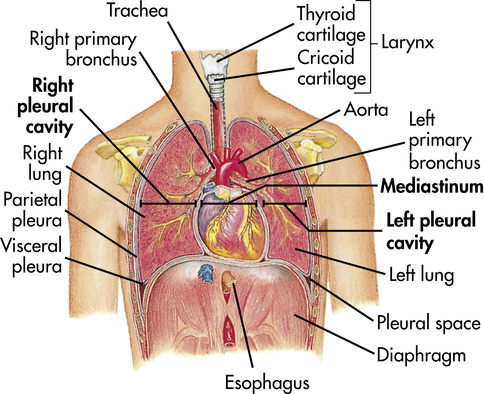
The thoracic cavity is divided into three subdivisions (left and right pleural divisions and mediastinum) by a partition formed by a serous membrane called the pleura. (From Thibodeau GA, Patton KT: Anatomy & physiology, ed 3, St Louis, 1996, Mosby.)
Functions of the Pulmonary System
The pulmonary system functions to (1) ventilate the alveoli, (2) diffuse gases into and out of the blood, and (3) perfuse the lungs so that the organs and tissues of the body receive blood that is rich in oxygen and low in CO2. Each component of the pulmonary system contributes to one or more of these functions (Figure 34-8).
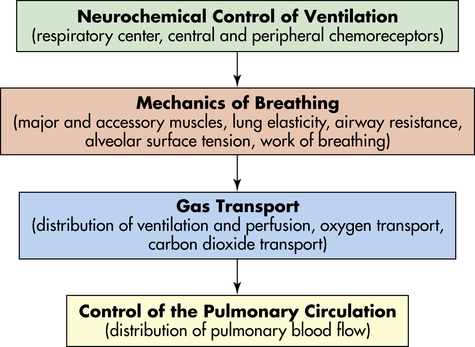
The central nervous system responds to neurochemical stimulation of ventilation and sends signals to the chest wall musculature. The response of the respiratory system to these impulses is influenced by several factors that affect the mechanisms of breathing and therefore affect the adequacy of ventilation. Gas transport between the alveoli and pulmonary capillary blood depends on a variety of physical and chemical activities. The control of the pulmonary circulation plays a role in the appropriate distribution of blood flow.
Ventilation
CO2, the gaseous form of carbonic acid (H2CO3), is a product of cellular metabolism. The lung eliminates about 10,000 milliequivalents (mEq) of carbonic acid per day in the form of CO2, which is produced at the rate of approximately 200 ml/minute. CO2 elimination is necessary to maintain a normal partial pressure of arterial CO2 (Paco2) of 40 mmHg and normal acid-base balance (see Chapter 3 for a discussion of acid-base regulation).9
Neurochemical Control of Ventilation
The mechanisms that control respiration are very complex.12–14 Breathing is usually involuntary because homeostatic changes in the ventilatory rate and volume are adjusted automatically by the nervous system to maintain normal gas exchange. Voluntary breathing is necessary for talking, singing, laughing, and holding one’s breath.
The respiratory center in the brainstem controls respiration by transmitting impulses to the respiratory muscles, causing them to contract and relax (Figure 34-9). The respiratory center is composed of several groups of neurons located bilaterally in the brainstem: the dorsal respiratory group (DRG), the ventral respiratory group (VRG), the pneumotaxic center, and the apneustic center.15 The basic automatic rhythm of respiration is set by the DRG, a cluster of inspiratory nerve cells located in the medulla that sends efferent impulses to the diaphragm and inspiratory intercostal muscles. The DRG also receives afferent impulses from peripheral chemoreceptors in the carotid and aortic bodies, which detect the Paco2 and the amount of oxygen in the arterial blood (Pao2). In addition, several different types of receptors in the lungs stimulate the VRG through afferent nerves. The VRG, also located in the medulla, contains inspiratory and expiratory neurons. It is almost inactive during normal, quiet respiration, becoming active when increased ventilatory effort is required. The pneumotaxic center and apneustic center, situated in the pons, do not generate primary rhythm, but rather act as modifiers of the inspiratory depth and rate established by the medullary centers.16 Breathing can be modified by input from the cortex, the limbic system, and the hypothalamus, and the pattern of breathing can be influenced by emotion and by disease.
Lung Receptors
Three types of lung receptors send impulses from the lungs to the dorsal respiratory group:
1. Irritant receptors (rapidly adapting receptors) are found in the epithelium of the conducting airways. They are sensitive to noxious aerosols (vapors), gases, and particulate matter (e.g., inhaled dusts), which cause them to initiate the cough reflex. When stimulated, irritant receptors also cause bronchoconstriction and increased ventilatory rate. These receptors are located primarily in the proximal larger airways and are nearly absent in the distal airways; thus it is possible for secretions to accumulate in the distal respiratory tree without initiating cough.
2. Stretch receptors (slowly adapting receptors) are located in the smooth muscles of airways and are sensitive to increases in the size or volume of the lungs. They decrease ventilatory rate and volume when stimulated, an occurrence sometimes referred to as the Hering-Breuer expiratory reflex. This reflex is active in newborns and assists with ventilation.17 In adults, this reflex is active only at high tidal volumes (such as with exercise and mechanical ventilation) and may play a role in protecting against excess lung inflation. Stretch receptors called rapidly adapting receptors (RARs) have been found to be an important mediator of cough.18
3. J-receptors (juxta-pulmonary capillary or pulmonary C fiber receptors) are located near the capillaries in the alveolar septa. They are sensitive to increased pulmonary capillary pressure, which stimulates them to initiate rapid, shallow breathing; laryngeal constriction on expiration and mucus secretion; hypotension; and bradycardia.19
The lung is innervated by the autonomic nervous system (ANS). Fibers of the sympathetic division of the ANS in the lung branch from the upper thoracic and cervical ganglia of the spinal cord. Fibers of the parasympathetic division of the ANS travel in the vagus nerve to the lung. (Structures and function of the ANS are discussed in detail in Chapter 15.) The parasympathetic and sympathetic divisions of the ANS control airway caliber (interior diameter of the airway lumen) by stimulating bronchial smooth muscle to contract or relax. The parasympathetic receptors cause smooth muscle to contract, whereas sympathetic receptors cause it to relax. Bronchial smooth muscle tone depends on equilibrium, that is, equal stimulation of contraction and relaxation. The parasympathetic division of the ANS is the main controller of airway caliber under normal conditions.20 Constriction occurs if the irritant receptors in the airway epithelium are stimulated by irritants in inspired air, by inflammatory mediators (e.g., histamine, serotonin, prostaglandins), by many drugs, and by humoral substances.
Chemoreceptors
Chemoreceptors monitor pH, Paco2, and Pao2. Central chemoreceptors monitor arterial blood indirectly by sensing changes in the pH of cerebrospinal fluid (CSF). They are located near the respiratory center and are sensitive to hydrogen ion concentration in the CSF. (Chapter 3 describes the relationship between ions and the pH, or acid-base status, of body fluids.) The pH, or concentration of hydrogen ions in the CSF, reflects Paco2 because, unlike H+ ions, CO2 in arterial blood diffuses across the blood-brain barrier (the capillary wall separating blood from cells of the central nervous system) into the CSF until the partial pressure of CO2 (Pco2) is equal on both sides. CO2 that has entered the CSF combines with H2O to form carbonic acid, which subsequently dissociates into hydrogen ions that are capable of stimulating the central chemoreceptors. In this way Paco2 regulates ventilation through its effect on the pH (hydrogen ion content) of the CSF.12,13,21
The central chemoreceptors are sensitive to very small changes in the pH of CSF (equivalent to a 1 to 2 mmHg change in Pco2) and are able to maintain a normal Paco2 under many different conditions, including strenuous exercise. If inadequate ventilation, or hypoventilation, is long term (e.g., in chronic obstructive pulmonary disease), these receptors become insensitive to small changes in Paco2 and regulate ventilation poorly. In addition, prolonged increases in Paco2 result in renal compensation through bicarbonate retention. This bicarbonate gradually diffuses into the CSF, where it normalizes the pH and negates the effect on ventilatory drive.22
The peripheral chemoreceptors are located in aortic bodies, the aortic arch, and carotid bodies at the bifurcation of the carotids, near the baroreceptors (see Chapter 31). Although the peripheral chemoreceptors are sensitive to changes in Paco2 and pH, they are primarily sensitive to oxygen levels in arterial blood (Pao2) and are responsible for all of the increase in ventilation that occurs in response to arterial hypoxemia.23 As Pao2 and pH decrease, peripheral chemoreceptors, particularly in the carotid bodies, send signals to the respiratory center to increase ventilation. The peripheral chemoreceptors are not as sensitive as the central chemoreceptors. The Pao2 must drop well below normal (to approximately 60 mmHg) before the peripheral chemoreceptors have much influence on ventilation.9 If Paco2 is elevated as well, however, ventilation increases much more than it would in response to either abnormality alone. The peripheral chemoreceptors become the major stimulus to ventilation when the central chemoreceptors are “reset” by chronic hypoventilation.
Mechanics of Breathing
Major and Accessory Muscles
The major muscles of inspiration are the diaphragm and the external intercostal muscles (muscles between the ribs) (Figure 34-10). The diaphragm is a dome-shaped muscle that separates the abdominal and thoracic cavities. When the diaphragm contracts, it flattens downward, increasing the volume of the thoracic cavity, and creates a negative pressure that draws gas into the lungs through the upper airways and trachea. Contraction of external intercostal muscles elevates the anterior portion of the ribs. This increases the volume of the thoracic cavity by increasing its front-to-back (anteroposterior [AP]) diameter. Although the external intercostal muscles may contract during quiet breathing, inspiration at rest usually is assisted by the diaphragm only.
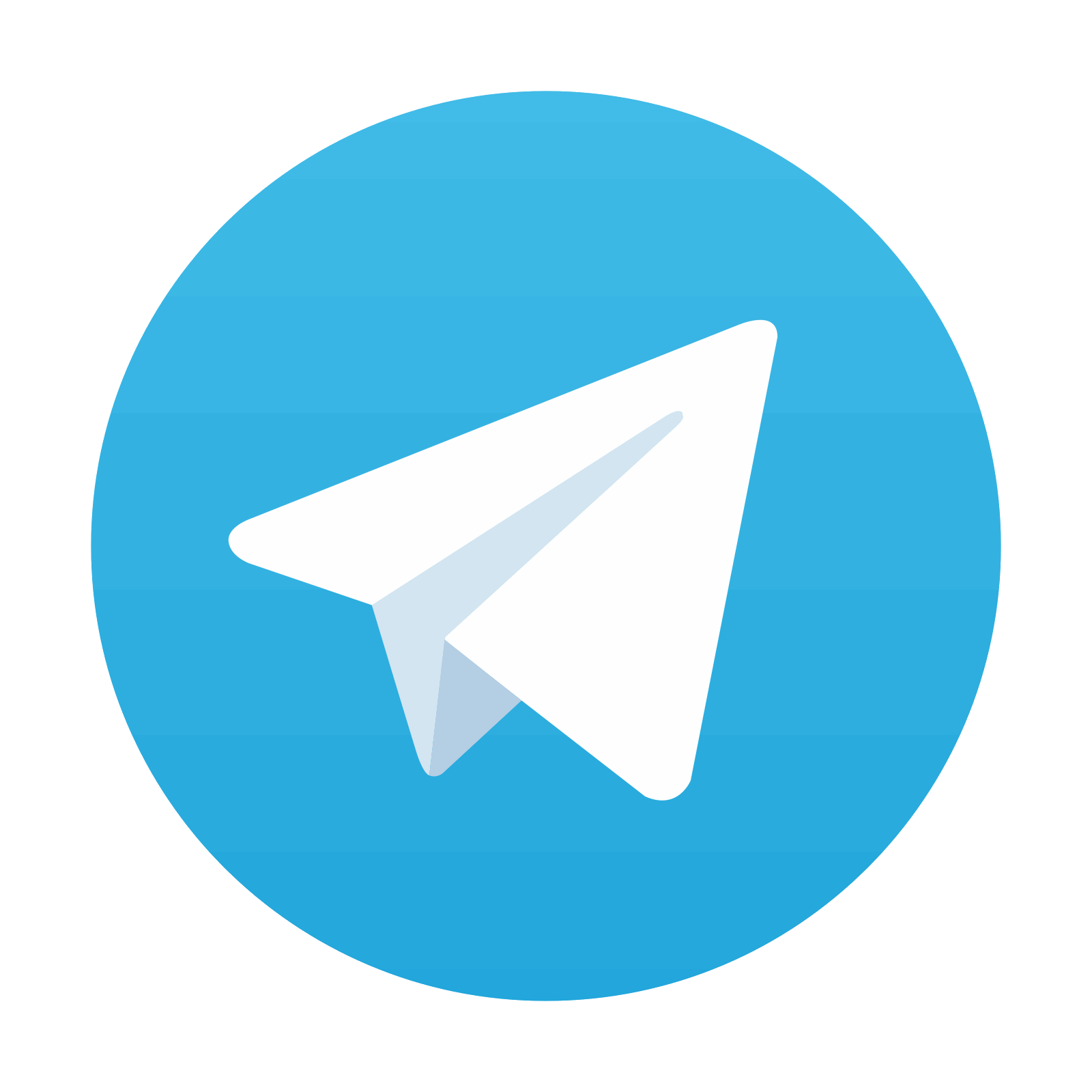
Stay updated, free articles. Join our Telegram channel

Full access? Get Clinical Tree
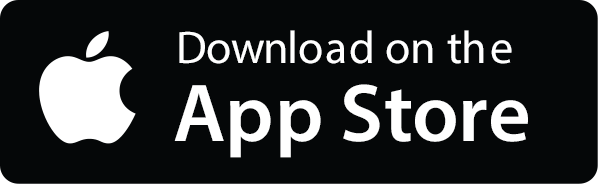
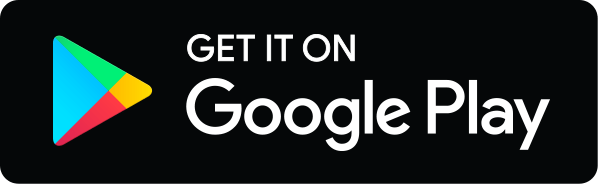
