Chapter 27
Structure and Function of the Hematologic System
Neal S. Rote and Kathryn L. McCance
Components of the Hematologic System
Composition of the Blood
Blood consists of various cells that circulate in the cardiovascular system suspended in a solution of protein and inorganic materials (plasma), which is approximately 92% water and 8% dissolved substances (solutes) (Figure 27-1). The blood volume amounts to about 6 quarts (5.5 L) in adults. The continuous movement of blood guarantees that critical components are available to all parts of the body to carry out their chief functions: (1) delivery of substances needed for cellular metabolism in the tissues, (2) removal of the wastes of cellular metabolism, (3) defense against invading microorganisms and injury, and (4) maintenance of acid-base balance.
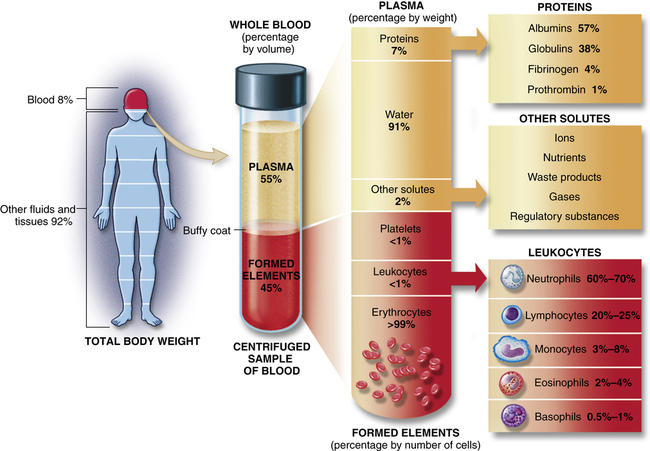
Approximate values for the components of blood in a normal adult. (From Patton KT, Thibodeau GA: Anatomy & physiology, ed 8, St Louis, 2013, Mosby.)
Plasma and Plasma Proteins
In adults, plasma accounts for 50% to 55% of blood volume. Plasma is a complex aqueous liquid containing a variety of organic and inorganic elements (Table 27-1). The concentration of these elements varies depending on diet, metabolic demand, hormones, and vitamins. Plasma differs from serum in that serum is plasma that has been allowed to clot in the laboratory in order to remove fibrinogen and other clotting factors that may interfere with some diagnostic tests.
TABLE 27-1
ORGANIC AND INORGANIC COMPONENTS OF ARTERIAL PLASMA
CONSTITUENT | AMOUNT/CONCENTRATION | MAJOR FUNCTIONS |
Water | 93% of plasma weight | Medium for carrying all other constituents |
Electrolytes | Total <1% of plasma weight | Maintain H2O in extracellular compartment; act as buffers; function in membrane excitability |
Na+ | 142 mEq/L (142 mM) | |
K+ | 4 mEq/L (4 mM) | |
Ca2+ | 5 mEq/L (2.5 mM) | |
Mg2+ | 3 mEq/L (1.5 mM) | |
Cl | 103 mEq/L (103 mM) | |
![]() | 27 mEq/L (27 mM) | |
Phosphate (mostly) | 2 mEq/L (1 mM) | |
Sulfate | 1 mEq/L (0.5 mM) | |
Proteins | 7.3 g/dl (2.5 mM) | Provide colloid osmotic pressure of plasma; act as buffers; bind other plasma constituents (lipids, hormones, vitamins, minerals, etc.); clotting factors; enzymes; enzyme precursors; antibodies (immunoglobulins); hormones; transporters |
Albumin | 4.5 g/dl | |
Globulins | 2.5 g/dl | |
Fibrinogen | 0.3 g/dl | |
Transferrin | 250 mg/dl | |
Ferritin | 15-300 mcg/L | |
Gases | ||
CO2 content | 22-32 mmol/L plasma | Byproduct of oxygenation, most CO2 content is from HCO3 and acts as a buffer |
O2 | Pao2 80 torr or greater (arterial); Pvo2 30-40 torr (venous) | Oxygenation |
N2 | 0.9 ml/dl | Byproduct of protein catabolism |
Nutrients | Provide nutrition and substances for tissue repair | |
Glucose and other carbohydrates | 100 mg/dl (5.6 mM) | |
Total amino acids | 40 mg/dl (2 mM) | |
Total lipids | 500 mg/dl (7.5 mM) | |
Cholesterol | 150-250 mg/dl (4-7 mM) | |
Individual vitamins | 0.0001-2.5 mg/dl | |
Individual trace elements | 0.001-0.3 mg/dl | |
Iron | 50-150 mcg/dl | |
Waste products | ||
Urea (blood urea nitrogen [BUN]) | 7-18 mg/dl (5.7 mM) | End product of protein catabolism |
Creatinine (from creatine) | 1 mg/dl (0.09 mM) | End product from energy metabolism |
Uric acid (from nucleic acids) | 5 mg/dl (0.3 mM) | End product from protein metabolism |
Bilirubin (from heme) | 0.2-1.2 mg/dl (0.003-0.018 mM) | End product of red blood cell destruction |
Individual hormones | 0.000001-0.05 mg/dl | Functions specific to target tissue |
Data from Vander AJ, Sherman JH, Luciano DS: Human physiology: the mechanisms of body function, ed 8, New York, 2001, McGraw-Hill.
The plasma contains a large number of proteins (plasma proteins) that constitute about 7% of the total plasma weight. These vary in structure and function and can be classified into two major groups, albumin and globulins. Most plasma proteins are produced by the liver. The major exception is antibodies (immunoglobulins), which are produced by plasma cells in the lymph nodes and other lymphoid tissues (see Chapter 8).
Albumin (about 60% of total plasma protein at a concentration of about 4 g/dl) serves as a carrier molecule for normal components of blood as well as drugs that have low solubility in water (e.g., free fatty acids, lipid-soluble hormones, thyroid hormones, bile salts). Its most essential role is regulation of the passage of water and solutes through the capillaries. Albumin molecules are large and do not diffuse freely through the vascular endothelium, and thus they maintain the critical colloidal osmotic pressure (or oncotic pressure) that regulates the passage of water and solutes into the surrounding tissues (see Chapters 1 and 3). Water and solute particles tend to diffuse out of the arterial portions of the capillaries because the blood pressure is greater in arterial than in venous blood vessels (see Chapter 3). Water and solutes move from tissue cells into the venous portions of the capillaries where the pressures are reversed, oncotic pressure being greater than intravascular pressure or hydrostatic pressure. In the case of decreased production (e.g., cirrhosis, other diffuse liver diseases, protein malnutrition) or excessive loss of albumin (e.g., certain kidney diseases, extensive burns), the reduced oncotic pressure leads to excessive movement of fluid and solutes into the tissue and decreased blood volume.
The remaining plasma proteins, or globulins, are often classified by their properties in an electric field (serum electrophoresis). Under the normal conditions used to perform serum electrophoresis, albumin is the most rapidly moving protein. The globulins are classified by their movement relative to albumin: alpha (α) globulins (those moving most closely to albumin), beta (β) globulins, and gamma (γ) globulins (those with the least movement). Depending on the electrophoretic procedure the alpha and beta globulins may be subdivided into subregions (α1-, α2-, β1-, or β2-globulins). Fibrinogen is a major plasma protein (about 4% of total plasma protein) that would move between the beta and gamma regions but is removed during the formation of serum. The gamma-globulin region consists primarily of immunoglobulin G (IgG) (see Chapter 8).
Plasma proteins can also be classified into groups by function: clotting, defense, transport, or regulation. The clotting factors promote coagulation and stop bleeding from damaged blood vessels. Fibrinogen is the most plentiful of the clotting factors and is the precursor of the fibrin clot. Proteins involved in defense, or protection, against infection include antibodies and complement proteins (see Chapters 7 and 8). Transport proteins specifically bind and carry a variety of inorganic and organic molecules, including iron (transferrin), copper (ceruloplasmin), steroid hormones, and vitamins (e.g., retinol-binding protein). The plasma lipids, triglycerides, phospholipids, cholesterol, and fatty acids are carried through the blood as complexes with plasma proteins; they are known as lipoproteins (see Chapters 1 and 32). Regulatory proteins include a variety of enzymatic inhibitors (e.g., α1-antitrypsin) that protect the tissues from damage, precursor molecules (e.g., kininogen) that are converted into active biologic molecules when needed, and protein hormones (e.g., cytokines) that communicate between cells.
Plasma also contains several charged inorganic ions (electrolytes) that regulate cell function, osmotic pressure, and blood pH. (Electrolytes are described in Chapters 1 and 3.)
Cellular Components of the Blood
The cellular elements of the blood are broadly classified as red blood cells (RBCs) (i.e., erythrocytes), white blood cells (WBCs) (i.e., leukocytes), and platelets (thrombocytes). The components of the blood are listed in Table 27-2.
TABLE 27-2
CELLULAR COMPONENTS OF THE BLOOD
CELL | STRUCTURAL CHARACTERISTICS∗ | NORMAL AMOUNTS OF CIRCULATING BLOOD | FUNCTION | LIFE SPAN |
Erythrocyte (red blood cell) | Nonnucleated cytoplasmic disk containing hemoglobin | 4.2-6.2 million/mm3 | Gas transport to and from tissue cells and lungs | 80-120 days |
Leukocyte (white blood cell) | Nucleated cell | 5000-10,000/mm3 | Body defense mechanisms | See below |
Lymphocyte | Mononuclear immunocyte | 25-33% of leukocyte count (leukocyte differential) | Humoral and cell-mediated immunity (see Chapter 7) | Days or years depending on type |
Monocyte and macrophage | Large kidney-shaped mononuclear phagocyte | 3-7% of leukocyte differential | Phagocytosis; mononuclear phagocyte system | Months or years |
Eosinophil | Segmented polymorphonuclear granulocyte with granules stainable by eosin dyes | 1-4% of leukocyte differential | Phagocytosis; response to parasites, control of allergic reactions | 8-12 days |
Neutrophil | Segmented polymorphonuclear granulocyte with granules stainable by neutral staining | 57-67% of leukocyte differential | Phagocytosis, particularly during early phase of inflammation, bacterial killing | 4 days |
Basophil | Lobate nuclear granulocyte with granules stainable by basic dyes | 0-0.75% of leukocyte differential | Similar to mast cell, secretes inflammatory mediators (e.g., histamine, chemotactic factors for eosinophils and neutrophils), involved with allergic reactions | Few hours to days |
Platelet | Irregularly shaped cytoplasmic fragment (not a cell) | 140,000-340,000/mm3 | Hemostasis following vascular injury; normal coagulation and clot formation/retraction | 8-11 days |
∗See bottom row of Figure 27-10 for illustrations of cells.
Erythrocytes
In 1628 Robert Burton described blood as a “hot, temperate red humor whose office is to nourish the whole body, to give it strength and color being dispersed by the veins through every part of it.”1 A few years later, with the invention of the microscope, researchers learned that erythrocytes give blood its red color.
The erythrocyte’s size and shape are ideally suited to its function as a gas carrier. An RBC is a small disk with two unique properties: (1) a biconcave shape and (2) the capacity to be reversibly deformed (Figure 27-2 and Figure 27-6). The flattened, biconcave shape provides a surface area/volume ratio that is optimal for gas diffusion into and out of the cell and for deformity.2 During its life span, the erythrocyte, which is 6 to 8 μm in diameter, repeatedly circulates through sinusoids of the spleen and capillaries that are only 2 μm in diameter. Reversible deformity enables the erythrocyte to assume a more compact torpedo-like shape, squeeze through the microcirculation, and return to normal.
Leukocytes
Leukocytes (white blood cells [WBCs]) defend the body against microorganisms that cause infection and remove debris, including dead or injured cells of all kinds (see Figure 27-2). The leukocytes act primarily in the tissues but are transported in the circulation. The average adult has approximately 5000 to 10,000 leukocytes/mm3 of blood.
Leukocytes are classified according to structure as either granulocytes or agranulocytes and according to function as either phagocytes or immunocytes. The granulocytes, which include neutrophils, basophils, and eosinophils, are all phagocytes. (Phagocytic action is described in Chapter 7.) Of the agranulocytes, the monocytes and macrophages are phagocytes, whereas the lymphocytes are immunocytes (cells that create immunity; see Chapter 8).
Granulocytes
Granulocytes have many membrane-bound granules in their cytoplasm. These granules contain enzymes capable of killing microorganisms and catabolizing debris ingested during phagocytosis. The granules also contain powerful biochemical mediators with inflammatory and immune functions. These mediators, along with the digestive enzymes, are released from granulocytes in response to specific stimuli. The biochemical mediators have vascular and intercellular effects and the enzymes participate in the breakdown of debris from sites of infection or injury. Granulocytes are capable of amoeboid movement, by which they migrate through vessel walls (diapedesis) and then to sites where their action is needed (see Chapter 7).
The neutrophil (polymorphonuclear neutrophil [PMN]) is the most numerous and best understood of the granulocytes (Figure 27-3, A). Neutrophils constitute about 55% of the total leukocyte count in adults. The cytoplasm of neutrophils contains small lysosomal granules and a central nucleus with two to five distinct lobes. Immature neutrophils are called bands or stabs. Mature neutrophils are called segmented neutrophils because of the characteristic appearance of their nucleus. Neutrophils reach a fully mature state in the bone marrow, and these mature neutrophils are called the marrow neutrophil reserve. Normally it takes about 14 days for neutrophils to develop from early precursors, but this process is accelerated by infection and treatment with colony-stimulating factors.
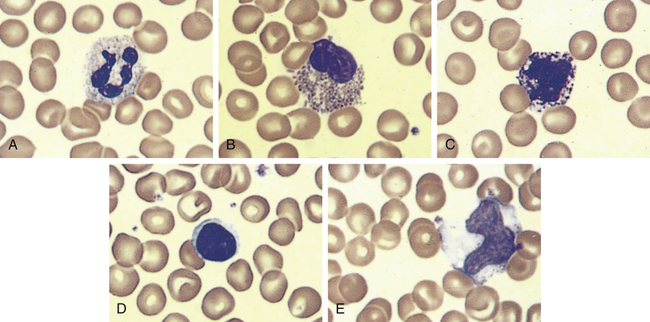
An example of leukocytes in a human blood smear. A, Neutrophil. B, Eosinophil. C, Basophil. D, Lymphocyte. E, Monocyte. (From Erlandsen S, Magney J: Color atlas of histology, St Louis, 1992, Mosby.)
Neutrophils are the chief phagocytes of early inflammation. Soon after bacterial invasion or tissue injury, neutrophils migrate out of the capillaries and into the inflamed site, where they ingest and destroy microorganisms and debris and then die in 1 or 2 days. The dissolution of dead neutrophils releases digestive enzymes from their cytoplasmic granules. These enzymes dissolve cellular debris and prepare the site for healing. (This final function, called débridement, is described in Chapter 7).
Eosinophils have large, coarse granules and constitute only 1% to 4% of the normal leukocyte count in adults (see Figure 27-3, B). Like neutrophils, eosinophils are capable of amoeboid movement and phagocytosis. Using a spectrum of pattern-recognition receptors, eosinophils ingest antigen-antibody complexes and viruses and are induced by mast cell chemotactic factors to attack parasites.3 Eosinophil secondary granules contain toxic chemicals (e.g., major basic protein, eosinophil cationic protein, eosinophil peroxidase, eosinophil-derived neurotoxin) that are highly destructive to parasites and viruses. The eosinophil granules contain a variety of enzymes (e.g., histaminase) that help to control inflammatory processes. (Their function in inflammation and defense against parasites is described in Chapters 7 and 8.) Eosinophils also release leukotrienes, prostaglandins, platelet-activating factor (PAF), and a variety of cytokines (e.g., interleukin-1 [IL-1], IL-6, tumor necrosis factor-alpha [TNF-α], granulocyte-macrophage colony-stimulating factor [GM-CSF]) and chemokines (e.g., IL-8) that augment the inflammatory response.4 Type I hypersensitivity allergic reactions and asthma are characterized by high numbers of circulating eosinophils, which may be involved in a dual role of regulation of inflammation and may contribute to the destructive inflammatory processes observed in the lungs of persons with asthma (see Chapter 9).
Basophils, which comprise less than 1% (0.01% to 0.3%) of the leukocytes, contain cytoplasmic granules that have an abundant mixture of biochemical mediators, including histamine, chemotactic factors, proteolytic enzymes (e.g., elastase, lysophospholipase), and an anticoagulant (heparin) (see Figure 27-3, C). Stimulation of basophils also induces synthesis of vasoactive lipid molecules (e.g., leukotrienes) and cytokines. Basophils produce IL-6 which induces IL-10 by Th1 cells and induction of Th2 cells that favor B-cell differentiation.5 Basophils also are a particularly rich source of the cytokine IL-4, which preferentially guides B-cell differentiation toward plasma cells that secrete IgE (see Chapter 8).
Mast cells are highly similar to basophils, but are generated from a different set of precursor cells in the bone marrow, from which they migrate in an immature form into tissues.6 They reside in vascularized connective tissues just beneath body epithelial surfaces, including the submucosal tissues of the gastrointestinal and respiratory tracts and the dermal layer that lies just below the surface of the skin. Mast cells play a central role in inflammation, and their activation and degranulation affect a great number of cells, including those involved in inflammation (e.g., vascular endothelial cells, smooth muscle cells, circulating platelets and leukocytes, nerves) and healing (e.g., fibroblasts), as well as glandular cells and cells of the immune system. Their activation contributes greatly to increased permeability of blood vessels and smooth muscle contraction (see Figure 7-11).
Lymphocytes constitute approximately 36% of the total leukocyte count and are the primary cells of the immune response (see Figure 27-3, D, and Chapter 8). Most lymphocytes transiently circulate in the blood and eventually reside in secondary lymphoid tissues as mature T cells, B cells, or plasma cells. The life span of the lymphocyte can be days, months, or years, depending on its type and subtype. (Lymphocyte function and dysfunction are described in detail in Unit III.)
Natural killer (NK) cells, which resemble large granular lymphocytes, kill some types of tumor cells (in vitro) and some virus-infected cells without being induced by previous exposure to these antigens (see Chapters 7 and 8). Hence they are named natural killer cells to differentiate them from T-cytotoxic cells, which are induced by antigen. NK cells also have the capacity to activate T cells and phagocytes and produce a variety of cytokines that can regulate immune responses. The predominant form of NK cells develops in the bone marrow and circulates in the blood, where it accounts for 5% to 10% of the circulating lymphoid pool, and is found mainly in the peripheral blood and spleen. NK cells develop independent of a thymus, although some NK precursors are found in the thymus and may develop into NKT cells that have markers of NK and T cells.
The monocytes and macrophages make up the mononuclear phagocyte system (MPS), formerly called the reticuloendothelial system (RES).7 Monocytes and macrophages are active phagocytes that participate in the immune and inflammatory responses. They also ingest dead or defective host cells, particularly blood cells.
Monocytes are the largest normal blood cell and have a horseshoe-shaped nucleus (see Figure 27-3, E). They are formed and released by the bone marrow into the bloodstream. Monocytes migrate into a variety of tissues and fully mature into tissue macrophages and myeloid dendritic cells (Table 27-3). Other monocytes may mature into macrophages in the circulation and migrate out of the vessels in response to infection or inflammation. Macrophages are generally larger and are more active as phagocytes than monocytes. Dendritic cells frequently extend projections (dendrites) into the tissue and take on a “neuron-like” appearance. The origin and turnover of many of the tissue macrophages are not precisely known. It seems clear that once monocytes leave the circulation, they do not return. They can survive many months or even years.
TABLE 27-3
NAME OF CELL | LOCATION |
Committed Stem Cells† | Bone marrow |
Monoblasts | Bone marrow |
Promonoblasts | Bone marrow |
Monocytes | Bone marrow and peripheral blood |
Macrophages | Tissue |
Kupffer cells | Liver macrophages |
Alveolar macrophages | Lung |
Histiocytes | Connective tissue |
Macrophages | Bone marrow |
Fixed and free macrophages | Spleen and lymph nodes |
Pleural and peritoneal macrophages Adipose macrophages | Serous cavities Adipose (fat) tissue |
Microglial cells | Nervous system |
Mesangial cells | Kidney |
Osteoclasts | Bone |
Langerhans cells | Skin |
Dendritic cells | Lymphoid tissue, lining of respiratory and gastrointestinal tracts |
∗Formerly called the reticuloendothelial system.
†Development of blood cells from stem cells in the marrow is described on this page and illustrated in Figure 27-10.
Modified from Kumar V et al: Robbins & Cotran pathologic basis of disease, ed 7, Philadelphia, 2005, Saunders.
The normal role of macrophages is to remove old and damaged cells and large-molecular substances from the blood. Cellular targets of macrophage phagocytosis include circulating senescent or damaged erythrocytes and platelets (removed primarily in spleen), dead neutrophils (in the circulation and at sites of inflammation), and cells undergoing apoptosis. Noncellular targets include antigen-antibody complexes, cellular debris, products of coagulation, and macromolecules (such as lipids and carbohydrates synthesized by the body as the result of faulty metabolism, as in storage diseases). Macrophages remove and kill contaminating microorganisms in the blood (mostly in the liver and spleen) and at sites of infection. Macrophages and, particularly, dendritic cells are the major “antigen-processing” and “antigen-presenting” cells that initiate immune responses (see Chapter 8). Macrophages initiate wound healing and tissue remodeling and if activated by cytokines from T cells secrete a large array of biologically active chemicals that if uncontrolled result in chronic inflammation and tissue injury (see Chapter 7). Osteoclasts are multinucleated macrophage-like cells specialized for the function of lacunar bone resorptions and remodeling in addition to phagocytosis.8
Platelets
Platelets (thrombocytes) are not true cells but irregularly-shaped cytoplasmic fragments that are essential for blood coagulation and control of bleeding.9 They are formed by fragmentation of very large (40 to 100 μm in diameter) cells known as megakaryocytes (Figure 27-4). They lack a nucleus and deoxyribonucleic acid (DNA), and are incapable of mitotic division. They do, however, contain cytoplasmic granules (i.e., dense granules, alpha granules) capable of releasing biochemical mediators when stimulated by injury to a blood vessel. The contents of the dense granules are generally proinflammatory (e.g., adenosine diphosphate [ADP], adenosine triphosphate [ATP], calcium, serotonin, and histamine).10 The alpha granules contain a mixture of coagulation factors, growth and angiogenic factors (e.g., platelet-derived growth factor [PDGF], vascular endothelial growth factor [VEGF], and basic fibroblast growth factor), and angiogenesis inhibitors (e.g., platelet factor 4, thrombospondin, and inhibitors of metalloproteinases). Depending upon the particular stimulus, platelets may selectively release promoters or inhibitors of angiogenesis.11 Activation also stimulates synthesis of arachidonic acid pathway products (e.g., thromboxane A2) (see Chapter 7).
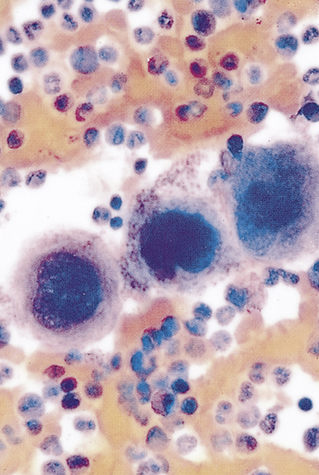
Note the large number of platelets (purple) surrounding the large megakaryocytes in the center. (From Miale JB: Laboratory medicine: hematology, ed 6, St Louis, 1982, Mosby.)
Lymphoid Organs
The lymphoid system is closely integrated with the circulatory system. The lymphoid organs, some of which are merely aggregations of lymphoid tissue, are classified as primary or secondary. The primary lymphoid organs are the thymus and the bone marrow. The secondary lymphoid organs consist of the spleen, lymph nodes, tonsils, and Peyer patches of the small intestine (see Figure 8-3). All of the lymphoid organs link the hematologic and immune systems in that they are sites of residence, proliferation, differentiation, or function of lymphocytes and mononuclear phagocytes (monocytes and macrophages).12 (The liver, which also has hematologic functions, is primarily a digestive organ and is described in Chapter 40.)
Spleen
The spleen is the largest of the secondary lymphoid organs. It is a site of fetal hematopoiesis; its mononuclear phagocytes filter and cleanse the blood; its lymphocytes mount an immune response to blood-borne microorganisms; and it serves as a blood reservoir (see Chapter 29).
The spleen is a concave, encapsulated organ that weighs approximately 150 g and is about the size of a fist. It is located in the left upper abdominal cavity, curved around a portion of the stomach (see Figure 8-3). Strands of connective tissue (trabeculae) extend from the capsule, dividing the spleen into compartments (Figure 27-5). The compartments contain masses of lymphoid tissue called splenic pulp. The spleen is interlaced with many blood vessels, some of which are capable of distending to store blood. Blood that circulates through the spleen comes from the splenic artery, which branches from the descending aorta and reenters the circulatory system through the splenic vein and into the portal vein.
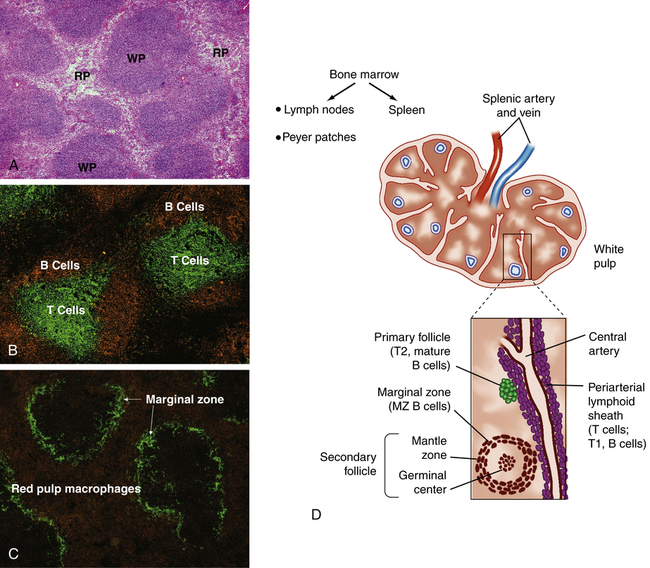
A, Spleen section stained with hematoxylin and eosin shows areas of densely packed cells, referred to as the white pulp (WP), separated by areas with more dispersed cell populations, referred to as the red pulp (RP). B, Spleen section that has been stained with fluorescently labeled antibodies specific for B cells (orange) and T cells (green) shows the distinct localization of B cells and T cells within the white pulp. C, Staining for macrophages (orange) and the splenic marginal zone (green) shows the density of macrophages and phagocytic cells in the red pulp and marginal zone. D, The spleen is enclosed in a capsule with the interior pulp divided into compartments by strands of connective tissue. The splenic pulp contains regions that are rich in lymphocytes (white pulp) and those containing erythrocytes (red pulp). The central arteries are frequently surrounded by a periarterial lymphoid sheath, primarily containing T cells, B cells, and macrophages, with adjacent lymphoid follicles. The secondary lymphoid follicles contain B cells that are proliferating in response to antigen. (A, B, and C from Mandell G, Bennett J, Dolin R: Mandell, Douglas, and Bennett’s principles and practice of infectious diseases, ed 7, Philadelphia, 2010, Churchill Livingstone; D from Hoffman R et al: Hematology: basic principles and practice, ed 6, Philadelphia, 2013, Churchill Livingstone.)
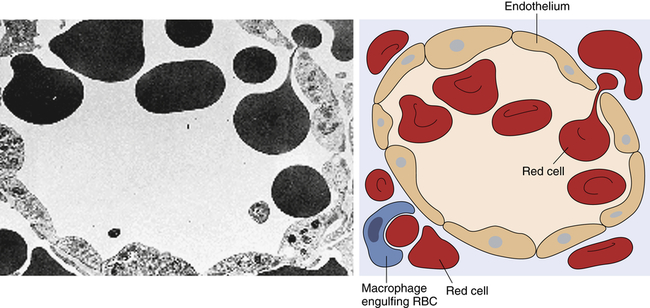
Transmission electron micrograph and schematic of erythrocytes in the process of squeezing from the red pulp cords into the sinus lumen. Note the degree of deformability required for red cells to pass through the wall of the sinus. (From Damjanov I, Linder J, editors: Anderson’s pathology, ed 10, St Louis, 1996, Mosby. Schematic from Kumar V, Fausto N, Abbas A: Robbins & Cotran pathologic basis of disease, ed 7, St Louis, 2005, Saunders.)
The portion of arterial blood that enters the spleen first encounters the white splenic pulp, which consists of masses of lymphoid tissue containing macrophages and lymphocytes, primarily T lymphocytes in proximity to the arterioles (see Figure 27-5, B and D). Cellular clumps (lymphoid follicles) are formed in the white pulp around the splenic arterioles. The lymphoid follicles consist primarily of B lymphocytes. These are the chief sites of immune function within the spleen. Here blood-borne antigens encounter lymphocytes, initiating the immune response and the conversion of lymphoid follicles into germinal centers (see Chapter 8).13
The endothelial lining of the venous sinuses is discontinuous (having gaps between endothelial cells) and therefore extremely permeable so that blood cells are allowed to exit the circulation (Figure 27-6). The red pulp contains a system of loosely interconnected resident macrophages that provide the principal site of splenic filtration. Because of the slow circulation in the sinuses, the macrophages easily phagocytose old, damaged, or dead blood cells of all kinds (but chiefly erythrocytes), microorganisms, macromolecules, and particles of debris. Hemoglobin from phagocytosed erythrocytes is catabolized, and heme (iron) is stored in the cytoplasm of the macrophages or released back into the blood (see Figure 27-17). The macrophages also can remove particulate inclusions containing denatured hemoglobin (Heinz bodies) from erythrocytes without harming the cells themselves. Blood that filters through the red pulp also finds its way into the venous sinuses and hence into the portal circulation.
Lymph Nodes
Structurally, lymph nodes are part of the lymphatic system. Lymphatic vessels collect interstitial fluid from the tissues and transport it, as lymph, through vessels of increasing size to the thoracic duct, which drains into the superior vena cava returning the lymph to the circulation. Lymph nodes are distributed throughout the body and provide filtration of the lymph during its journey through the lymphatics. Each lymph node is enclosed in a fibrous capsule, branches of which (trabeculae) extend inward to partition the node into several compartments (Figure 27-7). Reticular fibers of connective tissue divide the compartments into a meshwork throughout the lymph node. The node consists of outer (cortex) and inner (paracortex) cortical areas and an inner medulla. Lymph enters through multiple small afferent lymphatic vessels into the subcapsular sinus, just beneath the capsule; drains into the cortical sinuses to the medullary sinuses, from which the lymph is collected; and leaves the node by way of the efferent lymphatic vessel. Blood flows into the lymph nodes through the lymphatic artery, which ends in groups of postcapillary venules disturbed throughout the outer cortex. The blood is drained through the lymphatic vein.
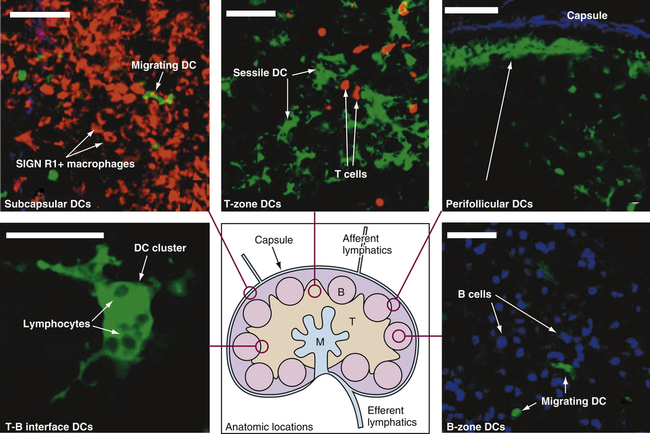
Drawing in lower center: Lymph enters via the afferent lymphatics and exits via the efferent lymphatics. T cells enter the lymph nodes via the high endothelial venules and exit via the medulla to the efferent lymphatics. B, B-cell follicles; M, medullary cords; T, T-cell zone. Other structures are labeled. Stained microscopic images: All images are linked to the drawing of the lymph node. DC, Dendritic cell. (All scale bars 50 μm.) (From Lindquist RL et al: Nat Immunol 5:1243–1250, 2004, by permission from Macmillan Publishers Ltd.)
Functionally, lymph nodes are part of the hematologic and immune systems and are the primary site for the first encounter between antigen and lymphocytes. Lymphocytes enter the lymph node from the blood through the postcapillary venules by means of diapedesis across the endothelial lining. B lymphocytes tend to migrate preferentially to nodes in the cortex and medulla, whereas T lymphocytes predominantly migrate to the paracortex (see Figure 27-7). Macrophages reside in the lymph node; help filter the lymph of debris, foreign substances, and microorganisms; and provide antigen-processing functions. The dendritic cells encounter and process antigens and microorganisms in other tissues, enter the lymph node through the afferent lymph vessels, and migrate throughout the nodes. The reticular network provides adhesive surfaces for trapping large numbers of phagocytes and lymphocytes and facilitating their organization into follicles or primary nodules. The presence of antigen, either removed from the lymph by macrophages or presented on the surface of dendritic cells, results in the production of secondary nodules containing germinal centers. In the germinal centers lymphocytes, particularly B cells, respond to antigenic stimulation by undergoing proliferation and further differentiation, including class-switch, into memory cells and plasma cells (see Chapter 8). Plasma cells migrate to the medullary cords. The B-lymphocyte proliferation in response to a great deal of antigen (e.g., during infection) may result in lymph node enlargement and tenderness (reactive lymph node).
Development of Blood Cells
Hematopoiesis
The typical human requires about 100 billion new blood cells per day. Blood cell production, termed hematopoiesis, is constantly ongoing, occurring in the liver and spleen of the fetus and only in bone marrow (medullary hematopoiesis) after birth (see Chapter 30). This process involves the biochemical stimulation of populations of relatively undifferentiated cells to undergo mitotic division (i.e., proliferation) and maturation (i.e., differentiation) into mature hematologic cells (Table 27-4). Although proliferation and differentiation are usually sequential, certain blood cells proliferate and differentiate simultaneously. Erythrocytes and granulocytes generally differentiate fully before entering the blood, but monocytes and lymphocytes continue to mature in the blood and in secondary lymphatic organs.
TABLE 27-4
HUMAN HEMATOPOIETIC GROWTH FACTORS (CYTOKINES, COLONY-STIMULATING FACTORS)
FACTOR | CELL ORIGIN | PRIMARY CELL STIMULATED |
M-CSF | Macrophage, lymphocyte, fibroblast, endothelial cell, osteoblast | Monocyte progenitor to monocyte |
GM-CSF | Macrophage, T cell, endothelial cell, fibroblast, mast cell | Common myeloid progenitor to granulocyte progenitor and monocyte progenitor |
G-CSF | Macrophage, fibroblast, endothelial cell | Granulocyte progenitor to neutrophil |
IL-2 | Th cell | T-cell progenitor to T cell |
IL-3 | T cell, monocyte/macrophage, stromal cell | Common myeloid progenitor to progenitors for megakaryocyte, erythroid, granulocyte, and monocyte series |
IL-4 | Th cell | B-cell progenitor to B cell |
IL-5 | Th cell, mast cell | Common myeloid progenitor to eosinophil |
IL-7 | Stromal cell, intestinal epithelium | Hematopoietic stem cell to common lymphoid progenitor Common lymphoid progenitor to progenitor B cell, pro NK cell, and progenitor T cell Progenitor T cell to T cell and progenitor B cell to B cell |
IL-11 | Stromal cell | Megakaryocyte progenitor to megakaryocyte |
IL-15 | Monocyte/macrophage | NK progenitor to NK cell |
Erythropoietin | Peritubular kidney cell and Kupffer cell | Common myeloid progenitor to erythrocyte progenitor Erythrocyte progenitor to erythrocyte |
Thrombopoietin | Liver, kidney, skeletal muscle | All cells in megakaryocyte lineage from common myeloid progenitor to platelet |
Stem cell factor (steel factor) | Stromal cell in bone marrow and many other cells | Hematopoietic progenitor to common myeloid progenitor Common myeloid progenitor to progenitors for megakaryocyte, erythroid, granulocyte, and monocyte series |
Various abnormalities in medullary hematopoiesis have been identified and are discussed in Chapter 28. Extramedullary hematopoiesis—blood cell production in tissues other than bone marrow—of apparently normal blood cells has been reported in the spleen, liver, and, less frequently, lymph nodes, adrenal glands, cartilage, adipose tissue, intrathoracic areas, and kidneys. In adults, however, extramedullary hematopoiesis is usually a sign of disease, occurring in pernicious anemia, sickle cell anemia, thalassemia, hemolytic disease of the newborn (erythroblastosis fetalis), hereditary spherocytosis, and certain leukemias.
Bone Marrow
Bone marrow is confined to the cavities of bone and is the primary site of residence of hematopoietic stem cells (Figure 27-8). Adults have two kinds of bone marrow: red, or active (hematopoietic), marrow (also called myeloid tissue) and yellow, or inactive, marrow. The large quantity of fat in inactive marrow gives its characteristic yellow color. Not all bones contain active marrow. In adults, active marrow is found primarily in the flat bones of the pelvis (34%), vertebrae (28%), cranium and mandible (13%), sternum and ribs (10%), and in the extreme proximal portions of the humerus and femur (4% to 8%). Inactive marrow predominates in cavities of other bones. (Bones are discussed further in Chapter 43.)
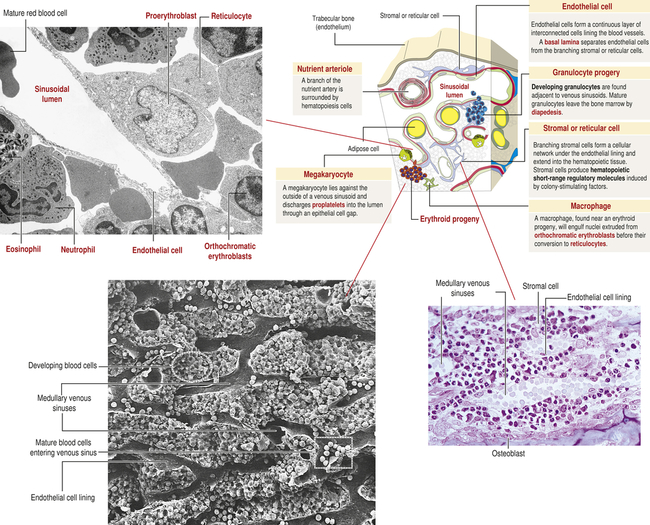
The hematologic compartment of the bone marrow consists of a variety of cellular microenvironments, called niches, that control differentiation of hematopoietic progenitor cells14 (Figure 27-9). The cellular composition of niches includes osteoclasts, osteoblasts, sinusoidal endothelial cells, fibroblasts, megakaryocytes, macrophages, and nerve cells.15 Osteoblasts are derived from fibroblasts and are responsible for construction of bone. Osteoclasts are multinucleate cells of monocytic origin that remodel bone by resorption. Both cells can produce cytokines that affect proliferation of hematopoietic cells. Recent studies have identified macrophage and megakaryocyte populations in bone marrow niches. The area is innervated through the sympathetic nervous system. At least two populations of stem cells are found in bone marrow niches. Mesenchymal stem cells (MSCs) are stromal cells that can differentiate into a variety of cells, including osteoblasts, adipocytes, and chondrocytes (produce cartilage). Hematopoietic stem cells (HSCs) are progenitors of all hematologic cells. Both populations of stem cells also undergo self-renewal in the bone marrow so that additional MSCs and HSCs are produced to replace those undergoing differentiation.
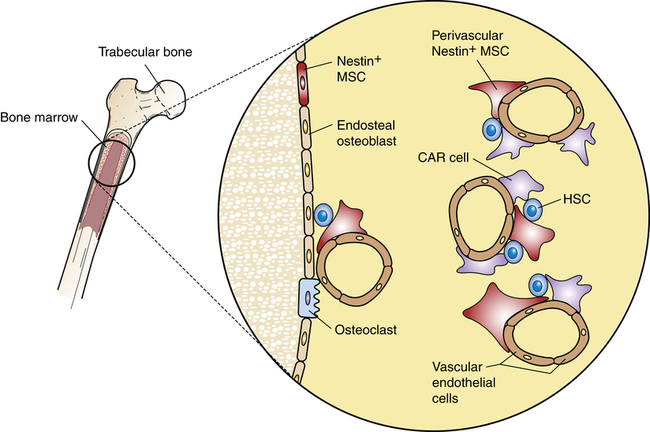
Stem cell niches are microenvironments where stem cells undergo hematopoiesis into all forms of blood cells. Stem cell niches retain and maintain adult resting hematopoietic stem cells (HSCs) and are activated after cell injury to promote cell renewal or differentiation to form new tissues. The fate of individual HSCs is determined by interactions (intercellular adherence, cytokines, chemokines) with specialized cells within the niches. Within osteoblastic niches the HSC interacts primarily with the osteoblasts and specialized mesenchynal stem cells (MSCs) that include nestin-expressing (Nestin+) MSCs and CXCL12-abundant reticular (CAR) cells. Within the vascular niches, the HSC interacts with vascular endothelial cells, Nestin+ MSC, and a more abundant population of CAR cells.
Two distinct types of niches have been identified: the osteoblastic (also called endosteal) niche and the vascular niche. The osteoblastic niche is centralized around osteoblasts, which line the surface of bone, whereas the vascular niche is organized around sinusoidal endothelial cells. In both niches, HSCs are affected by direct cell-to-cell signaling and by soluble mediators produced by cells within each niche. Each niche also contains two specialized cells derived from MSCs: CXCL12-abundant reticular (CAR) cells and nestin-expressing cells.16
CAR cells resemble reticular cells with long cellular processes. CAR cells closely interact with HSCs and provide important intercellular signaling through several HSC regulatory molecules, including CXCL12, SCF, VCAM-1, and ANG1. Chemokine ligand 12 (CXCL12) is a chemokine that reacts with the C-X-C chemokine receptor type 4 (CXCR4) on HSCs. The cytokine stem cell factor (SCF; also known as steel factor), which is expressed as a cell surface transmembrane protein or a soluble protein, reacts with the HSC KIT receptor (named stem cell growth factor receptor, proto-oncogene c-Kit, or CD117). Vascular cell adhesion molecule 1 (VCAM-1) mediates intercellular adhesion through its receptor integrin α4β1 (also called very late antigen 4, or VLA4). The secreted protein angiopoietin 1 (ANG1) reacts with a tyrosine kinase receptor. Nestin-expressing cells express large amounts of the intermediate filament protein nestin and, particularly, SCF and VCAM-1. Although both MSC-derived cells are present in the osteoblastic niche and vascular niche, the CAR cell is the predominant cell in the vascular niche.17
Each bone marrow niche affects HSCs differently. In the osteoblastic niche, HSCs are in direct contact with osteoblasts, CAR cells, and nestin-expressing cells. The effect is retention of HSCs in the bone marrow in a quiescent (dormant) state.18 HSCs that traffic to the vascular niche directly contact endothelial cells, as well as nestin-expressing cells and larger numbers of perivascular CAR cells. The cumulative signaling events induce HSC activation, proceeding to increased cellular division and hematopoietic differentiation. Other cells in the bone marrow, including macrophages and adipocytes, contribute to regulation of hematopoiesis.
Cell Differentiation
Within the bone marrow niches, each type of blood cell originates from common hematopoietic stem cells that proliferate and differentiate under control of a variety of cytokines and growth factors (Figure 27-10 and see Table 27-4). During this process some hematopoietic stem cells undergo alternative paths of differentiation into more differentiated stem cells that are committed to a particular line of blood cells.
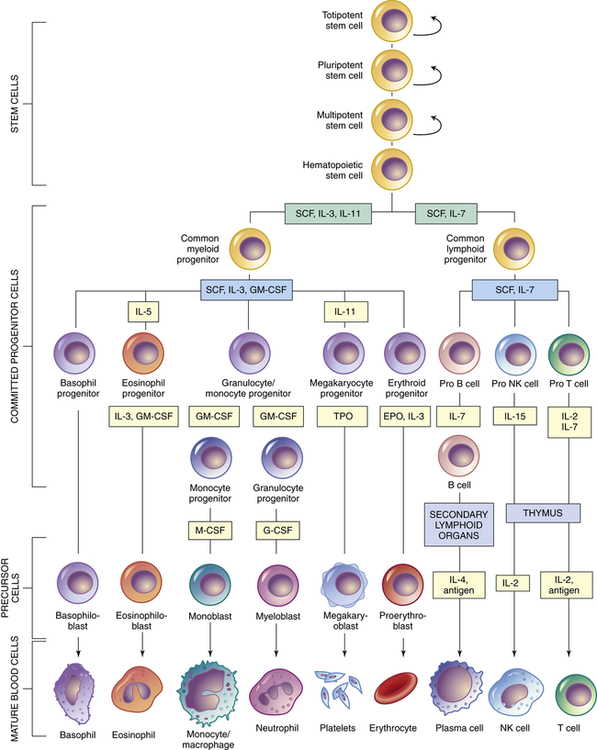
Curved arrows indicate proliferation and expansion of prehematopoietic stem cell populations. EPO, Erythropoietin; G-CSF, granulocyte colony-stimulating factor; GM-CSF, granulocyte-macrophage colony-stimulating factor; IL, interleukin; M-CSF, macrophage colony-stimulating factor; NK, natural killer; SCF, stem cell factor; TPO, thrombopoietin.
Hematopoietic stem cells represent just one stage in a process of progressive cellular differentiation. All humans originate from a single cell (the fertilized egg) that has the capacity to proliferate and eventually differentiate into the huge diversity of cells of the human body. After fertilization, the egg divides over a 5-day period to form a hollow ball (blastocyst) that implants on the uterus. Until about 3 days after fertilization, each cell (blastomere) is undifferentiated and retains the capacity to differentiate into any cell type. In the 5-day blastocyst, the outer layer cells have undergone differentiation and commitment to become the placenta. Cells of the inner cell mass (embryonic stem cells), however, continue to have unlimited differentiation potential (currently referred to as being pluripotent) and can grow into different kinds of tissue—blood, nerves, heart, bone, and so forth. After implantation, cells of the inner cell mass begin differentiation into other cell types. Differentiation is a multistep process and results in intermediate groups of stem cells (multipotent stem cells) with more limited, but still impressive, abilities to differentiate into many different types of cells (see Figure 27-10).19
Hematopoietic stem cells have partially differentiated.20 They have the capacity to differentiate easily into any of the hematologic cell populations but are very difficult to differentiate into other cell types, like nerve or muscle cells. The challenge of getting any partially committed multipotent stem cells to differentiate reliably involves coaxing them with identical chemical signals that the body uses naturally for differentiation. This is a daunting task with potentially astonishing clinical implications. For example, bone marrow might become the reservoir from which stem cells are harvested and then stimulated to produce nerve cells to help with the treatments of spinal cord injuries.
As with all stem cells, the hematopoietic stem cells are self-renewing (they have the ability to proliferate without further differentiation) so that a relatively constant population of stem cells is available. Some hematopoietic stem cells will continue differentiation into hematopoietic progenitor cells. Progenitor cells retain proliferative capacity but are committed to possible further differentiation into particular types of hematologic cells: lymphoid (lymphocytes, NK cells), granulocyte-monocyte (granulocytes, monocytes, macrophages), and megakaryocyte-erythroid (platelets, erythrocytes) progenitor cells (see Figure 27-10).
In addition to intercellular signaling events between HSCs and cells in the osteoblastic and vascular niches, several cytokines participate in hematopoiesis, particularly colony-stimulating factors (CSFs or hematopoietic growth factors), which stimulate the proliferation of progenitor cells and their progeny and initiate the maturation events necessary to produce fully mature cells (see Figure 27-10).21 Multiple cell types in the hematopoietic organs, including endothelial cells, fibroblasts, and lymphocytes, produce the necessary CSFs.
Hematopoiesis in the bone marrow occurs in two separate pools: the stem cell pool and the bone marrow pool (Figure 27-11). The stem cell pool is the product of self-renewal that maintains the number of pluripotent stem cells and partially committed progenitor cells. The bone marrow pool contains cells that are proliferating and maturing in preparation for release into the circulation and mature cells that are stored for later release into the peripheral blood. The peripheral blood also contains two pools of cells: those in the circulation and those stored around the walls of the blood vessels (often called the marginating storage pool). The marginating storage pool primarily consists of neutrophils that adhere to the endothelium in vessels where the blood flow is relatively slow. These cells can rapidly move into tissues and mucous membranes when needed in an inflammatory response.
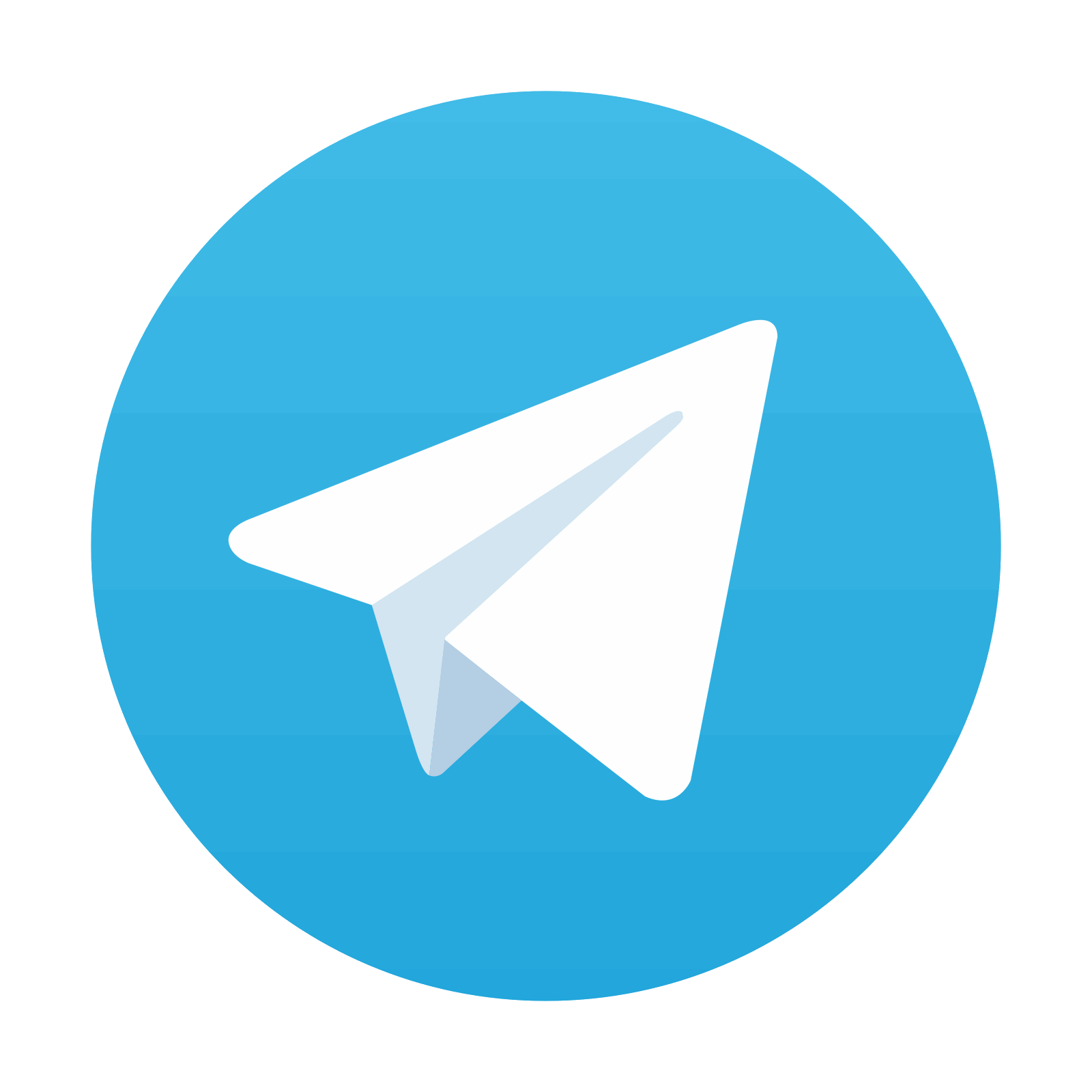
Stay updated, free articles. Join our Telegram channel

Full access? Get Clinical Tree
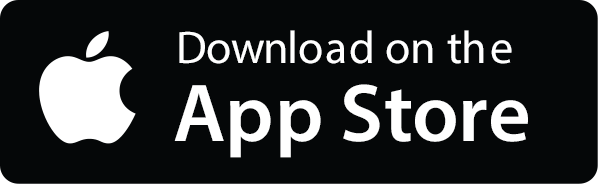
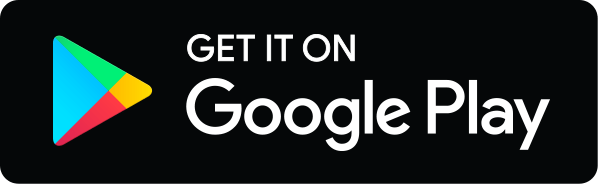