Chapter 40
Structure and Function of the Digestive System
Alexa K. Doig and Sue E. Huether
The Gastrointestinal Tract
The gastrointestinal tract (alimentary canal) consists of the mouth, esophagus, stomach, small intestine, large intestine, rectum, and anus (Figure 40-1). It carries out the following digestive processes:
2. Propulsion of food and wastes from the mouth to the anus
3. Secretion of mucus, water, and enzymes
4. Mechanical digestion of food particles
5. Chemical digestion of food particles
6. Absorption of digested food
Histologically, the gastrointestinal tract consists of four layers. From the inside out, they are the mucosa, submucosa, muscularis, and serosa or adventitia (esophagus only). These concentric layers vary in thickness, and each layer has sublayers (Figure 40-2). Neurons forming the enteric nervous system are located solely within the gastrointestinal tract and are controlled by local and autonomic nervous system stimuli. The enteric nervous system comprises three nerve plexuses located in different layers of the gastrointestinal walls. The submucosal plexus (Meissner plexus) is located in the muscularis mucosae, the myenteric plexus (Auerbach plexus) between the inner circular and outer longitudinal muscle layers in the muscularis, and the subserosal plexus just beneath the serosa. The enteric (intramural) plexus neurons regulate motility reflexes, blood flow, absorption, secretions, and immune response.1
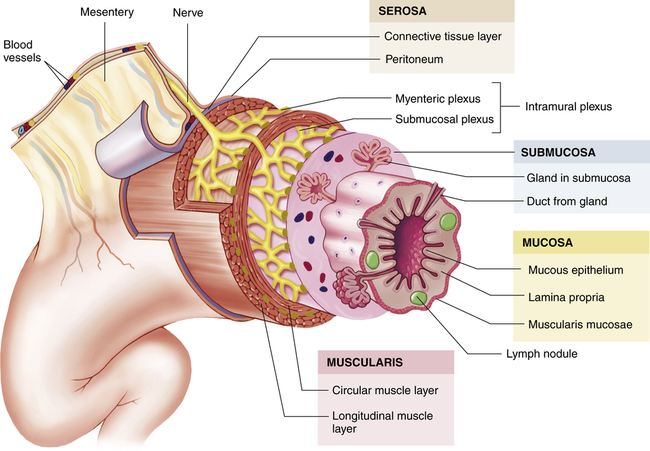
The wall of the GI tract is made up of four layers with a network of nerves between the layers. Shown here is a generalized diagram of a segment of the GI tract. Note that the serosa is continuous with a fold of serous membrane called a mesentery. Note also that digestive glands may empty their products into the lumen of the GI tract by way of ducts. (From Patton KT, Thibodeau GA: Anatomy & physiology, ed 8, St Louis, 2013, Mosby.)
Mouth and Esophagus
Salivation
The three pairs of salivary glands (the submandibular, sublingual, and parotid glands) (Figure 40-3) secrete about 1 L of saliva per day. Saliva consists mostly of water that contains varying amounts of mucus, sodium, bicarbonate, chloride, potassium, and salivary α-amylase (ptyalin), an enzyme that initiates carbohydrate digestion in the mouth and stomach.
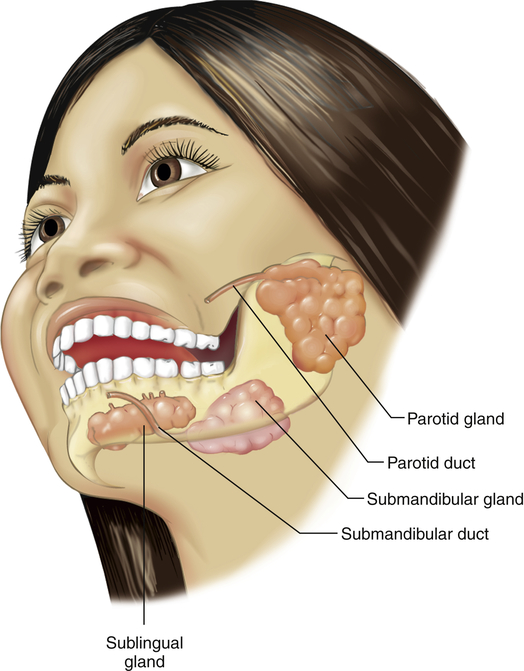
The sympathetic and parasympathetic divisions of the autonomic nervous system control salivation. Because cholinergic parasympathetic fibers stimulate the salivary glands, atropine (an anticholinergic agent) inhibits salivation and makes the mouth dry. β-Adrenergic stimulation from sympathetic fibers also increases salivary secretion. The salivary glands are not regulated by hormones, although hormones are found in saliva.1a
Swallowing
The esophagus is a hollow muscular tube approximately 25 cm long that conducts substances from the oropharynx to the stomach (see Figure 40-1). The pharynx and upper third of the esophagus contain striated muscle that is directly innervated by skeletal motor neurons that control swallowing. The middle third contains a mix of striated and smooth muscle, and the lower third is smooth muscle that is innervated by preganglionic cholinergic fibers from the vagus nerve. Peristalsis is stimulated when afferent fibers distributed along the length of the esophagus sense changes in wall tension caused by stretching as food passes. The greater the tension, the greater the intensity of esophageal contraction. Occasionally, intense contractions cause pain similar to “heartburn” or angina.
Each end of the esophagus is opened and closed by a sphincter. The upper esophageal sphincter (cricopharyngeal muscle) prevents entry of air into the esophagus during respiration.2 The lower esophageal sphincter (cardiac sphincter) prevents regurgitation from the stomach. The lower esophageal sphincter is located near the esophageal hiatus—the opening in the diaphragm where the esophagus ends at the stomach.3
Swallowing is a complex event mediated by the trigeminal nucleus, nucleus tractus solitarius, and reticular formation of the brainstem and also involves other brain regions, including the insula/claustrum and cerebellum.4
Peristalsis that immediately follows the oropharyngeal phase of swallowing is called primary peristalsis. If a bolus of food becomes stuck in the esophageal lumen, the distention of the esophageal wall stimulates secondary peristalsis, a wave of contraction and relaxation that is independent of voluntary swallowing. This is in response to stretch receptors that are stimulated by increased wall tension, causing an increase in impulses from the swallowing center of the brain.
When it is closed, the lower esophageal sphincter serves as a barrier between the stomach and esophagus. Cholinergic vagal stimulation and the digestive hormone gastrin increase sphincter tone. Nonadrenergic, noncholinergic vagal impulses relax the lower esophageal sphincter, as do the hormones progesterone, secretin, and glucagon. Relaxation during swallowing is mediated by the vagus nerve.5
Stomach
The stomach is a hollow muscular organ that stores food during eating, secretes digestive juices, mixes food with these juices, and propels partially digested food, called chyme, into the duodenum of the small intestine. The anatomy of the stomach is presented in Figure 40-4. Its major anatomic boundaries are the lower esophageal sphincter, where food passes through the cardiac orifice at the gastroduodenal junction into the stomach, and the pyloric sphincter, which relaxes as food is propelled into the duodenum. Functional areas of the stomach are the fundus (upper portion), body (middle portion), and antrum (lower portion).
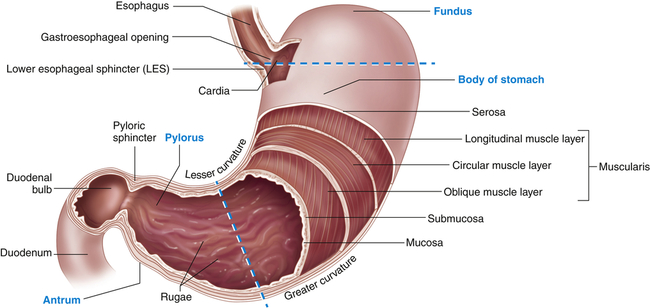
A portion of the anterior wall has been cut away to reveal the muscle layers of the stomach wall. Note that the mucosa lining the stomach forms folds called rugae. The dashed lines distinguish the fundus, body, and antrum of the stomach. (Modified from Patton KT, Thibodeau GA: Anatomy & physiology, ed 8, St Louis, 2013, Mosby.)
The stomach has three layers of smooth muscle: an outer, longitudinal layer; a middle, circular layer; and an inner, oblique layer (the most prominent) (see Figure 40-4). These layers become progressively thicker in the body and antrum, where food is mixed, churned, and pushed into the duodenum. The circular layer is most prominent and the oblique layer is the least complete; the longitudinal layer is absent on the anterior and posterior surfaces. The glandular epithelium is discussed in the section about secretory functions of the stomach (see p. 1398).
Gastric Motility
In its resting state the stomach is small and contains about 50 ml of fluid. There is little wall tension, and the muscle layers in the fundus contract very little. Swallowing causes the fundus to relax (receptive relaxation) to receive a bolus of food from the esophagus. Relaxation is coordinated by efferent, nonadrenergic, noncholinergic vagal fibers and is facilitated by gastrin and cholecystokinin, two polypeptide hormones secreted by the gastrointestinal mucosa. (The actions of digestive hormones are summarized in Table 40-1.) Food is stored in vertical or oblique layers as it arrives in the fundus, whereas fluids flow relatively quickly down to the antrum.
TABLE 40-1
SELECTED HORMONES AND NEUROTRANSMITTERS OF THE DIGESTIVE SYSTEM∗
SOURCE | HORMONE† | STIMULUS FOR SECRETION | ACTION |
Mucosa of the stomach | Gastrin | Presence of partially digested proteins in the stomach | Stimulates gastric glands to secrete hydrochloric acid and pepsinogen; growth of gastric mucosa; promotes gastric motility |
Histamine | Gastrin | Stimulates acid secretion | |
Somatostatin | Acid in the stomach | Inhibits acid and pepsinogen secretion and release of gastrin | |
Acetylcholine | Vagus and local nerves in stomach | Stimulates release of pepsinogen and acid secretion | |
Gastrin-releasing peptide (bombesin) | Vagus and local nerves in stomach | Stimulates gastrin and release of pepsinogen and acid secretion | |
Mucosa of the small intestine | Motilin | Presence of acid and fat in the duodenum | Increases gastrointestinal motility |
Secretin | Presence of chyme (acid, partially digested proteins, fats) in the duodenum | Stimulates pancreas to secrete alkaline pancreatic juice and liver to secrete bile; decreases gastrointestinal motility; inhibits gastrin and gastric acid secretion | |
Cholecystokinin | Presence of chyme (acid, partially digested proteins, fats) in the duodenum | Stimulates gallbladder to eject bile and pancreas to secrete alkaline fluid; decreases gastric motility; constricts pyloric sphincter; inhibits gastrin; delays gastric emptying | |
Enteroglucagon | Intraluminal fats and carbohydrates | Weakly inhibits gastric and pancreatic secretion and enhances insulin release, lipolysis, ketogenesis, and glycogenolysis; delays gastric emptying | |
Entero-oxyntin | Presence of chyme in duodenum | Delays gastric emptying | |
Gastric inhibitory peptide (GIP) | Fat and glucose in small intestine | Inhibits gastric secretion and gastric emptying, stimulates insulin release; inhibits intestinal motility | |
Peptide YY | Intraluminal fat and bile acids | Inhibits postprandial gastric acid and pancreatic secretion and delays gastric and small bowel emptying | |
Pancreatic polypeptide | Protein, fat, and glucose in small intestine | Decreases pancreatic and enzyme secretion | |
Vasoactive intestinal peptide | Intestinal mucosa and muscle | Relaxes intestinal smooth muscle, increases blood flow |
∗Data from Schubert ML, Peura DA: Gastroenterology 134(7):1842–1860, 2008; Wren AM, Bloom SR: Gastroenterology 132(6):2116–2130, 2007.
†Note: The digestive hormones are not secreted into the gastrointestinal lumen but rather into the bloodstream, in which they travel to target tissues. There are more than 30 peptide hormone genes expressed in the gastrointestinal tract and more than 100 hormonally active peptides.
Modified from Johnson LR: Gastrointestinal physiology, ed 7, St Louis, 2007, Mosby.
Gastric (stomach) motility increases with the initiation of peristaltic waves, which sweep over the body of the stomach toward the antrum. The rate of peristaltic contractions is approximately three per minute and is influenced by neural and hormonal activity. Gastrin and motilin (small intestine hormones) and the vagus nerve increase contraction by making the threshold potential of muscle fibers less negative. (The neural and biochemical mechanisms of muscle contraction are described in Chapter 43.) Sympathetic activity and secretin (another small intestine hormone) are inhibitory and make the threshold potential more negative. The rate of peristalsis is mediated by pacemaker cells that initiate a wave of depolarization (basic electrical rhythm), which moves from the upper part of the stomach to the pylorus.
The rate of gastric emptying (movement of gastric contents into the duodenum) depends on the volume, osmotic pressure, and chemical composition of the gastric contents. Larger volumes of food increase gastric pressure, peristalsis, and rate of emptying. Solids, fats, and nonisotonic solutions delay gastric emptying.6 (Osmotic pressure and tonicity are described in Chapters 1 and 3.) Products of fat digestion, which are formed in the duodenum by the action of bile from the liver and enzymes from the pancreas, stimulate the secretion of cholecystokinin.7 This hormone inhibits food intake, reduces gastric motility, and decreases gastric emptying so that fats are not emptied into the duodenum at a rate that exceeds the rate of bile and enzyme secretion. Osmoreceptors in the wall of the duodenum are sensitive to the osmotic pressure of duodenal contents. The arrival of hypertonic or hypotonic gastric contents activates the osmoreceptors, which delays gastric emptying to facilitate formation of an isosmotic duodenal environment. The rate at which acid enters the duodenum also influences gastric emptying. Secretions from the pancreas, liver, and duodenal mucosa neutralize gastric hydrochloric acid in the duodenum. The rate of emptying is adjusted to the duodenum’s ability to neutralize the incoming acidity. Peristaltic activity in the stomach is also affected by blood glucose levels. Low blood glucose levels stimulate the vagus nerve and gastric smooth muscles, increasing the rate of contraction.8
Gastric Secretion
Stimulated by eating, the stomach produces large volumes of gastric secretions. Specialized cells located throughout the gastric mucosa produce mucus, acid, enzymes, hormones, intrinsic factor, and gastroferrin. Intrinsic factor is necessary for the intestinal absorption of vitamin B12 and gastroferrin facilitates small intestinal absorption of iron. The hormones are secreted into the blood and travel to target tissues in the bloodstream. The other gastric secretions are released directly into the stomach lumen under neural and hormonal regulation.9 Mucus covering the entire mucosa, intercellular tight junctions, bicarbonate secretion, and submucosal acid sensors form a protective barrier against acid and proteolytic enzymes, which otherwise would damage the gastric lining.10
In the fundus and body of the stomach the gastric glands of the mucosa are the primary secretory units (Figure 40-5). Several of these glands (three to seven) empty into a common duct known as the gastric pit. The parietal cells (oxyntic cells) within the glands secrete hydrochloric acid, intrinsic factor, and gastroferrin. The chief cells within the glands secrete pepsinogen, an enzyme precursor that is readily converted to pepsin (a proteolytic enzyme) in the gastric fluid. The pyloric gland mucosa in the antrum synthesizes and releases the hormone gastrin from G cells. Enterochromaffin-like cells secrete histamine, and D cells secrete somatostatin.
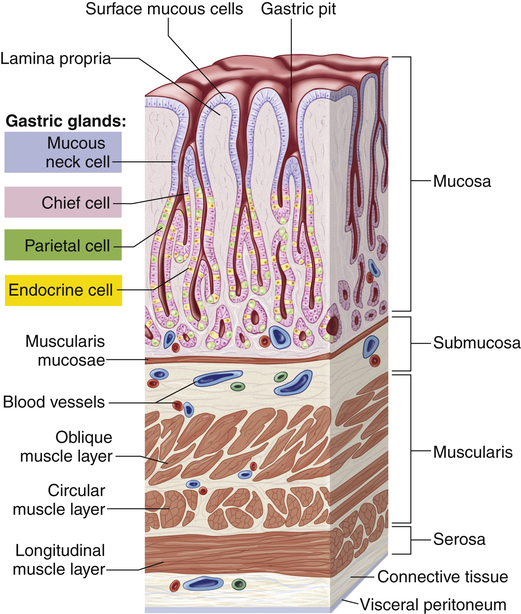
Gastric pits are depressions in the epithelial lining of the stomach. At the bottom of each pit is one or more tubular gastric glands. Chief cells produce the enzymes of gastric juice, and parietal cells produce stomach acid. (From Patton KT, Thibodeau GA: Anatomy & physiology, ed 8, St Louis, 2013, Mosby.)
The composition of gastric fluid depends on volume and flow rate (Figure 40-6). Potassium level remains relatively constant, but its concentration is greater in gastric secretions than in plasma. The rate of secretion varies with the time of day; lower in the morning and higher in the afternoon and evening. Loss of gastric secretions through vomiting, drainage, or suction may decrease body stores of sodium, potassium, hydrogen, and chloride.
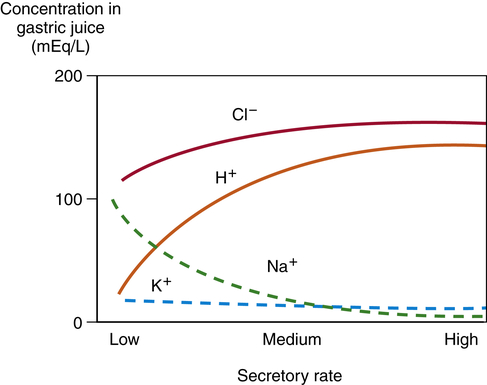
Sodium (Na+) concentration is lower in the gastric secretions than in the plasma, whereas hydrogen (H+), potassium (K+), and chloride (Cl−) concentrations are higher. Gastric secretions are close to isotonic. Secretory rate increases during the cephalic and gastric phases of digestion.
Acid
The major functions of gastric hydrochloric acid are to dissolve food fibers, act as a bactericide against swallowed organisms, and convert pepsinogen to pepsin. The production of acid by the parietal cells requires the transport of hydrogen and chloride from the parietal cells to the stomach lumen. Acid is formed in the parietal cells, primarily through the hydrolysis of water (Figure 40-7). At a high rate of gastric secretion, bicarbonate moves into the plasma, producing an “alkaline tide” in the venous blood, which also may result in a more alkaline urine.11
Acid secretion by parietal cells is stimulated by acetylcholine (ACh) (a neurotransmitter), gastrin (a hormone), and histamine (a biochemical mediator). The vagus nerve releases ACh and stimulates the secretion of histamine and gastrin-releasing peptide (GRP), which stimulates release of gastrin.12 Histamine secretion is also stimulated by gastrin. Histamine is stored in enterochromaffin cells (mast cells; see Chapter 7) in the gastric mucosa. Histamine receptors in the gastric mucosa are H2 receptors (unlike those in the bronchial mucosa, which are H1 receptors). Gastric lipase is produced by glands in the fundus of the stomach and is most effective in an acidic environment. Caffeine stimulates acid secretion, as does calcium. Prostaglandins, enterogastrones (such as gastric inhibitory peptide), somatostatin, and secretin inhibit acid secretion.13
Mucus
The gastric mucosa is protected from the digestive actions of acid and pepsin by a coating of mucus called the mucosal barrier. Gastric mucosal blood flow is important to maintaining mucosal barrier function.14
Intrinsic factor (IF), a mucoprotein produced by parietal cells, combines with vitamin B12 in the stomach. It is required for the absorption of vitamin B12 by the ileum. Atrophic gastritis and failure to absorb vitamin B12 result in pernicious anemia (see Chapter 28).
Phases of Gastric Secretion
The secretion of gastric juice is influenced by numerous stimuli that together facilitate the process of digestion. The phases of gastric secretion are the cephalic phase, gastric phase, and intestinal phase (Figure 40-8).
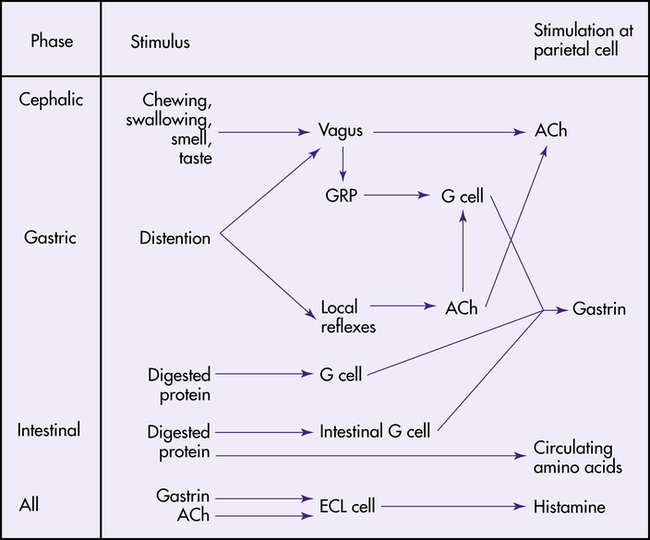
ACh, Acetylcholine; ECL, enterochromaffin-like cell; GRP, gastrin-releasing peptide. (From Johnson LR: Gastrointestinal physiology, ed 7, St Louis, 2007, Mosby.)
Cephalic Phase
The anticipatory and sensory experiences of smelling, seeing, tasting, chewing, and swallowing food contribute to the cephalic phase of secretion.15 The cephalic phase of gastric secretion is mediated by the vagus nerve through the myenteric plexus. Acetylcholine stimulates the parietal and chief cells to secrete acid and pepsinogen, respectively. The G cells in the antrum release gastrin, which circulates through the bloodstream to the gastric glands and stimulates acid and pepsinogen secretion.
Small Intestine
The small intestine is about 5 to 6 m long and is functionally divided into three segments: the duodenum, jejunum, and ileum (Figure 40-9). The duodenum begins at the pylorus and ends where it joins the jejunum at a suspensory ligament called the Treitz ligament. The end of the jejunum and beginning of the ileum are not distinguished by an anatomic marker. These structures are not grossly different, but the jejunum has a slightly larger lumen. The ileocecal valve (sphincter) controls the flow of digested material from the ileum into the large intestine and prevents reflux into the small intestine.
The smooth muscles of the small intestine are arranged in two layers: a longitudinal, outer layer; and a thicker, inner circular layer (see Figure 40-9). Circular folds of the small intestine mucosa slow the passage of food, thereby providing more time for digestion and absorption. The folds are most numerous and prominent in the jejunum and upper ileum.
Absorption occurs through villi, which cover the circular folds and are the functional units of the intestine (see Figure 40-9). A villus is composed of absorptive columnar epithelial cells (enterocytes) and mucus-secreting goblet cells of the mucosa. Each villus secretes some of the enzymes necessary for digestion and absorbs nutrients. Near the surface, columnar cells closely adhere to each other at sites called tight junctions. Water and electrolytes are absorbed through these intercellular spaces. The surface of each columnar epithelial cell on the villus contains tiny projections called microvilli (see Figure 40-9). Together the microvilli create a mucosal surface known as the brush border. The villi and microvilli greatly increase the surface area available for absorption. Coating the brush border is an “unstirred” layer of fluid that is important for the absorption of substances other than water and electrolytes. The lamina propria (a connective tissue layer of the mucosa) lies beneath the epithelial cells of the villi and contains lymphocytes; plasma cells, which produce immunoglobulins; and macrophages.
Central arterioles ascend within each villus and branch into a capillary array that extends around the base of the columnar cells and cascades down to the venules that lead to the hepatic portal circulation. The opposing ascending and descending blood flow provides a countercurrent exchange system for absorbed substances and blood gases. A central lacteal, or lymphatic capillary, is also contained within each villus and is important for the absorption and transport of fat molecules. Contents of the lacteals flow to regional nodes and channels that eventually drain into the thoracic duct16 (see Figure 40-9).
Between the bases of the villi are the crypts of Lieberkühn (intestinal glands), which extend to the submucosal layer. Undifferentiated (stem cells) and secretory cells and Paneth epithelial cells are located here. The stem cells are precursors of columnar epithelial and goblet cells. These premature cells produce alkaline fluids containing electrolytes, mucus, and water. These cells arise from the base of the crypt and move toward the tip of the villus, maturing in shape and function as they progress. After becoming columnar cells and completing their migration to the tip of the villus, they function for a few days and then are shed into the intestinal lumen and digested. The entire epithelial population is replaced about every 4 to 7 days. Many factors can influence this process of cellular proliferation. Starvation, vitamin B12 deficiency, and cytotoxic drugs or irradiation suppress cell division and shorten the villi. The decreased absorption that results can cause diarrhea and malnutrition. Nutrient intake and intestinal resection stimulate cell production. The Paneth cells located at the base of the crypts produce defensins and other antibiotic peptides and proteins.17 Other secretory cells produce digestive enzymes.
Intestinal Digestion and Absorption
The process of intestinal digestion is initiated in the stomach by the actions of gastric hydrochloric acid and pepsin, which break down food fibers and proteins. The chyme that passes into the duodenum is a liquid that contains small particles of undigested food. Digestion is continued in the proximal portion of the small intestine by the action of pancreatic enzymes, intestinal brush-border enzymes, and bile salts (Box 40-1). Here carbohydrates are broken down to monosaccharides and disaccharides; proteins are degraded further to amino acids and peptides; and fats are emulsified and reduced to fatty acids and monoglycerides (Figure 40-10). These nutrients, along with water, vitamins, and electrolytes, are absorbed across the intestinal mucosa and into the blood by active transport, diffusion, or facilitated diffusion. Products of carbohydrate and protein breakdown move into villus capillaries and then to the liver through the hepatic portal vein. Digested fats move into the lacteals and eventually reach the liver through the systemic circulation. Intestinal motility exposes nutrients to a large mucosal surface area by mixing chyme and moving it through the lumen. Different segments of the gastrointestinal tract absorb different nutrients. Digestion and absorption of all major nutrients occur in the small intestine. Sites of absorption are shown in Figure 40-11.
Water and Electrolyte Transport by the Small Intestine
The epithelial cell membranes of the small intestine are formed of lipids and therefore are hydrophobic, or tend to repel water. (The properties of cell membranes are described in Chapter 1.) Therefore, water and electrolytes are transported in both directions (toward the capillary blood or toward the intestinal lumen) through the tight junctions and intercellular spaces rather than across cell membranes. Water diffuses passively across hydrostatic pressure and osmotic gradients established by the active transport of sodium and other substances. Approximately 85% to 90% of the water that enters the gastrointestinal tract each day is absorbed in the small intestine. The remaining water and electrolytes are absorbed at a constant rate in the large intestine.18 Sodium passes through the tight junctions and is actively transported across cell membranes. Sodium and glucose share a common active transport carrier (sodium-glucose ligand transporter1 [SGLT1]) so that sodium absorption is enhanced by glucose transport18a (Figure 40-12). Potassium moves passively across the tight junctions with changes in the electrochemical gradient. Chloride is actively secreted throughout the large and small intestines.
Carbohydrates
Carbohydrate (starch, table sugar [sucrose], milk sugar [lactose], cereal sugar [maltose]) accounts for at least 50% of the American diet. Because only monosaccharides (ribose, galactose, glucose, fructose) are absorbed by the intestinal mucosa, the complex carbohydrates (polysaccharides and oligosaccharides) must be hydrolyzed to their simplest form (see Figure 40-10). Salivary and pancreatic amylases break down starches to oligosaccharides by splitting α-1,4-glucosidic linkages of long-chain molecules. The major disaccharides are sucrose (glucose-fructose), maltose (glucose-glucose), and lactose (glucose-galactose). Approximately half of starch hydrolysis occurs in the stomach and about half in the duodenum. In the small intestine, disaccharides are hydrolyzed by brush-border enzymes (sucrase, maltase, and lactase) to their respective monosaccharides.
The sugars are absorbed primarily in the duodenum and upper jejunum. The monosaccharides pass through the unstirred layer by diffusion. At the cell membrane, glucose and galactose are actively transported with a sodium carrier (SGLT1) and fructose absorption is facilitated by glucose transporter 5 (GLUT5) and GLUT7.19 Transport of all three monosaccharides from the cytosol to the bloodstream is facilitated by GLUT220 (see Figure 40-12). Insulin facilitates glucose transport into fat and muscle cells via glucose transporter 4 (GLUT4).
Proteins
The site of digestion of protein depends on the source of the protein. For example, casein from bovine milk precipitates in the stomach and is digested by gastric pepsin and acid, whereas the soluble proteins whey and soy pass rapidly through the stomach and are digested by pancreatic enzymes.21 Major protein hydrolysis is accomplished in the small intestine by the pancreatic enzymes trypsin, chymotrypsin, and carboxypeptidase (see Figure 40-10). Trypsin and chymotrypsin (endopeptidase) hydrolyze the interior bonds of the large molecules, and carboxypeptidases cleave the end amino acids (exopeptidase). Hydrolysis of proteins is also carried out by the brush-border enzymes and enzymes in the epithelial cytosol (intracellular fluid). The brush-border enzymes hydrolyze the large oligopeptides (proteins composed of three to six amino acids) into smaller peptides, which can cross cell membranes. Enzymes in the cytosol then break them down to amino acids. Amino acids are actively transported from the cytosol into the bloodstream by a sodium-dependent carrier in the basolateral membrane. There also are free amino acids that can be absorbed directly from the intestinal lumen using a membrane transport protein. Like the sugars, proteins are absorbed primarily in the proximal area of the small intestine.
Fats
Approximately 90 to 100 g of fat is consumed daily by the average American. Fat is an important source of calories and is a primary structural component of cell membranes and organelles. Sources of dietary fat are reviewed in Box 40-2. Although triglycerides are the major dietary lipids, cholesterol, phospholipids, and fat-soluble vitamins also have nutritional importance. The digestion and absorption of fat occur in four phases: (1) emulsification and lipolysis, (2) micelle formation, (3) fat absorption, and (4) resynthesis of triglycerides and phospholipids.
The mechanical action of the stomach and small intestine disperses the triglyceride droplets into small particles. Emulsification is the process by which emulsifying agents (fatty acids, monoglycerides, lecithin, cholesterol, protein, bile salts) in the small intestinal lumen cover the small fat particles and prevent them from re-forming into fat droplets. Emulsified fat is then ready for lipolysis (lipid hydrolysis) by pancreatic lipase, phospholipase, and hydrolase. Lipase breaks down triglycerides to diglycerides, monoglycerides, free fatty acids, and glycerol (see Figure 40-10). The action of lipase requires the presence of colipase, a pancreatic enzyme that allows lipase to penetrate the triglyceride molecule. Phospholipase cleaves fatty acids from phospholipids, and cholesterol esterase breaks cholesterol esters into fatty acids and glycerol.
The products of lipid hydrolysis must be made water soluble if they are to be absorbed efficiently from the intestinal lumen. This is accomplished by the formation of water-soluble molecules known as micelles (Figure 40-13). Micelles are formed of bile salts (see p. 1411), the products of fat hydrolysis, fat-soluble vitamins, and cholesterol. The fats form the core of the micelle, and the polar bile salts form an outer shell, with the hydrophobic (“water-hating”) side facing the interior and the hydrophilic (“water-loving”) side facing the aqueous (water-like) content of the intestinal lumen. Because the unstirred layer of the brush border is aqueous, the micelles readily diffuse through it. The micelles maintain the fat molecules in the dissolved or solubilized form, which allows them to move more rapidly from the micelle toward the absorbing surface of the intestinal epithelium. The fat products of the micelle then readily diffuse through the epithelial cell membrane, while the bile salts remain in the lumen and proceed to the ileum, where they are absorbed into the circulation and returned to the liver via the enterohepatic circulation (Figure 40-14 and Figure 40-20, p. 1411). Almost all of the bile salts are recycled in this way.
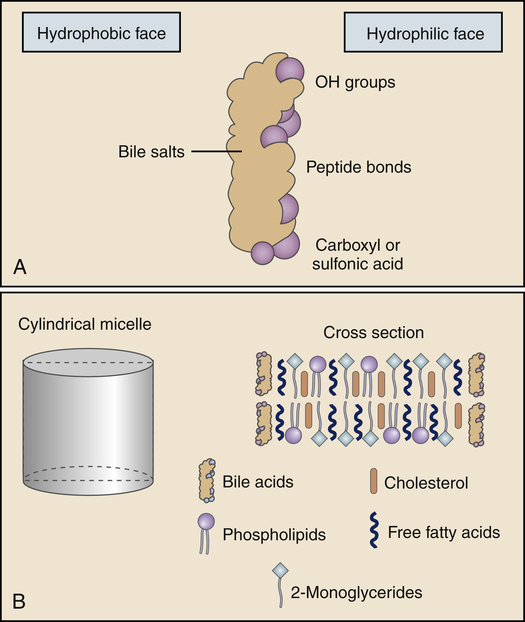
A, A bile salt molecule in solution. The molecule is amphipathic in that it has a hydrophilic face and a hydrophobic face. The amphipathic structure is key in the ability of the bile salts to emulsify lipids and form micelles. B, A model of the structure of a bile salt–lipid mixed micelle, an emulsified fat. (From Levy MN, Koeppen BM, Stanton BA: Principles of physiology, ed 4, St Louis, 2006, Mosby.)
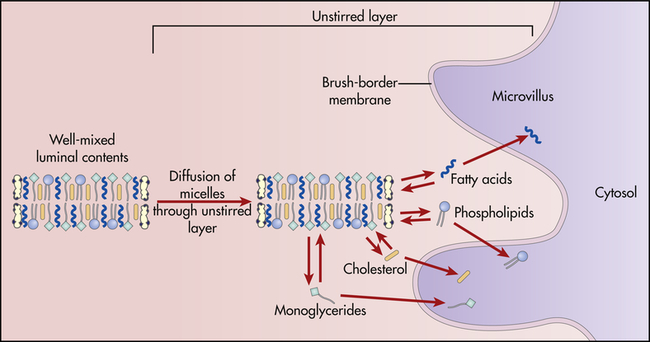
Micelles of bile salts and products of lipid digestion diffuse through the unstirred layer and among the microvilli. As digestive products are absorbed from free solution by epithelial cells of the villi, more digestive products dissociate from the micelles. (From Levy MN, Koeppen BM, Stanton BA: Principles of physiology, ed 4, St Louis, 2006, Mosby.)
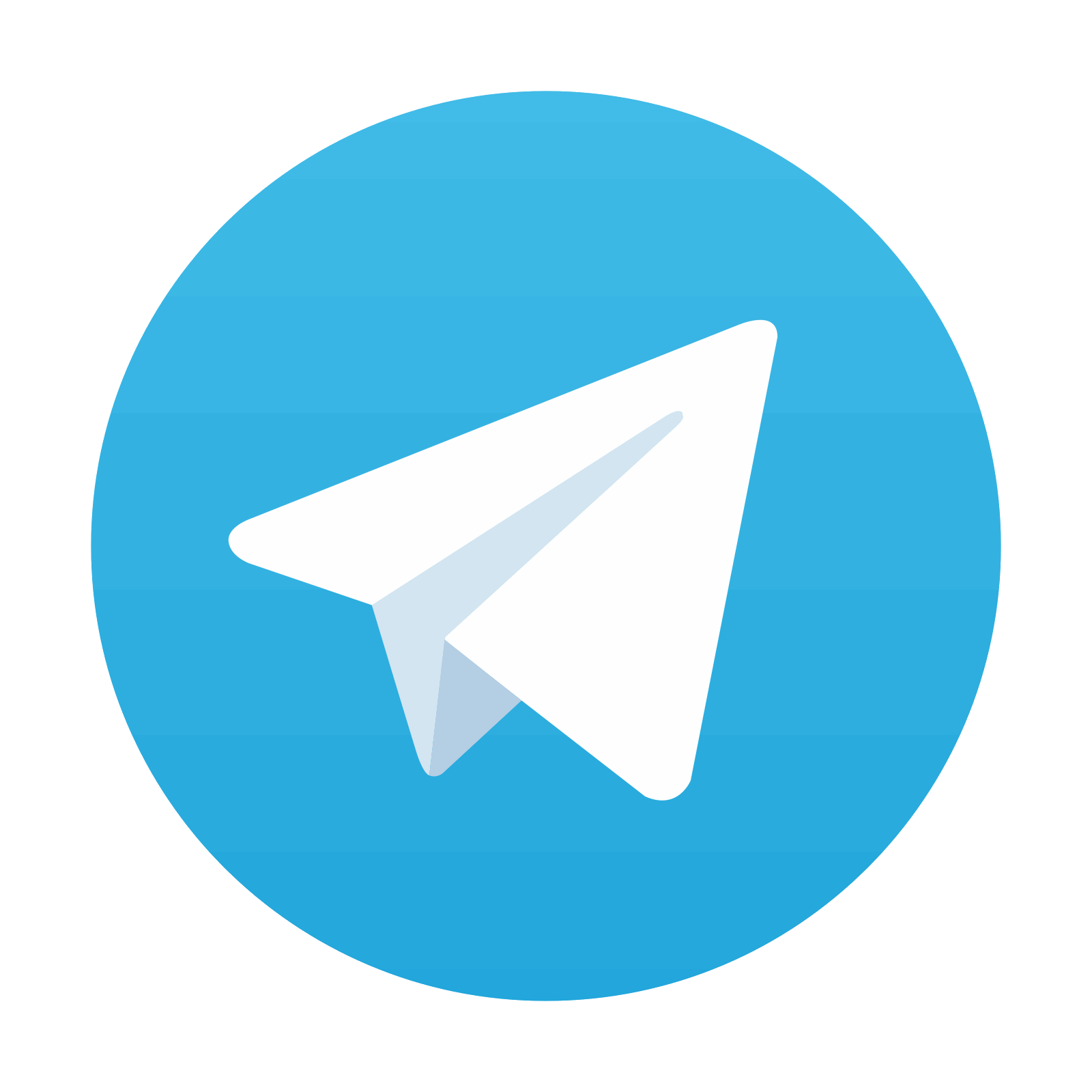
Stay updated, free articles. Join our Telegram channel

Full access? Get Clinical Tree
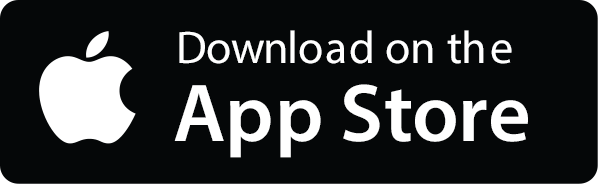
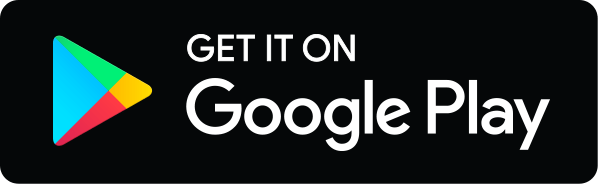