1 Introduction
Parenteral drug delivery systems and many medicinal products, such as dressings and sutures, must be sterile in order to avoid the possibilities of microbial degradation or infection occurring as a result of their use. Sterility is also important for any material or instrument likely to contact broken skin or internal organs. Although pathogenic bacteria, fungi or viruses pose the most obvious danger to a patient, it should be also realized that micro-organisms usually regarded as non-pathogenic and which inadvertently gain access to body cavities in sufficient numbers may cause a severe, possibly fatal infection. Consequently, injections, ophthalmic preparations, irrigation fluids, dialysis solutions, sutures and ligatures, implants, and certain surgical dressings, as well as instruments necessary for their use or administration, must be presented in a sterile condition.
Although there is always a chance of an idiosyncratic reaction between a medicine and a patient caused by sensitivity, allergic reaction or unwanted side effects, for sterile products there is the added requirement that they must be free of viable microorganisms. This consequently means the product should be manufactured in a manner that reduces to the lowest likelihood the risk of microbial contamination. Thus a sterile product should not contain viable bacteria, yeasts or fungi, nor other microorganisms such as rickettsiae, mycoplasmas, protozoa or viruses. The absence of prion particles is also desirable but difficult to demonstrate (see Chapter 2). Sterilization processes concentrate on the destruction or removal of microorganisms. Each process is designed to remove the most problematic microorganism (i.e. the smallest bacteria in filtration or the most heat-resistant bacterial spores in heat sterilization processes) on the basis that, once a sterilization process has been chosen, elimination of the most problematic species will have led to the elimination of all less resistant microorganisms.
The principles behind the sterilization processes are described in Chapter 21. The choice of method is determined largely by the ability of the formulation and container to withstand the physical stresses applied during the sterilization process. All products intended for sterilization should be manufactured under clean conditions and therefore will be of low microbial content (bioburden) prior to sterilization. Under these conditions, the sterilization process will not be overtaxed and will generally be within the safety limits needed to provide the required level of sterility assurance (Chapter 21). The next section emphasizes parenteral products, but the practices described apply to many other types of sterile product.
The most obviously recognized sterile pharmaceutical preparations are injections. These vary from very small-volume antigenic products to large-volume, total parenteral nutrition products. Other sterile products include ophthalmic preparations, creams and dusting powders. This section describes their formulation and packaging and the constraints imposed by sterilization on stability, formulation and packaging of some of the more common sterile products.
2.1 Injections
Injections may be aqueous solutions, oily solutions (because of poor aqueous solubility or the necessity for a prolongation of drug activity), aqueous suspensions or oily suspensions. They may be aseptically produced or terminally sterilized in their final containers (Chapter 23). Those drugs that are unstable in solution may be presented as a freeze-dried (lyophilized) powder. The choice of final packaging should not determine the method of sterilization.
2.1.1 Formulation philosophy
An injection must be manufactured under conditions that result in a product containing the minimum possible levels of particles and pyrogenic substances (section 4.4). Its formulation and packaging must maintain physical and chemical stability throughout the production processes, the intended shelf life and during administration. To achieve this, excipients such as buffers and antioxidants may be required to ensure chemical stability, and solubilizers, such as propylene glycol or polysorbates, may be necessary for drugs with poor aqueous solubility to maintain the drug in solution. Table 22.1 lists some chemical constituents of common injections and ophthalmic preparations.
Table 22.1 Some examples of excipients used in formulations and reasons for their inclusion
Product | Excipients | Reason for inclusion |
Diazepam injection | Ethanol | Cosolvent |
Propylene glycol | Cosolvent | |
Insulin isophane injection (Humalin, Eli-Lilly) | m-Cresol | Preservative |
Glycerol | Tonicity modifier | |
Phenol | Preservative | |
Protamine sulphate | Forms insulin complex | |
Dibasic sodium phosphate | Buffer | |
Zinc oxide | Adjusts zinc content | |
Hydrochloric acid | pH adjustment | |
Sodium hydroxide | pH adjustment | |
Promethazine hydrochloride injection USP | Disodium edetate | Chelating agent |
Sodium metabisulphite | Antioxidant | |
Phenol | Preservative | |
Sodium acetate | Buffer | |
Acetic acid | Buffer | |
Minims chloramphenicol eye drops | Borax | Buffer |
Boric acid | Buffer | |
Minims prednisolone sodium phosphate eye drops | Disodium edetate | Chelating agent |
Sodium chloride | Tonicity adjustment | |
Sodium dihydrogen phosphate | Buffer | |
Sodium hydroxide | pH adjustment |
Many injections are formulated as aqueous solutions, with Water for Injections (see Chapter 23) as the vehicle. Their formulation depends on several factors including the aqueous solubility of the active ingredient, the dose, its thermal stability, the route of administration, and whether the product is to be offered as a multiple-dose product (i.e. with doses removed on different occasions) or as a single-dose form (as the term suggests, only one dose per container). Most injections are prepared in single-dose form but this is mandatory for certain routes, e.g. spinal injections where the intrathecal route is used, and large-volume intravenous infusions. Multiple-dose injections may require the inclusion of a suitable preservative to prevent contamination following the removal of each dose. Injections used for several routes, including the intrathecal and intracardiac routes, must not contain a preservative because of potential long-term damage to the patient. A review of the preservatives used in parenteral products has been given by Meyer & Shi (2009).
Some types of injections must be isotonic with blood serum. This applies particularly to large-volume intravenous infusions if at all possible; hypotonic solutions may cause lysis of red blood corpuscles and thus must not be used for this purpose. Conversely, hypertonic solutions can be employed; these induce shrinkage, but not lysis, of red cells, which recover their shape later. Intraspinal injections must also be isotonic to reduce pain at the site of injection; so should intramuscular and subcutaneous injections. Adjustment to isotonicity can be determined from either the depression of freezing point or from sodium chloride equivalents. The depression of the freezing point depends on the number of dissolved particles (molecules or ions) present in a solution. The equation:
where W is the percentage (w/v) of adjusting substance, a is the freezing point of unadjusted solution and b is the depression of the freezing point of water induced by 1% w/v of adjusting substance, allows the determination of how much adjusting substance is required to produce isotonicity with blood plasma.
Alternatively the sodium chloride equivalent, which is produced by dividing the value for the depression of freezing point produced by a solution of the substance by the corresponding value of a solution of sodium chloride of the same strength, may be used. Fuller details of each method may be found in the Pharmaceutical Codex (1994).
2.1.2 Intravenous infusions
Intravenous infusions consist of large-volume injections or drips (500 ml or more) that are infused at various rates (50–500 ml/h) into the venous system. They are generally sterilized in an autoclave. Examples include isotonic solutions of sodium chloride or glucose that are used to maintain fluid and electrolyte balance, for replacement of extracellular body fluids (e.g. after surgery or prolonged periods of fluid loss), as a supplementary energy source (1 L of 5% w/v glucose yields 714 kJ of energy) or as a vehicle for drugs. Other important examples are blood products, which are collected and processed in sterile containers, and plasma substitutes, e.g. dextrans and degraded gelatin. Dextrans are glucose polymers in which the glucose monomers are joined by 1–6-α links; they are produced by certain bacteria of the genus Leuconostoc, e.g. Leuconostoc mesenteroides.
2.1.2.2 Intravenous a dditives
A common hospital practice is to add drugs to infusions immediately before administration. Regularly used additives include potassium chloride, lidocaine (lignocaine), heparin, certain vitamins and antibiotics. Potentially this can be a hazardous practice. For instance, the drug may precipitate in the infusion fluid because of the pH (e.g. amphotericin) or the presence of calcium salts (e.g. thiopentone); the drug may degrade rapidly (e.g. ampicillin in 5% w/v glucose); multiple additions may lead to precipitation of one or both of the drugs or to accelerated degradation; and finally, drug loss may occur because of sorption by the container. For instance, insulin is adsorbed by glass or by polyvinyl chloride (PVC); glyceryl trinitrite and diazepam are absorbed by PVC. Apart from these problems, if the addition is not carried out under strict aseptic conditions the fluid can become contaminated with microorganisms during the procedure. Thus any addition should be made in a laminar-flow workstation or isolator, and the fluid should, ideally, be administered within 24 hours of preparation.
Another approach to the problem of providing an intravenous drug additive service is to add the drug to a small volume (50–100 ml) infusion in a collapsible plastic container and store the preparation at −20°C in a freezer. The infusion can be removed when required and thawed rapidly in a microwave oven. Many antibiotics are stable for several months when stored in minibags at −20°C and are unaffected by the thawing process. Other antibiotics, e.g. ampicillin, degrade even when frozen.
2.1.2.2 Total parenteral nutrition
Total parenteral nutrition (TPN) is the use of mixtures of amino acids, vitamins, electrolytes, trace elements and an energy source (glucose and fat) in the long-term feeding of patients who are unconscious or unable to take food. All or most of the ingredients to feed a patient for 1 day are combined aseptically in one large (3 L capacity) collapsible plastic bag, the contents of which are infused over a 12–24 hour period. Transfer of amino acid, glucose and electrolyte infusions, and the addition of vitamins and trace elements must be carried out with great care under aseptic conditions to avoid microbial contamination. These solutions often provide good growth conditions for bacteria and moulds. Fats are administered as oil-in-water emulsions comprising small droplets of a suitable vegetable oil (e.g. soyabean) emulsified with egg lecithin and sterilized by autoclaving. In many cases, the fat emulsion is added to the 3 L bag. Thus TPN fluids are complex mixtures and a multitude of potential interactions, both chemical and physicochemical, may occur between their individual components resulting in decomposition, creaming, precipitation or even the formation of toxic by-products. Trace elements, calcium, vitamins and lipids are particularly prone to affecting the stability.
Although many vitamins may be administered as a single dose at various time intervals, many of the patient’s requirements will be found in what is basically an emulsion formulation, prepared aseptically and thus with no terminal sterilization. The product usually contains both essential and non-essential amino acids rather than fully formed protein, and energy is provided at a ratio of 0.6–1.1 MJ per gram of protein nitrogen. A mixture of carbohydrate (glucose) and fat (as an emulsion) provides the energy and electrolytes, trace elements and vitamins are included as required. Thus the TPN fluid is prepared to suit the individual patient’s needs. The fact that the product contains so many ingredients makes TPN fluids extremely difficult to prepare, and once vitamins are added, their chemical instability reduces the shelf life. During preparation, TPN fluids are compounded from individual solutions or emulsions. Generally, the bulk of the final volume is derived from glucose solutions, amino acid solutions and fat emulsions; small-volume solutions are added to these before filling. During compounding, electrolytes are added to the amino acid solutions and phosphate salts to the dextrose (glucose) solutions, which are then mixed and the lipid emulsions added. This order of mixing is adopted because the pH of glucose solutions decreases due to degradation during their sterilization and addition of emulsions to this low pH solution might cause emulsion instability. The mixing of the amino acids with the glucose solutions provides a vehicle with some degree of buffering capacity. Calcium might precipitate as the phosphate if its salts were to be added directly to the phospholipid emulsion. Vitamins are added to the lipid emulsion or to the bag immediately before use.
A number of other difficulties may be encountered. Polyunsaturated acids are subject to hydrolysis. Any residual air might cause oxidation of labile vitamins, e.g. vitamin C. Lipids (the fat emulsions and fat-soluble vitamins formulated as an emulsion) may extract plasticizer from a plastic container, especially if the bag is based on PVC. Any electrolytes may compromise emulsion stability by altering the electrochemistry around the dispersed oil droplets, thus allowing the droplets to move closer to each other (due to a disruption of the Stern layer) and coalesce; a less noticeable problem would be changes in the globule size. Additionally, the plastic bag might absorb the oil-soluble vitamins and care has to be taken in the selection of the container to avoid moisture loss. As a final example of the complex nature of TPN fluids, amino acids may undergo the Maillard reaction with glucose, resulting in discoloration. An account of the clinical aspects of TPN can be found in Harper & Lamerton (2009).
2.1.3 Small-volume injections
This category includes single-dose injections, usually of 1–2 ml but as high as 50 ml, dispensed in borosilicate glass ampoules, plastic (polyethylene or polypropylene) ampoules or, rarely, multiple-dose glass vials of 5–25 ml capacity stoppered with a rubber closure through which a hypodermic needle can be inserted, e.g. insulins, vaccines. The closure is designed to reseal after withdrawal of the needle. It is unwise to include too many doses in a multiple-dose container because of the risk of microbial contamination during repeated use. Preservatives must be added to injections in multiple-dose containers to prevent contamination during withdrawal of successive doses. However, preservatives may not be used in injections in which the total volume to be injected at one time exceeds 15 ml. This may occur if the solubility of a drug is such that a therapeutic dose can only be achieved in this volume of solvent. There is also an absolute prohibition on the inclusion of preservatives in intra-arterial, intracardiac, intrathecal or subarachnoid, intracisternal and peridural injections, and various ophthalmic injections.
2.1.3.1 Small-volume oily injections
Certain small-volume injections are available where the drug is dissolved in a viscous oil because it is insoluble in water and therefore a non-aqueous solvent is used. In addition, drugs in non-aqueous solvents provide a depot effect, e.g. for hormones. The intramuscular route of injection must be used. The vehicle may be a metabolizable fixed oil such as arachis oil or sesame oil (but not a mineral oil) or an ester, such as ethyl oleate, which is also capable of being metabolized. The latter is less viscous and therefore easier to administer, but the depot effect is of shorter duration. The drug is normally dissolved in the oil, filtered under pressure and distributed into ampoules. After sealing, the ampoules are sterilized by dry heat. A preservative is probably ineffective in such a medium and therefore offers very little protection against contamination in a multiple-dose oily injection.
2.1.4 Freeze-dried products
In brief, freeze-drying (lyophilization) consists of preparing the drug solution (with buffers and cryoprotectants), filtering through a bacteria-proof filter, dispensing into containers, removing water in a freeze-drier, then capping and closing the containers. Many biotechnology products are freeze-dried.
Freeze-drying is an aseptic process whereby water is removed from a frozen product mainly by sublimation, i.e. by the conversion of ice directly into the vapour state without the intermediary of liquid water. It is a batch process, of relatively long duration, and is used frequently for drugs of poor stability. Drugs are reconstituted into solution immediately prior to injection. The process consists of three stages:
- freezing, which slows down degradation and solidifies the product
- primary drying, whereby energy is provided to the system and a vacuum applied to expedite the removal of moisture at subambient temperatures
- secondary drying, whereby the product is heated to remove the last traces (2%) of water.
A number of characteristics of the formulation control the behaviour of the product during the lyophilization cycle. These include the glass transition temperature and the collapse temperature. The maintenance of sterility and retention of the appropriate sterility assurance level (SAL) is implicit in the freeze-drier design (Pikal, 2007). Although membrane-filtered sterile solutions may be used to fill containers to be placed into the freeze-drier, other measures to maintain sterility are also employed. These include using steam sterilization of the drier; gaseous sterilization has not been widely adopted. The temperature of shelves is regulated using a circulating fluid such as dimethylsiloxane oil. Electronics and computerization have led to the accumulation of better data for validation. Stoppering systems allow the successful sealing of the containers and gas entering the drier may be filtered to effect sterilization.
2.1.5 Packaging, closures and blow-fill technology
The packaging and closures must prevent loss of vehicle, excipient or drug during sterilization and storage. Additionally, ingress of microorganisms must be prevented. The packaging must not contribute any significant amounts of extractable chemicals to the contents, e.g. vulcanizing agents from rubbers or plasticizers from PVC infusion containers.
2.1.5.1 Glass containers
Single-dose injections are usually packed in glass ampoules containing 1, 2 or 5 ml of product. To ensure removal of the correct dose volume by syringe and needle, it is necessary to add an appropriate overage to the ampoule. Thus a 1 ml ampoule will actually contain 1.1 ml of product and a 2 ml ampoule should contain 2.15 ml of product.
Many injectables are sealed with a rubber closure held on by an aluminium screw-cap or crimp-on ring. The rubber should be non-fragmenting, not release soluble extractives, and be sufficiently soft and pliable to seal around the needle inserted immediately prior to use. Although filled bottles are sterilized by autoclaving, it is still possible for the infusions in glass bottles to become contaminated with microorganisms through the seal before use. For instance, during the final part of the autoclave cycle, bottles may be spray-cooled with water to hasten the cooling process. However, if there is a poor fit between bottle lip and rubber plug (a skirted inset type is used) it is possible for the spray-cooling water to spread by capillary movement between bottle thread and screw-cap and even to enter the bottle contents. Failure may also result from any imperfection of the bottle or plug. Microorganisms may gain access to the product within the containers during storage if hairline cracks (due to bad handling or rough treatment) are present which permit fluid seepage. Finally, contamination may occur during use (1) if poor aseptic techniques are applied when setting up the infusion, (2) via an ineffective air inlet (which allows replacement of the infused fluid with air in glass bottles), or (3) when changing the giving set or bottle.
Three types of glass are suitable for use in the manufacture of containers for injectable preparations. These are a neutral borosilicate glass, a sulphated soda glass and a soft, moderately hydrolytic resistant glass. The glasses are classified by their hydrolytic resistance. The choice for a container depends on the properties of the solution they are used to package. The advantages of glasses as container materials include their chemical resistance, the fact that they do not absorb or leach organic materials, their impermeability to water vapour and other gases, their transparency, their ability to form rigid strong stable containers which resist puncture, their ability to hold a vacuum and their overall stability to moist heat or dry heat sterilization. However, glass containers may break and crack during the sterilization process, they are attacked by alkaline solutions (and so may be a problem with, for example, sodium citrate bladder irrigation), they are heavy and require venting during administration of their contents.
2.1.5.2 Closures
Closures are made of a polymer and their formulation include curing agents, activators, antioxidants, plasticizers, fillers and pigments. They have to be selected with the drug product in mind to avoid chemical incompatibility and possible reaction with the ingredients in the product formulation. Sorption of the preservative from multiple-dose formulations has frequently been a problem and closures may therefore require saturation with the ingredients in the product prior to packaging.
Closures should be flexible, to conform to the shape of the vial; resilient, so as to reseal after each needle puncture; tough, so that low fragmentation levels occur when punctured; non-thermoplastic, so that the heat sterilization process is tolerated; and chemically compatible with the drug formulation. Early closures were sulphur-based, and easily cured with accelerators to speed up the curing rate. Unfortunately, a high degree of water-extractable by-products could be taken up by the product that they were intended to protect. Consequently they have been replaced by modern polymer formulations with low extract curatives. Bromobutyl and chlorobutyl rubbers show superior performance although special polymers, e.g. nitrile rubbers, are used for mineral oil products. Problems of incompatibility may be overcome by film bonding a fluorocarbon barrier film to the surfaces of the closures.
2.1.5.3 Plastic containers
Most infusions are now packed in plastic containers. The plastic material should be pliable, thermoresistant, transparent and non-toxic. The plastics may contain antioxidants, stabilizers, lubricants, plasticizers, fillers and colorants. Suitable materials are PVC (which may present a problem with moisture loss) and polyethylene. The former is transparent and very pliable, allowing the pack to collapse as the contents are withdrawn (consequently no air inlet is required). These packs are also amenable to the inclusion of ports into the bag, allowing greater safety during use. Such ports may be protected by sterile overseals.
Two problems arise: (1) the possibility of toxic extractives, e.g. diethyl phthalate, from the plastic entering the fluid if poor quality PVC is used, and (2) moisture permeability leading to loss of water if the packs are not protected by a water-impermeable outer wrap. Bags of high-quality polyethylene are readily moulded (although separate ports cannot be included), translucent and free from potential toxic extractives. Again, these packs normally collapse readily during infusion. An important advantage of all plastic packs is that the containers are hermetically sealed prior to autoclaving and therefore spray-cooling water cannot enter the pack unless there is seal failure, an easily detected occurrence. However, autoclaving of plastic bags is more complex than that of bottled fluids because a steam/air mixture is necessary to prevent bursting of the bags when heated (air ballasting); adequate mixing of the steam and air is therefore required to prevent layering of gases inside the chamber.
2.1.5.4 Blow-fill technology
Blow-fill technology is an aseptic process whereby the container is formed from thermoplastic granules, filled with sterile solution and sealed, all within one automatic operation. The bulk solution should have a low bioburden and is delivered to the machine through a filling system that has been previously sanitized and steam sterilized in situ. Concern has been expressed that the machine itself may generate particles. The plastic granules are composed usually of polyethylene, polypropylene or one of their copolymers and are heat extruded at about 200°C into a tube. The two halves of a mould close around this tube and seal the base. The required quantity of sterile fluid is filled into the container, which is then sealed. Products packed in this way include intravenous solutions, and small-volume parenteral, ophthalmic and nebulizer solutions. The technique offers lower costs than conventional packaging.
2.1.5.5 Cartridges and ready-to-use syringes
Small-volume injections may also be packaged in cartridges or directly into disposable syringes. The latter are immediately available for use but have a high cost of production and their fixed content may lead to waste of material that remains uninjected after single use. Cartridges are lower cost and may be fitted into injection pens; many insulin products are produced in this manner because of their low waste, ease of use and not requiring the patient to draw a dose volume into a separate syringe. Cartridges have a plunger stopper at one end of a cylindrical glass body containing the product for injection, and the other end is sealed with a rubber-lined crimp cap. Processing steps include preparing the bulk sterile solution for injection, washing and siliconizing the plunger stoppers, caps and glass cartridges, inserting the plunger stopper, filling and closing. The product is then sterilized, but care has to be taken that the internal pressures that develop during the autoclave cycle do not force the cartridge plunger out of the cartridge. The industry is developing a range of devices designed to breach the skin’s defences to allow transdermal delivery (Arora et al., 2008). These include microneedle arrays and needle-free injections.
2.1.6 Quality control of ampoules and infusion containers
2.1.6.1 Particulate c ontamination
Because of the possible clinical consequences (such as granuloma of the lung) of injecting solid particles into the bloodstream, the number of particles present in injections and other solutions used in body cavities must be restricted. The British Pharmacopoeia (2010) states that injectable preparations which are solutions ‘when examined under suitable conditions of visibility are clear and practically free from particles’. It also sets limits for sub-visible particles in injections based on the principle of light blockage. Not more than 100 particles/ml greater than 5 μm and not more than 50 particles/ml greater than 10 μm should be generally obtained. The British Pharmacopoeia (2010) describes a microscopic method for determination of the particulate contamination of injections and intravenous infusions. The counting methods should estimate extraneous particles, but not bubbles, that are unintentionally present in the solutions. If the method provides a means for identifying and detecting the particles, insight may be gained into their possible origin. Filtration and observation using light microscopy have clear advantages, including simplicity and allowing the operator to visualize the particles.
All parenterally injected solutions should be checked for particulate contamination, but the above procedure is clearly impractical as a bulk screening exercise. Those products contaminated with particulate matter should be rejected. In practice, all products may be tested individually by a human observer against split white/black screens and/or under polarized light for obvious particulate contamination, and again there is a method described in pharmacopoeias based on the split-screen technique. Nowadays optical control equipment can take over this arduous and boring task.
2.1.6.2 Integrity of seals
The integrity of sealing of ampoules should be assessed on an individual basis. Two techniques are available that depend on dye ingress under vacuum or electronic means. With dye intrusion, the ampoules are submerged in a dye solution and under an applied vacuum. Any container that has cracks in its structure or is not sealed will admit the dye when the vacuum is reduced. On washing, badly sealed ampoules will be coloured. This technique underestimates the problem of bad sealing. In the alternative technique, high-frequency spark testing, the presence of a leak causes a change in a high-frequency electrical signal placed across the ampoule. The method is limited to aqueous products with a high conductivity. It is a very sensitive technique and detects weak seals not detected by the dye test. In reality, both tests should be used in parallel.
2.2 Non-injectable sterile fluids
There are many other types of solution in a sterile form, for use particularly in hospitals.
2.2.1 Non-injectable water
This is sterile water, not necessarily of injectable water standards, which is used widely during surgical procedures for wound irrigation, moistening of tissues, washing of surgeons’gloves and instruments during use and, when warmed, as a haemostat. Isotonic saline may also be used. Topical water (as it is often called) is prepared in 500 ml and 1 l polyethylene or polypropylene containers with a wide neck and tear-off cap to allow for ease of pouring.
2.2.2 Urological (bladder) irrigation solutions
These are used for rinsing of the urinary tract to aid tissue integrity and cleanliness during or after surgery. Either water or glycine solution is used, the latter eliminating the risk of intravascular haemolysis when electrosurgical instruments are used. These are sterile solutions produced in collapsible or semirigid plastic containers of up to 3 L capacity.
2.2.3 Peritoneal dialysis and haemodialysis solutions
Peritoneal dialysis solutions are admitted into the peritoneal cavity as a means of removing accumulated waste or toxic products following renal failure or poisoning. They contain electrolytes and glucose (1.4–7% w/v) to provide a solution equivalent to potassium-free extracellular fluid; lactate or acetate is added as a source of bicarbonate ions. Slightly hypertonic solutions are usually employed to avoid increasing the water content of the intravascular compartment. A more hypertonic solution containing a higher glucose concentration is used to achieve a more rapid removal of water. In fact, the peritoneal cavity behaves as if it were separated from the body organs by a semipermeable membrane. Warm peritoneal solution (up to 5 L) is perfused into the cavity for 30–90 minutes and then drained out completely. This procedure can then be repeated as often as required. As the procedure requires larger volumes, these fluids are commonly packed in 2.5 L containers. It is not uncommon to add drugs (for instance potassium chloride or heparin) to the fluid prior to use.
Haemodialysis is the process of circulating a patient’s blood through a machine via tubing composed of a semipermeable material such that waste products permeate into the dialysing fluid and the blood then returns to the patient. Haemodialysis solutions need not be sterile but must be free from heavy bacterial contamination.
2.2.4 Inhaler solutions
In cases of severe asthmatic attacks, bronchodilators and steroids for direct delivery to the lungs may be needed in large doses. This is achieved by direct inhalation via a nebulizer device; this converts a liquid into a mist or fine spray. The drug is diluted in small volumes of Water for Injections before loading into the reservoir of the machine. This vehicle must be sterile and preservative-free and is therefore prepared as a terminally sterilized unit dose in polyethylene nebules.
2.3 Ophthalmic preparations
2.3.1 Design philosophy
Medication intended for instillation on to the surface of the eye is formulated in aqueous solution as eye drops or lotion or in an oily base as an ointment. Because of the possibility of eye infection occurring, particularly after abrasion or damage to the corneal surface, all ophthalmic preparations must be sterile. As there is a very poor blood supply to the anterior chamber, defence against microbial invasion is minimal; furthermore, it appears to provide a particularly good environment for growth of bacteria. As well as being sterile, eye products should also be relatively free from particles that might cause damage to the cornea. However, unlike aqueous injections the recommended vehicle is purified water because the presence of pyrogens is not clinically significant.
Another type of sterile ophthalmic product is the contact lens solution. However, unlike the other types this is not used for medication purposes but merely as wetting, cleaning and soaking conditions for contact lenses.
2.3.2 Eye drops
Some typical excipients for eye drops are given in Table 22.1. Eye drops are presented for use in (1) sterile single-dose plastic sachets (often termed Minims) containing 0.3–0.5 ml of liquid, (2) multiple-dose amber fluted eye dropper bottles including the rubber teat as part of the closed container or supplied separately, or (3) plastic bottles with integral dropper. A breakable seal indicates that the dropper or cap has not been removed prior to initial use. Although a standard design of bottle is used in hospitals, many proprietary products are manufactured in plastic bottles designed to improve safety and care of use. The maximum volume in each container is limited to 10 ml. Because of the likelihood of microbial contamination of eye dropper bottles during use (arising from repeated opening or contact of the dropper with infected eye tissue or the hands of the patient), it is essential to protect the product with a preservative (Matthews & Skinner, 2006). Eye drops for surgical theatre use should be supplied in single-dose containers.
Examples of preservatives are phenylmercuric nitrate or acetate (0.002% w/v), chlorhexidine acetate (0.01% w/v), thiomersal (0.01% w/v) and benzalkonium chloride (0.01% w/v). Chlorocresol is too toxic to the corneal epithelium, but 8-hydroxyquinoline and thiomersal may be used in specific instances. The principal consideration in relation to antimicrobial properties is the activity of the bactericide against Pseudomonas aeruginosa, a major source of serious nosocomial eye infections. There is some concern over the toxicity of mercurials, and their use is becoming less common. Although benzalkonium chloride is probably the most active of the recommended preservatives, it cannot always be used because of its incompatibility with many compounds commonly used to treat eye diseases, nor should it be used to preserve eye drops containing anaesthetics. As benzalkonium chloride reacts with natural rubbers, silicone or butyl rubber teats should be substituted and products should not be stored for more than 3 months after manufacture because silicone rubber is permeable to water vapour. As with all rubber components, the rubber teat should be pre-equilibrated with the preservative before use. Thermostable eye drops and lotions are sterilized at 121°C for 15 minutes. For thermolabile drugs, filtration sterilization followed by aseptic filling into sterile containers is necessary. Eye drops in plastic bottles are prepared aseptically.
In order to lessen the risk of eye drops becoming heavily contaminated, either by repeated inoculation or by the growth of resistant organisms in the solution, use is restricted to 1 month after the container is first opened. This is usually reduced to 7 days for hospital ward use on one eye of a single patient. The period is shorter in the hospital environment because of the greater danger of contamination by potential pathogens, particularly pseudomonads.
2.3.3 Eye lotions
Eye lotions are isotonic solutions used for washing or bathing the eyes. They are sterilized by autoclaving in relatively large-volume containers (100 ml or greater) of coloured fluted glass with a rubber closure and screw-cap, or packed in plastic containers with a screw-cap or tear-off seal. They may contain a preservative if intended for intermittent domiciliary use for up to 7 days. If intended for first aid or similar purposes, however, no bactericide is included and any remaining solution is discarded after 24 hours.
2.3.4 Eye ointments
Eye ointments are prepared in a semisolid base—e.g. Simple Eye Ointment BP, which consists of yellow soft paraffin (8 parts), liquid paraffin (1 part) and wool fat (1 part). The base is filtered when molten to remove particles and sterilized at 160°C for 2 hours. The drug is incorporated prior to sterilization if heat-stable, or added aseptically to the sterile base. Finally the product is aseptically packed in clear sterile aluminium or plastic tubes. As the product contains virtually no water, the danger of bacteria proliferating in the ointment is negligible.
2.3.5 Contact lens solutions
Most contact lenses are worn for optical reasons as an alternative to spectacles. Contact lenses are of two types: hard lenses, which are hydrophobic, and soft lenses, which may be either hydrophilic or hydrophobic. The surfaces of lenses must be wetted before use and wetting solutions are used for this purpose. Hard, and more especially, soft lenses become heavily contaminated with protein material during use and therefore must be cleaned before disinfection. Contact lenses are potential sources of eye infection and, consequently, microorganisms should be removed before the lens is again inserted into the eye. Lenses must also be clean and easily wettable by lachrymal secretions. Contact lens solutions are thus sterile solutions of the various types described below. Apart from achieving their stated functions, either singly or in combination, all solutions must be non-irritating or must protect against microbial contamination during use and storage.
2.3.5.1 Wetting solutions
These are used to hydrate the surfaces of hard lenses after disinfection. As they must also cope with chance contamination, they must contain a preservative as well as a wetting agent. They may be isotonic with lachrymal secretions and be formulated to a pH of about 7.2 for compatibility with normal tears.
2.3.5.2 Cleaning solutions
These are responsible for the removal of ocular debris and protein deposits, and contain a cleaning agent that consists of a surfactant and/or an enzyme product. As they must also cope with chance contamination, they contain a preservative, are isotonic and have a pH of about 7.2.
2.3.5.3 Soaking solutions
These are solutions for disinfection of lenses but also maintain the lenses in a hydrated state. The antimicrobial agents used for disinfecting hard lenses are those used in eye drops (benzalkonium, chlorhexidine, phenylmercuric acetate or nitrate, thiomersal and chlorbutol). Ethylenediamine tetraacetic acid (EDTA) is usually present as a synergist. Benzalkonium chloride and chlorbutol are strongly bound to hydrophilic soft contact lenses and therefore cannot be used in storage solutions for these; chlorhexidine and thiomersal are usually employed. It must be added that the concentrations of all preservatives used in contact lens solutions are lower than those employed in eye drops, to minimize irritancy. Hydrogen peroxide is becoming commonly used but must be inactivated before the lenses are inserted onto the eyes. Finally, heat may be utilized as an alternative method to disinfect soft contact lenses, especially the hydrophilic types. Lenses are boiled in isotonic saline.
2.4 Dressings
Dressings and surgical materials are used widely in medicine, both as a means of protecting and providing comfort for wounds and for many associated activities such as cleaning and swabbing. They may or may not be used on areas of broken skin. If there is a potential danger of infection arising from the use of a dressing then it must be sterile. For instance, sterile dressings must be used on all open wounds, both surgical and traumatic, on burns, and during and after catheterization at a site of injection. It is also important to appreciate that sterile dressings must be packaged in such a way that they can be applied to the wound aseptically.
Dressings are described in the British Pharmacopoeia (2010). Methods for their sterilization include autoclaving, dry heat, ethylene oxide and ionizing radiation. Any other effective method may be used. The choice is governed principally by the stability of the dressing constituents to the stress applied and the nature of their components. Most celluloses and synthetic fibres withstand autoclaving, but there are exceptions. For instance, boric acid tenderizes cellulose fibres during autoclaving, and dressings containing waxes cannot be sterilized by moist heat. Certain constituents are also adversely affected on exposure to large doses of gamma radiation. Examples of dressings that are required to be sterile are listed in Table 22.2, together with other dressings and materials that may be sterilized when required.
Table 22.2 Uses of surgical dressings and methods of sterilization
Dressing | Uses | Method of sterilization |
Required to be sterile | ||
Chlorhexidine gauze dressing | Medicated open wound dressing, burns, grafts | Any combination of dry heat, gamma radiation and ethylene oxide |
Framycetin gauze dressing | Medicated open wound dressing, burns, grafts | |
Knitted viscose primary dressing | Ulcerative and granulating wounds | |
Paraffin gauze dressing | Burns, scalds and grafts | |
Perforated film absorbent dressing | Postoperative wounds | |
Polyurethane foam dressing | Burns, ulcers, grafts, granulating wounds | |
Semipermeable adhesive dressing | Adhesive dressing for open wounds, IV sites, stoma care, etc. | |
Sodium fusidate gauze dressing | Medicated open wound dressing, burns, grafts | |
May be sterile for use in certain circumstances | ||
Absorbent cotton wool | Swabbing, cleaning, medication application | Any method |
Elastic adhesive dressing | Protective wound dressings | Ethylene oxide or gamma radiation |
Plastic wound dressings | Protective dressing (permeable or occlusive) | Ethylene oxide or gamma radiation |
Absorbent cotton gauze | Absorbent wound dressing | Any method |
Gauze pads | Swabbing, dressing, wound packing | Any method |
Absorbent viscose wadding | Wound cleaning, swabbing, skin antiseptic | Any method |
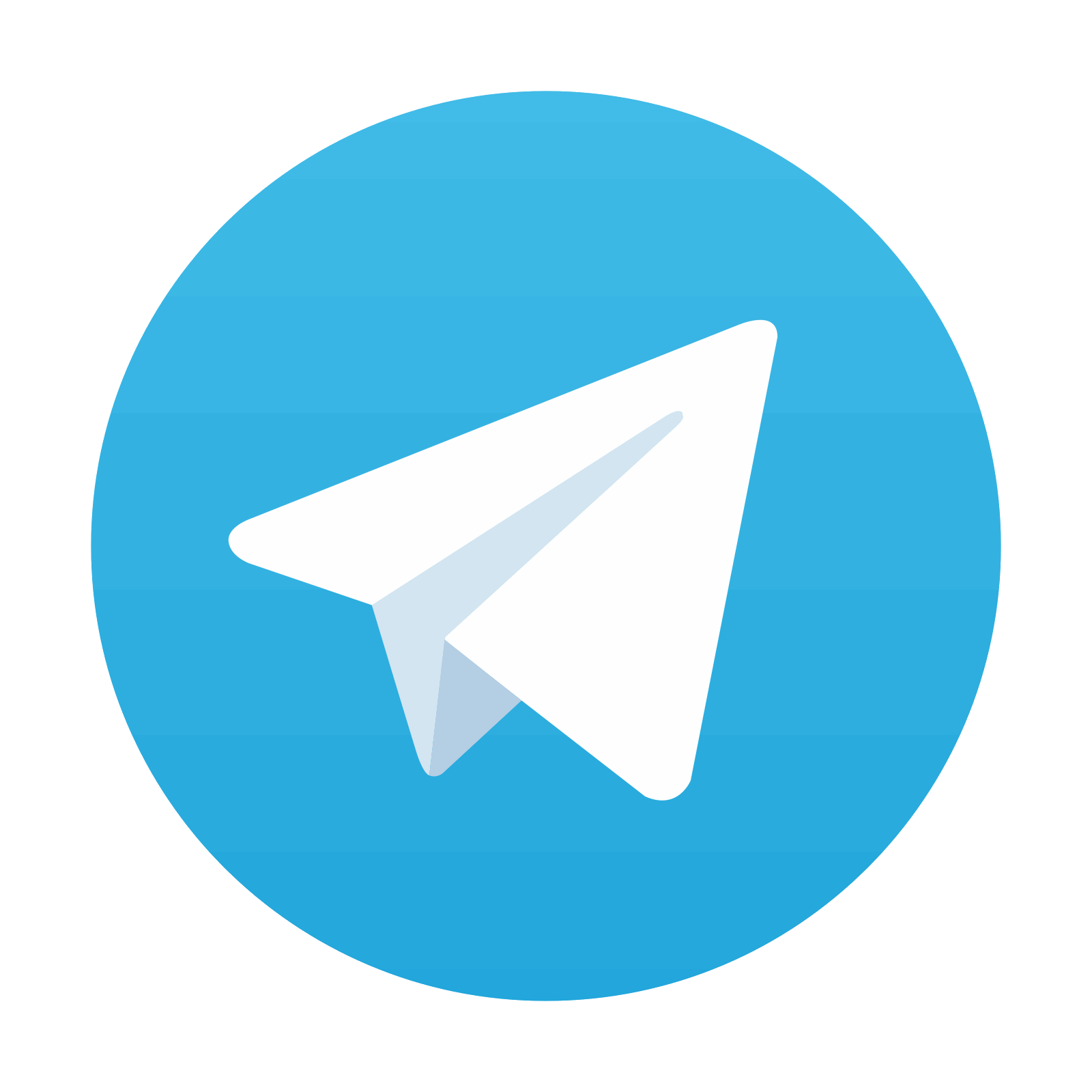