Chapter 49
Shock, Multiple Organ Dysfunction Syndrome, and Burns in Children
Mary Fran Hazinski, Mary A. Mondozzi and Rose A. Urdiales Baker
Shock and Multiple Organ Dysfunction Syndrome
Shock in children is present when there are signs of poor systemic perfusion, regardless of the blood pressure—shock may be present with normal, high, or low blood pressure. When the systolic blood pressure is adequate for age, but there are signs of inadequate tissue perfusion, the child is in compensated shock. In this child, although the systolic blood pressure may be normal, the diastolic or mean blood pressure is typically low. If systolic hypotension is associated with inadequate tissue perfusion, the child is in hypotensive (formerly called decompensated) shock.1–3
Multiple organ dysfunction syndrome (MODS) is the simultaneous failure of at least two organs resulting from a single cause. MODS may be either primary or secondary. Primary MODS is directly attributable to the insult and typically occurs soon (3 to 7 days) after an insult. Secondary MODS typically occurs later and may be associated with more sequential development of organ dysfunction. Risk factors for MODS include severe or prolonged shock, sepsis, trauma, cardiopulmonary arrest, congenital heart disease, and liver and bone marrow transplantation.3–5 Children with chronic diseases have an increased risk for MODS and increased mortality.5
Types of Shock
Shock is categorized by type as follows:2,3
1. Hypovolemic shock: caused by inadequate intravascular volume relative to the vascular space
2. Cardiogenic shock: results from impairment of myocardial function
3. Distributive shock (including septic, anaphylactic, and neurogenic): results from inappropriate distribution of blood flow, increased capillary permeability, and myocardial dysfunction (e.g., septic or anaphylactic shock) or central nervous system injury (e.g., neurogenic or spinal shock)
4. Obstructive shock: caused by a mechanical obstruction to blood flow into and through the heart and great vessels (e.g., cardiac tamponade, pulmonary embolus, obstructive congenital heart lesions such as critical aortic stenosis) resulting in low cardiac output
In obstructive shock, low cardiac output is caused by mechanical obstruction to blood flow, such as cardiac tamponade, tension pneumothorax, critical left heart or aortic obstruction, or pulmonary embolus.2 Obstructive shock is difficult to distinguish from cardiogenic shock because both may present with signs of low cardiac output and evidence of systemic or pulmonary venous congestion. Prompt detection and treatment are critical to survival.2,3
Clinical Manifestations of Shock
The child with inadequate cardiac output demonstrates signs of inadequate blood flow to some tissue beds and evidence of organ system dysfunction (Box 49-1). Critical parameters to evaluate when approaching the child include assessment of consciousness, breathing, and color. This initial assessment is described in the Pediatric Advanced Life Support course.2 Through this assessment the provider determines if the child “looks good” (appears stable, in no acute distress) or “looks bad” (in need of immediate intervention).6 More detailed assessment of organ function and acid-base status will detect evidence of inadequate organ perfusion.
The child’s level of consciousness and responsiveness often provides valuable information about the severity of illness. The healthy infant should orient to faces, make eye contact, and track bright objects across a visual field. The healthy toddler is reluctant to be separated from parents or examined by strangers, and the healthy child is alert and responds to questions. By comparison, the critically ill infant or child is often extremely irritable; lethargy indicates severe deterioration in the child’s level of consciousness. A decreased response to painful stimulation is abnormal and usually indicates severe cardiorespiratory or neurologic compromise.2,6
If perfusion is adequate and the ambient temperature is warm, the child’s color will be consistent over the surface of the skin, with pink lips and mucous membranes. Children who are in a cold environment, those who have undergone hypothermic surgery or a procedure in a cold room, and children in shock often demonstrate mottling (a marbled or “blotchy” appearance to the skin).2,3,6 Pallor also may be observed when perfusion is poor (Figure 49-1). Children with sepsis occasionally demonstrate flushed, bright red skin.2,3,6
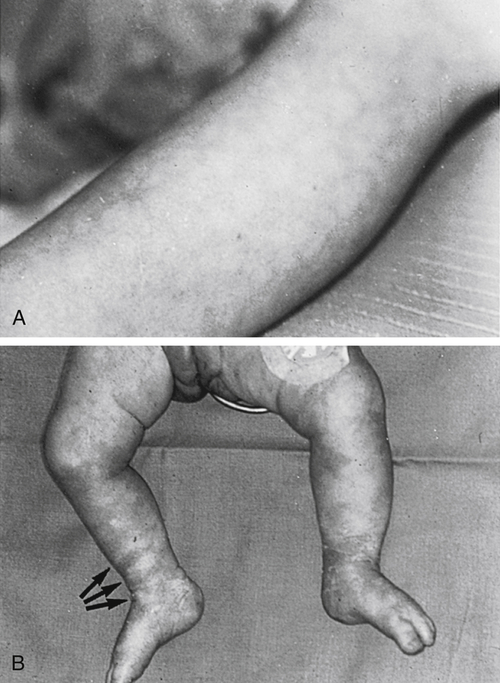
A, Mottling of skin color often indicates inadequate tissue oxygenation; this may result from hypoxemia or poor systemic perfusion. This child developed myocardial dysfunction and signs of cardiogenic shock. B, Mottled skin color is often associated with other signs of compromise of skin perfusion, including delayed capillary refill. The skin over this infant’s right ankle was blanched using three fingers (arrows), and the skin failed to perfuse for more than 5 seconds. This infant suffered from septic shock. (From Hazinski MF: Cardiovascular disorders. In Hazinski MF, editor: Nursing care of the critically ill child, ed 2, St Louis, 1992, Mosby.)
If perfusion is adequate and the ambient temperature is warm, the child’s capillary refill is normally brisk (<2 seconds). A prolonged capillary refill time (>2 seconds ) may indicate a compromise in systemic perfusion (see Figure 49-1, B) and the development of shock.2,3,6 However, evaluation of capillary refill time is subjective and gradual changes may be difficult to detect.7
The child’s vital signs must be evaluated in light of the child’s clinical condition. Normal vital signs are not always appropriate in the seriously ill or injured child6 (Box 49-2 and Table 49-1).
TABLE 49-1
HEART RATES | ||||
AGE | AWAKE HEART RATE (PER MIN) | SLEEPING HEART RATE (PER MIN) | RESPIRATORY RATE∗ (BREATHS PER MIN) | |
Newborn | 100-205 | 90-160 | ||
Infant (6 mo) | 100-180 | 90-160 | 30-53 | |
Toddler | 98-140 | 80-120 | 27-37 | |
Preschooler | 80-120 | 65-100 | 20-28 | |
School-age child | 75-118 | 58-90 | 18-25 | |
Adolescent | 60-100 | 50-90 | 12-20 | |
BLOOD PRESSURES | ||||
AGE | SYSTOLIC BLOOD PRESSURE (mmHg)† | DIASTOLIC BLOOD PRESSURE (mmHg)† | MEAN ARTERIAL PRESSURE (mmHg)‡ | SYSTOLIC HYPOTENSION (mmHg)§ |
Birth (12 hr, <1000 g) | 39-59 | 16-36 | 28-42¶ | <40-50 |
Birth (12 hr, 3-kg weight) | 60-76 | 31-45 | 48-57 | <50 |
Newborn (96 hr) | 67-84 | 35-53 | 45-60 | <60 |
Infant (1-12 mo) | 72-104 | 37-56 | 50-62 | <70 |
Toddler (1-2 yr) | 86-106 | 42-63 | 49-62 | <70 + (2 × age in years) |
Preschool (3-5 yr) | 89-112 | 46-72 | 58-69 | <70 + (2 × age in years) |
School-age child (6-7 yr) | 97-115 | 57-76 | 66-72 | <70 + (2 × age in years) |
Preadolescent (10-12 yr) | 102-120 | 61-80 | 71-79 | <90 |
Adolescent (12-15 yr) | 110-131 | 64-83 | 73-84 | <90 |
Blood pressure ranges from the following sources: Gemelli M et al: Eur J Pediatr 149:318–320, 1990; Versmold H et al: Pediatrics 67:107, 1981; Haque IU, Zaritsky AL: Pediatr Crit Care 8:138–144, 2007; National Heart, Lung and Blood Institute: Fourth report on the diagnosis, evaluation, and treatment of high blood pressure in children and adolescents, Bethesda, MD, 2004, National Heart, Lung and Blood Institute. Available at www.nhlbi.nih.gov/guidelines/hypertension/child.tbl.htm. Accessed July 10, 2013.
∗Respiratory rates from Fleming S et al: Normal ranges of heart rate and respiratory rate in children from birth to 18 years of age: a systematic review of observational studies, Lancet 377:1011–1018, 2011.
†Systolic and diastolic blood pressure ranges assume 50th percentile for height for children 1 year and older, and are consistent with the Pediatric Advanced Life Support course (Chameides L et al: Pediatric advanced life support provider manual, Dallas, 2011, American Heart Association).
‡Mean arterial pressures (Diastolic pressure + [Difference between systolic and diastolic pressures ÷ 3]) for 1 year and older, assuming 50th percentile for height.
§Threshold for hypotension in children 1 to 10 years old from Pediatric Advanced Life Support course (Chameides L et al: Pediatric advanced life support provider manual, Dallas, 2011, American Heart Association).
¶Approximately equal to postconception age in weeks (may add 5 mmHg).
From Hazinski MF: Children are different. In Hazinski MF, editor: Nursing care of the critically ill child, ed 3, St Louis, 2013, Mosby.
The child’s heart rate should be appropriate for age and clinical condition. The child in shock is often tachycardic. The tachycardia may be primary (i.e., associated with a tachydysrhythmia) or secondary to stress (i.e., sinus tachycardia). If the heart rate is extremely rapid or if it is present in the child with decreased myocardial function, tachycardia may be the cause rather than the symptom of shock. In general, if the ventricular rate exceeds 200 to 220/min in the infant or 160 to 180/min in the child, ventricular diastolic filling time and coronary artery perfusion time are significantly reduced and stroke volume falls. As a result, cardiac output falls and signs of heart failure or shock develop. Once supraventricular or ventricular tachycardia produces signs of shock, urgent treatment is required.8
Bradycardia, an abnormally low heart rate, can cause a fall in cardiac output or it can be a symptom of deterioration. In young animal models, a fall in heart rate produces a commensurate fall in cardiac output.9 The most common cause of bradycardia in young children is hypoxia.2,10 Therefore, if the infant or child develops bradycardia with poor perfusion, the provider must immediately assess and support the child’s airway, oxygenation, and ventilation, and be prepared to initiate chest compressions if the heart rate and systemic perfusion do not improve.2,6,10
Bradycardia often indicates impending cardiovascular collapse and is the most common terminal cardiac rhythm observed in children.2,3,6,10 The child’s stroke volume may be altered by conditions affecting ventricular preload, compliance, contractility, and afterload (Table 49-2). Evaluate and optimize each of these variables in the treatment of shock (see Chapter 48).
TABLE 49-2
FACTORS AFFECTING CARDIOVASCULAR PERFORMANCE IN CHILDREN
FACTOR | COMMENTS |
Heart rate | Major factor affecting cardiac output in children. Normally more rapid in children than in adults. Because the stroke volume is smaller than in adults, the cardiac output of the child is more closely related to heart rate than stroke volume. Tachycardia is expected in the seriously ill or injured child. The most common cause of bradycardia in young children is hypoxia; bradycardia is an ominous sign if present in association with poor perfusion. Urgent treatment is required once bradycardia or supraventricular or ventricular tachycardia produces signs of shock. |
Stroke volume | Averages 1.5 ml/kg; affected by conditions altering ventricular preload, compliance, contractility, and afterload |
Ventricular end-diastolic pressure (VEDP) | Optimal pressure for children in shock is unknown. Aggressive fluid administration is linked to improved survival in children with septic shock. |
Ventricular compliance or distensibility | Can be affected by congenital heart defects such as atrial septal defects (ASDs) and complex congenital heart defects. If compliance is low, such as in newborns and infants, volume administration may increase VEDP. Hypoplastic ventricles are often noncompliant. Hypertrophied ventricles, present in children with severe pulmonary stenosis or aortic stenosis, may become fibrotic and noncompliant. Increased compliance may be present in early septic shock. |
Contractility | Contractility probably similar in normal newborns, infants, children, and adults. Newborn myocardium does have fewer contractile proteins and higher water content than adult myocardium, but the clinical significance of this is probably minimal. |
Afterload | Newborn myocardium can adapt to mild, nonacute increases in afterload. Afterload may be increased in children with systemic vasoconstriction or pulmonary hypertension (constrictors include alveolar hypoxia, acidosis, hypothermia, and alveolar distention). Some uncorrected congenital heart defects may increase afterload. Coarctation of the aorta and aortic stenosis increase left ventricular afterload. Pulmonary stenosis increases right ventricular afterload. Afterload may be decreased in septic shock. |
Oxygen delivery and consumption | Highest per kilogram body weight during the neonatal period and infancy. The young child requires a higher cardiac output and oxygen delivery per kilogram than the adult. Increased oxygen consumption occurs in critically ill newborns exposed to cold because they cannot shiver to generate heat. Other causes of increased oxygen consumption in children and infants include fever, sepsis, trauma, pain, and seizures. |
VEDP, Ventricular end-diastolic pressure.
Evaluate the child’s blood pressure in light of age and clinical condition; note that shock may be present despite a systolic blood pressure within the normal range for age. When hypovolemic or cardiogenic shock is present, compensatory vasoconstriction may initially maintain the systolic blood pressure, although the mean and diastolic arterial pressures may fall. The development of systolic hypotension in the child with hypovolemic or cardiogenic shock indicates severe shock, and rapid deterioration may follow. In septic shock, by comparison, mild systolic hypotension may develop early in the clinical course. However, in all children, the development of systolic hypotension or a fall in mean arterial pressure indicates hypotensive shock, and urgent treatment is needed.2,3,6
If a central venous catheter is in place, the superior vena cava (SVC) oxygen saturation is monitored to evaluate the balance of oxygen delivery versus consumption. The SVC saturation is central venous oxygen saturation (SCVO2) that is used as a surrogate for mixed venous oxygen saturation that would require a sampling of blood from the pulmonary artery. Normally, the SCVO2 is 25% to 30% below the arterial oxygen saturation. A fall in SCVO2 can indicate a fall in oxygen delivery (caused by decreased arterial oxygen content or decreased cardiac output), an uncompensated rise in oxygen consumption, or both.3
Infants have high glucose needs and low glycogen stores that may be rapidly depleted during stress. For this reason, hypoglycemia (glucose ≤60 mg/dl) may be observed in seriously ill or injured infants and may be associated with cardiovascular or neurologic deterioration.2,11 Hyperglycemia (>150 mg/dl) may develop in critically ill or injured children as the result of a relative insulin-resistant state associated with high levels of endogenous catecholamines and hydrocortisone secretion.3,11 Such hyperglycemia in children is often observed in the first 12 to 18 hours after initial injury or development of shock, then falls to normal levels; persistent hyperglycemia (>180 mg/dl or 10 mmol/L) has been linked with poor survival in critically ill children,12,13 and hypo-, hyperglycemia, and glucose instability (episodes of both hypo- and hyperglycemia in same child) have been linked with development of multiple organ dysfunction.13
Hypovolemic Shock
Hypotension is a sign of severe, decompensated hypovolemic shock and may not develop unless intravascular volume loss is rapid or severe.2,3 Hypotension typically develops with greater than 10% isotonic or hypotonic dehydration in the infant or child (or greater than 6% to 7% dehydration in the adolescent).11 Hypotension may not be observed in the child with trauma unless hemorrhage totals about 20% to 25% of circulating blood volume.14,15
Relative hypovolemia may be caused by a decrease in the intravascular volume relative to the vascular space. This may be associated with the vasodilation of sepsis, anaphylaxis, or neurogenic shock (see following text) or with β2-adrenergic drug toxicity. A relative hypovolemia also may be caused by increased capillary permeability with a redistribution of intravascular volume, such as with burns, anaphylaxis, or sepsis. The translocation of extravascular fluid to a location that is neither intravascular nor intracellular, as in edema, is termed “third spacing” of fluids.11
Compensatory Responses
Significant dehydration, hypovolemia, and low cardiac output stimulate adrenergic and renal compensatory mechanisms characterized by the “fight-or-flight” response. These include tachycardia and redistribution of blood from the skin, gut, and kidney to the brain and heart. Reduced renal perfusion stimulates the renin-angiotensin-aldosterone system, resulting in renal sodium and water retention. Decreased atrial stretch stimulates the secretion of antidiuretic hormone (ADH, also known as arginine vasopressin [AVP]) and produces free water retention by the kidneys.3 These mechanisms are similar in adults and children and may help restore or maintain intravascular volume over time. Neonatal and young infant kidneys, however, are incapable of excreting concentrated urine, so these compensatory mechanisms are relatively ineffective during the first weeks of life.11
Compensatory mechanisms cannot be maintained indefinitely. Systemic vasoconstriction increases left ventricular afterload and myocardial oxygen consumption and may produce tissue ischemia. Prolonged tachycardia may impair subendocardial blood flow and increase myocardial oxygen consumption; both may ultimately contribute to myocardial ischemia.3 Extreme tachypnea increases oxygen demand and reduces effective ventilation. A severe compromise in blood flow and systemic perfusion contributes to cerebral, renal, or hepatic ischemia and possible organ failure.
Clinical Manifestations
The child with hypovolemic shock demonstrates signs of inadequate blood flow to some tissue beds and some evidence of organ system dysfunction (see Box 49-1). The infant or child may be irritable or lethargic. Respirations will be rapid and may be labored if shock is severe or associated with myocardial failure. The skin will be mottled, although pallor also may be observed. A prolonged capillary refill time (>2 seconds) is consistent with the development of shock.2,3,6,16
The child in hypovolemic shock is often tachycardic. Bradycardia often indicates impending cardiovascular collapse or cardiac arrest and is the most common terminal cardiac rhythm observed in children.2,3,10 When hypovolemic shock is present, compensatory vasoconstriction may initially maintain the systolic blood pressure, although the mean arterial pressure may fall. The development of systolic hypotension often indicates severe shock, and rapid deterioration may follow. The CVP is less than 5 to 8 mmHg, unless heart failure or pulmonary hypertension is present.
Clinically significant dehydration is associated with weight loss (Table 49-3). Fluid intake and output records (or reports from parents or primary caretakers) reveal a history of inadequate fluid intake or excessive fluid losses. The child with significant dehydration demonstrates dry mucous membranes, a sunken fontanel (in infants), and poor skin turgor11 (Table 49-4). Moderate isotonic dehydration produces signs of peripheral circulatory compromise, and severe isotonic dehydration will produce hypotension. The blood urea nitrogen (BUN) and urine specific gravity are usually elevated. The serum sodium concentration and osmolality are affected by the type and severity of dehydration present.
TABLE 49-3
TYPE OF DEHYDRATION | CLINICAL INDICATORS |
Isotonic dehydration | Fluid output exceeds intake. Loss of free water is proportional to loss of sodium, so serum sodium concentration remains normal. Fluid loss is from intravascular and extravascular compartments. Compromises peripheral perfusion when the young child has lost approximately 10% (100 ml/kg) of body weight. Compromises systemic perfusion in the adolescent with acute fluid loss equivalent to 5% to 6% of body weight. |
Produces hypotension (decompensated shock) when the young child has lost 15% (150 ml/kg) of body weight. Produces hypotension in the adolescent with a fluid loss equivalent to 7% to 9% of body weight because body water constitutes a smaller percentage of body weight in older children and adults than in young children. | |
Hypotonic/hyponatremic dehydration | Associated with a proportionately greater loss of sodium than free water; thus the serum sodium falls. Resultant acute fall in serum osmolality produces an acute extravascular fluid shift and further loss of extravascular volume. Fluid loss in hypotonic dehydration is primarily from the intravascular compartment; thus a compromise in systemic perfusion will be observed after even small quantities of fluid loss. |
Poor peripheral perfusion occurs in a child with a fluid loss equivalent to 5% (50 ml/kg) of body weight. Adolescents with hyponatremic dehydration may demonstrate a compromise in peripheral perfusion with a fluid loss equivalent to approximately 3% of body weight. | |
Hypotension often is observed when fluid loss is equal to approximately 10% (100 ml/kg) of body weight. Hypotension in an adolescent is observed when the fluid loss equals approximately 5% to 6% of body weight. | |
Hypertonic/hypernatremic dehydration | Free water deficit is proportionately greater than the deficit of sodium, so serum sodium concentration rises, increasing serum osmolality and producing an intravascular shift of free water. For this reason the child with hypernatremic dehydration is likely to maintain intravascular volume and systemic perfusion until relatively large quantities of fluid are lost. |
Compromise in systemic perfusion is not likely to be observed in the child with hypernatremic dehydration until severe dehydration is present with a fluid loss equivalent to 10% of body weight (or 5% to 6% of body weight in the adolescent). | |
Hypotension may not be observed until the fluid loss approximates 15% or more of body weight (7% to 9% or more of body weight in the adolescent). | |
Hypotension in the child with hypertonic/hypernatremic dehydration indicates a substantial fluid deficit. However, the deficit must be replaced carefully to correct shock and avoid rapid lowering of serum sodium concentrations. |
Data in part from Roberts KE: Pediatr Rev 22:380–386, 2001; Roberts KE: Fluid, electrolyte and endocrine problems. In Hazinski MF, editor: Nursing care of the critically ill child, ed 3, St Louis, 2013, Mosby.
TABLE 49-4
SEVERITY OF (ISOTONIC) DEHYDRATION BASED ON CLINICAL EXAM∗
CLINICAL PARAMETERS | MILD | MODERATE | SEVERE |
Body weight loss | |||
Infant | 5% (50 ml/kg) | 10% (100 ml/kg) | 15% (150 ml/kg) |
Adolescent | 3% (30 ml/kg) | 5%-6% (50-60 ml/kg) | 7%-9% (70-90 ml/kg) |
General appearance | Alert, restless, thirsty | Lethargic, postural dizziness | Limp, coma, cold and cyanotic extremities |
Radial pulse | Full | Thready, weak, rapid | Feeble, not palpable |
Respiration | Normal | Deep | Deep, rapid |
Skin elasticity | Pinch retracts immediately | Pinch retracts slowly | Pinch retracts very slowly (>2 seconds) |
Eyes | Normal | Sunken | Very sunken |
Tears | Present | Diminished | Absent |
Mucous membranes | Moist | Dry | Very dry (parched) |
Urine output | Normal | ↓ | ↓ or absent |
Capillary refill time | <2 seconds | >2 seconds | Prolonged |
Heart rate | Varies with age | Varies with age | Varies with age |
Blood pressure | Normal | Normal | Reduced |
∗The interpretation of the assessments must be appropriately modified for age and type of dehydration (hypotonic or hypertonic).
Modified from Perkin RM et al: Shock, cardiac arrest and resuscitation. In Hazinski MF, editor: Nursing care of the critically ill child, ed 3, St Louis, 2013, Mosby.
Hemorrhage is another potential cause of hypovolemic shock. To appreciate the significance of any blood lost or drawn for laboratory analysis, the total blood loss should be considered as a percentage of the child’s circulating blood volume (Table 49-5).
TABLE 49-5
ESTIMATION OF PEDIATRIC CIRCULATING BLOOD VOLUME
AGE OF CHILD | BLOOD VOLUME (ml/kg body weight) |
Newborn | 80-85 |
Infant | 75-80 |
Child | 70-75 |
Adolescent | 65-70 |
From Hazinski MF: Children are different. In Hazinski MF, editor: Nursing care of the critically ill child, ed 3, St Louis, 2013, Mosby.
Tachycardia, peripheral vasoconstriction, and altered level of consciousness may be the only early evidence of hemorrhage in the child with trauma (Table 49-6). Acute blood loss (hemorrhage) may not compromise a child’s peripheral perfusion until an estimated 25% to 30% of intravascular volume is lost (i.e., an acute intravascular or blood loss of 16 to 24 ml/kg).14–16 Once hypotension develops, cardiovascular collapse is imminent and immediate, rapid intravascular volume expansion is required and surgical intervention may be needed.
TABLE 49-6
CLASSIFICATION OF PEDIATRIC HEMORRHAGIC SHOCK IN TRAUMA PATIENTS BASED ON CLINICAL EVALUATION
SYSTEM | MILD HEMORRHAGE, COMPENSATED SHOCK, SIMPLE HYPOVOLEMIA (<30%) | MODERATE HEMORRHAGE, DECOMPENSATED SHOCK, MARKED HYPOVOLEMIA (30%-45%) | SEVERE HEMORRHAGE, CARDIOPULMONARY FAILURE, PROFOUND HYPOVOLEMIA (>45%) |
Cardiovascular | Tachycardia Weak peripheral pulses, strong central pulses Low to normal blood pressure (systolic BP >70 mmHg + [2 × (age in years)]) Mild acidosis | Moderate tachycardia Thready peripheral pulses, weak central pulses Frank hypotension (systolic BP <70 mmHg + [2 × (age in years)]) Moderate acidosis | Severe tachycardia Absent peripheral pulses, thready central pulses Profound hypotension (systolic BP <50 mmHg) Severe acidosis |
Respiratory | Mild tachypnea | Moderate tachypnea | Severe tachypnea |
Central nervous system | Irritable, confused | Agitated or lethargic | Obtunded, comatose |
Skin | Cool extremities, mottling Poor capillary refill (72 sec) | Cool extremities, pallor Delayed capillary refill (72 sec) | Cool extremities, cyanosis Prolonged (>5 sec) capillary refill |
Kidneys | Mild oliguria, increased specific gravity | Marked oliguria, increased blood urea nitrogen (BUN) | Anuria |
From Soud T, Pieper P, Hazinski MF: Pediatric trauma. In Hazinski MF, editor: Nursing care of the critically ill child, ed 2, St Louis, 1992, Mosby.
Signs of neurogenic shock in the child with a recent, severe spinal cord injury include warm skin and hypotension with a low diastolic blood pressure. Signs of poor systemic perfusion also are observed (see Clinical Manifestations of Shock, previously), although loss of sympathetic nervous system tone prevents the typical tachycardic response.
Cardiogenic Shock
1. Following cardiovascular surgery or with inflammatory disease of the heart, such as cardiomyopathy and myocarditis
2. With drug toxicity or severe electrolyte or acid-base imbalances
3. As a complication of any form of shock and early in septic shock
Compensatory Responses
In the early stages of cardiogenic shock, adrenergic compensatory mechanisms produce tachycardia, peripheral vasoconstriction, and constriction of the splanchnic arteries to divert blood flow from the skin, gut, and kidneys to maintain flow to the heart and brain.2,3,16 These compensatory mechanisms may be sufficient to maintain the child’s systolic blood pressure and effective coronary artery and cerebral blood flow. However, tachycardia and systemic arterial constriction increase myocardial oxygen consumption. In addition, reduction in gut and kidney blood flow may produce hepatic, mesenteric, or renal ischemia or failure. Decreased renal perfusion stimulates the renin-angiotensin-aldosterone system, as described for hypovolemic shock.
If the mean arterial pressure or pulse pressure falls, stimulation of the baroreceptors in the carotid sinuses and aortic arch is reduced. This reduced baroreceptor activity removes inhibition from the vasomotor center in the medulla, resulting in increased adrenergic stimulation.3 If myocardial dysfunction progresses, cardiac output and systemic blood pressure ultimately fall. Myocardial ischemia then exacerbates myocardial dysfunction, and multisystem organ failure may result from persistent or severe organ ischemia.
Clinical Manifestations
The child with cardiogenic shock demonstrates signs of inadequate systemic perfusion despite adequate intravascular volume or even relative hypervolemia. This form of shock is generally associated with low cardiac output. The child’s extremities are cool to touch (will cool peripherally to proximally), with delayed capillary refill despite a warm, ambient temperature.2,6,16 The skin may be mottled (see Figure 49-1).
Septic Shock
Sepsis and its complications result from activation of biochemical and physiologic cascades that lead to the formation or activation of cytokines and other mediators that produce vasodilation, increased capillary permeability, maldistribution of blood flow, and cardiovascular dysfunction.3 Sepsis and its complications may result in organ system dysfunction and are leading causes of death in noncoronary intensive care units. An estimated 42,000 pediatric cases of sepsis are reported annually in the United States and result in approximately 4400 deaths per year.17
Watson’s 2003 hospital data from seven states17 still provides the most detailed information regarding causes of pediatric sepsis in the United States. The most common sites of infection from these data were primary bloodstream (25%) and respiratory tract (37%) infections;17 about half of children with sepsis had an underlying disease and more than one fifth were low–birth-weight neonates.17,18
Many bloodstream and respiratory infections may be prevented with proper handwashing by healthcare providers before and after contact with children, appropriate sterile and aseptic technique during catheter insertion and tubing changes, and use of protocols to reduce central venous catheter infections and ventilator-associated pneumonias.19,20 More pediatric data are needed to develop evidence-based recommendations to reduce hospital-acquired infections and sepsis.
The microorganisms that cause sepsis vary according to age, immune function, and location (e.g., in the hospital versus in the community).21 Approximately 40% of all hospital-acquired infections in adults and children are linked to gram-negative infections; 40% to gram-positive infections; and 20% to viruses, fungi, or rickettsial microorganisms.18 Prevention of hospital-acquired infections can substantially reduce the risk of sepsis and its complications.19,20 Clinicians must be aware of the common pathogens in various populations.
Factors associated with risk for the development of sepsis include extremes of age (infants, young children and older adults)3,17; invasive catheters, surgical incisions, or wounds or burns; immunocompromise; and long-term antibiotic therapy.17,18 Many of these risk factors are present in seriously ill or injured children and children with a chronic disease.
It is now clear that genetic variations among children and among invading microorganisms can alter the outcome of infections, sepsis, and septic shock.21 Genetic variation in any of several genes that affect host responses to invading microorganisms can influence outcomes of infections.22 Certainly, infectious diseases, such as meningococcal disease, can vary widely in their invasiveness and in the severity of effects on the host.23 It is anticipated that genetic information will ultimately influence identification of a child’s susceptibility to infection and sepsis, risk modification, early detection of sepsis, tailoring of therapy, and prognostication.22
Both proinflammatory and anti-inflammatory cytokines serve essential protective functions in fighting infection and modulating the immune response. Sepsis is caused by the effects of the invading microorganism and its toxins, but most effects that contribute to shock result from a disruption in the balance between proinflammatory mediators (including tumor necrosis factor-alpha [TNF-α], interleukin [IL]-1, IL-6, and IL-8; platelet-activating factor; arachidonic acid metabolites; nitric oxide; and many kinins) and anti-inflammatory mediators (IL-4, IL-10, IL-11, and IL-13; transforming growth factor-beta; colony-stimulating factors; soluble tumor necrosis factor receptor; IL-1 receptor antagonist; and activated protein C).3 Extremely high levels of proinflammatory mediators, such as TNF, nitric oxide, and platelet-activating factor can become destructive, even after the triggering microorganism is eradicated.3,21
High proinflammatory cytokine levels have been implicated in the development of sepsis-induced disseminated intravascular coagulation, pulmonary injury, and microcirculatory disruptions, such as are observed in burns, severe trauma, shock reperfusion syndromes, and MODS.21 Tumor necrosis factor levels have been directly related to mortality in newborns and children with meningitis and sepsis,24 and interleukin-8 levels have been shown to be reliable predictors of pediatric sepsis mortality.22
Increased nitric oxide concentrations are thought to contribute to vasodilation, hypotension, and decreased myocardial function that develop during adult sepsis. In comparison, infants with sepsis often demonstrate pulmonary vasoconstriction resulting from shock-induced acidosis and hypoxia, and may benefit from administration of inhaled nitric oxide or other vasodilators.25
During sepsis, endotoxin stimulates the endothelium to become a secretory organ. The endothelium changes from the normal profibrinolytic and anticoagulant state to an antifibrinolytic and precoagulant one.25 This change can lead to the development of microthrombin in some areas of the microcirculation, further contributing to maldistribution of blood flow. Mediators (e.g., activated protein C) that regulate coagulation pathways have been implicated in the sepsis process.25–27 Activated protein C also regulates inflammation and activated protein C deficiency is a marker for severe sepsis in all ages.27 Although administration of activated protein C improved survival in adults with severe sepsis,28 it has not been shown to improve outcome in children and may produce excessive bleeding complications.25
There is clear interaction among catecholamines, adrenoreceptors, and glucocorticoids. Endogenous glucocorticoids have an anti-inflammatory effect (they decrease activation of proinflammatory mediators), and they modulate vasomotor tone by enhancing cardiovascular and vasomotor response to catecholamines.29
Critically ill children may have adrenal insufficiency (caused by adrenal hemorrhage, decreased renal perfusion, inhibition of corticosteroid production by TNF, or actual adrenal disease) or a relative adrenal insufficiency, with inadequate adrenal stress response or decreased response to circulating glucocorticoids.30,31 When sepsis is present and the child fails to respond to initial fluid therapy and vasoactive support, providers should rule out adrenal insufficiency and consider hydrocortisone administration, especially if risk of adrenal insufficiency is present.32
