html xmlns=”http://www.w3.org/1999/xhtml” xmlns:epub=”http://www.idpf.org/2007/ops”>
11Scale-Up and Transfer of Hard Shell Formulations across Machine Types
Larry L. Augsburger and Michael Levin
Scaling Up within the Same Design and Operating Principle
Regulatory Meaning of Same Design and Operating Principle
Scaling Up within the Same Subclass
Transferring between Dosator and Dosing Disc Machines
Bulk Density and Densification
Introduction
Scale-up of any physical process implies increasing the batch size or a procedure for applying the same process to different output volumes. Once you understand what makes these processes of different scales similar, you can eliminate many scale-up problems. A rational approach to scale-up has been used in physical sciences, viz., fluid dynamics and chemical engineering, for quite some time. This approach is based on process similarities between different scales and uses dimensional analysis that was developed a century ago and has since gained wide recognition in many industries, especially in chemical engineering.1 This approach is warrantied when there is a geometric, kinematic, and dynamic similarity between processes of different scales.
In encapsulation, as well as in tableting unit operations, batch size enlargement does not mean a size increase of the processing volume. In both tableting and capsule-filling applications, the process scale-up involves production in what is essentially the same unit volume (die cavity or capsule size). However, unlike tableting, encapsulation scale-up is generally achieved by means of increasing the number of processing units rather than the speed of operation. Since the size of each unit volume is fixed, one of the conditions of the theory of models (similar geometric space) is partially met when using similar equipment. However, there are still kinematic and dynamic parameters that need to be investigated and matched for any scale-up or process transfer. Technology transfer to machines of a different type presents another difficulty as the procedures to maintain quality are mainly empirical. One way to eliminate potential scale-up or technology transfer problems is to develop formulations that are very robust with respect to processing conditions.
Scale-up problems may require post-approval changes that affect formulation composition, site change, and manufacturing process or equipment changes (by the way, from the regulatory standpoint, scale-up and scale-down are treated with the same degree of scrutiny). In a typical drug development cycle, once a set of clinical studies have been completed or NDA/ANDA (New Drug Application/Abbreviated New Drug Application) has been approved, it becomes very difficult to change the product or the process to accommodate specific production needs. Such needs may include changes in batch size and manufacturing equipment or process. Post-approval changes in the size of a batch from the pilot scale to larger or smaller production scales call for submission of additional information in the application, with a specific requirement that the new batches are to be produced using similar equipment and in full compliance with current good manufacturing practices (CGMPs) and the existing standard operating procedures (SOPs). Manufacturing changes may require new stability, dissolution, and in vivo bioequivalence testing. This is especially true for Level 2 equipment changes (change in equipment to a different design and different operating principles) and the process changes of Level 2 (process changes including changes such as mixing times and operating speeds within application/validation ranges) and Level 3 (change in the type of process used in the manufacture of the product, such as a change from wet granulation to direct compression of dry powder).
If the process is different after scale-up, the company has to demonstrate that the product produced by a modified process will be equivalent, stability studies and dissolution profiles. Of practical importance are the issues associated with a technology transfer in a global market. Equipment standardization inevitably will cause a variety of engineering and process optimization concerns that, generally speaking, can be classified as SUPAC (scale-up and post-approval changes). In what follows, we will address these issues in detail.
Scaling Up within the Same Design and Operating Principle
Regulatory Meaning of Same Design and Operating Principle
The current (1999) guidance, “SUPAC-IR/MR: Immediate Release and Modified Release Solid Oral Dosage Forms Manufacturing Equipment Addendum”2 applies to both immediate- and modified-release oral solid dosage forms. It was developed by the US Food and Drug Administration (FDA) with the assistance of the International Society of Pharmaceutical Engineering (ISPE) and is an update of earlier documents, SUPAC-IR (1995)3 and SUPAC-MR (1997).4 This guidance is organized in broad categories of unit operations (e.g., blending, drying, granulation, etc.). For each unit operation, a table is provided in which equipment is classified by class (operating principle) and subclass (design characteristic). Generally, equipment within the same class and subclass are considered to have the same design and operating principle under SUPAC-IR (1995) and SUPAC-MR (1997). A change from equipment in one class to equipment in a different class would usually be considered a change in design and operating principle.
The SUPAC Guidances classify changes according to their level. The documentation and reporting requirements also vary according to the level of changes. Scale up to and including a factor of 10 times the size of the pilot/bio-batch is considered a Level 1 change provided the equipment used to produce the test batch(es) is of the same design and operating principles, CGMPs are followed, and the same SOPs, controls, and formulation and manufacturing procedures are used. Level 1 changes are not likely to cause any detectable impact on formulation quality or performance and need only be reported in the annual report.
Scale-up beyond 10 times the pilot/bio-batch is a Level 2 change provided the equipment used to produce the test batch(es) is of the same design and operating principles and the same SOPs, controls, and formulation and manufacturing procedures are used. Level 2 changes are defined as those changes that could significantly affect formulation quality and performance and require the filing of “changes being effected supplement.” Interestingly, neither case requires in vivo bioequivalence data to support the change of scale. The guidance should be consulted for a full description of the test documentation needed to be reported to FDA in these cases.
However, equipment changes to a different subclass within the same class should be carefully considered and evaluated on a case-by-case basis. By definition, encapsulating, or the “division of material into a hard gelatin capsule,” is an operating principle. That is, all encapsulators should have in common rectification, separation of caps from bodies, dosing of fill material/formulation, rejoining of caps and bodies, and ejection of the filled capsules. Nevertheless, the various methods of dosing the formulation represent different design characteristics and are considered subclasses. Thus, a change from a dosator machine to a dosing disc machine would constitute a change in subclass, but not of operating principle.
According to a survey of industry practices, 64% of companies develop formulations using small-scale equipment of the same design and operating principles as the production equipment, whereas about 18% of the responding companies indicated that they use small-scale development equipment of a subclass different from the intended production equipment.5 Preferences among the companies appeared about evenly divided between dosator and dosing disc machines, with about 18% using both machine types. In the following sections, we will address some of the issues involved in scaling up or transferring formulations within and between these subclasses.
Scaling Up within the Same Subclass
Generally, there should be minimal problems when changing scale within the same subclass. Higher-output plug-forming machines of the same manufacturer’s series typically do not make plugs any faster than the lower-output machines. Higher throughput is primarily achieved in these machines by increasing the number of dosing units. Nevertheless, there are factors that formulators should take into account when make making such scale changes. For example, the greater diameter and turning radius of the powder bowl of larger dosing disc machines may need to be considered. To minimize weight variation in such machines, there may be an optimal flow criterion that should be met, and that criterion may vary with the turning radius. Kurihara and Ichikawa6 studied the relationship between the angle of repose, weight variation, and the degree of acceleration that takes place in disc movement, which, in turn, depends on dosing disc diameter and rotational speed. They reported a minimum point in the plot of the angle of repose versus coefficient of variation of filling weight for a dosing disc machine (Höfliger Karg GKF 1000). This observation indicates that powder is distributed over the dosing disc by the centrifugal action of the indexing rotation (baffles are provided to help maintain a uniform powder level). It means that powders with sufficiently high angles of repose may not have sufficient mobility to distribute well over the dosing disc via the intermittent indexing motion, whereas powders having angles of repose that are sufficiently low may be too fluid to maintain a uniform bed. Working with an instrumented GKF model 330, Shah et al.7 observed that a uniform powder bed height was not maintained at the first tamping station because of its proximity to the scrape-off device adjacent to ejection. More recent workers have also pointed to the need to properly calibrate the flow properties of formulations for this type of filling machine. Heda et al.8 studied several model formulations, each having different flow properties, on a GKF 400 machine. They proposed that Carr Compressibility Index (CI) values should be in the range of 18–30% to maintain low weight variation, which agrees well with Podczeck and Newton9 who reported an optimum range of 15–30% based on a study of various materials filled on a GKF 400S machine. More poorly flowing powders (CI > 30%) were found by Heda et al. to dam up around the ejection station. Podczeck10 reported that poor flowing powders display an “avalanching behavior” in front of the ejection station; that is, the powder mass alternatively builds up and collapses in the manner of an avalanche at certain intervals of time such that the powder mass over stations 1 and 2 can vary dramatically and cause increased capsule fill weight variation.
Nalluri et al.11 found weight variability to correlate with the angle of internal friction and the avalanche energy of blends of acetaminophen and microcrystalline cellulose 102 filled on a Harro Höfliger KFM III-C dosing disc machine. Beyond about 75% of drug loading, variability increased and changed differently as a function of drug level. This threshold level was also predicted by percolation theory and is considered the point beyond which flowability becomes dominated by the drug.
The MG-2 series of continuous motion dosator machines also exhibit similar plug-forming parameters across machines with different production capacities. To illustrate this point, one can compare the 16-station (rated up to 48,000 capsules per hour [cph]) and 64-station machines (rated up to 200,000 cph). These two machines are reported to operate at the same revolutions per minute (RPM), have the same spacing between dosators, and have similar dosator penetration and withdrawal speeds, plug compression dwell times, and plug ejection speeds.12 The powder bed into which dosators dip to form plugs is scraped off neatly to a specific bed height and is presented in an annular ring (the “powder trough”) that rotates with the dosator turret (dosing head). Because the powder bed and dosing head rotate at slightly different speeds, a plug is not formed from the same location in the powder bed until approximately 8–10 revolutions have occurred when the bed is presumed to have been restored and stabilized.12 Maintaining a uniform powder bed density is critical for dosators to pick up uniform weights of powder in all cases. Contributing to plug uniformity in MG2 machines is the fact that the dosators in these machines do not punch vertical holes through the bed. The difference in rotational speeds between the turret and the powder trough also causes the dosators to enter the powder bed at an angle. The axes of rotation of the annular powder bed and the dosing head are offset such that as the turret rotates, dosators alternatively pass over the annular powder trough where they form plugs and then over bushings bearing capsule bodies where they eject the plugs.
With the MG-2 Futura series of machines, formulations can be scaled up within the same machine by changing the number of dosators to yield outputs ranging from 6,000 to 96,000 cph. Since the turret and annular powder bed dimensions are unchanged, few problems should be expected when scaling up within this range. However, when fewer than the full complement of 16 dosators are installed, the dwell time of powder in the trough and feed mechanism will be longer compared to the fully tooled machine.
Differences in dwell time in the feed mechanism of MG-2 machines have been reported to affect dissolution and content uniformity. For instance, Desai et al.13 found the dissolution rates of three drugs of varying solubility to slow after 30 or 45 min of running on an MG-2 machine fitted with four dosators. This slowdown, which was most apparent with the least-soluble drug, was attributed to increased coating of particles with hydrophobic magnesium stearate as a result of prolonged mixing by the propeller blade in the hopper. Desai et al. carried out a simulation in which the capsule machine was run for up to 60 min without filling capsules. During this time, the propeller blade continued to mix the formulation in the hopper. Capsules were hand filled (to eliminate possible confounding effects of plug compression) from the powder initially and at the end of a run. After 60 min, dissolution from capsules filled from the hopper was significantly reduced from that of capsules filled before the start of the run.
The positive results of additional studies carried out by Desai et al. supported various formulation changes that may ameliorate the problem, for example replacing magnesium stearate with more hydrophilic lubricants (Stear-O-Wet or sodium stearyl fumarate), reducing the level of magnesium stearate, and replacing pre-gelatinized starch (10% in the subject formula) with equal concentrations of a super disintegrant (Explotab or Primojel).13
In another example, Johansen et al.14 reported both a segregation problem and an overmixing problem on an MG-2 model G36/4 machine during a trial production run of 25-mg indomethacin capsules. The formulation was mixed using a planetary mixer, with magnesium stearate being added separately and mixed for 5 min. Both drug content and dissolution were found to vary with time during the run. They concluded that the powder throughput was so slow at the mixing blade rotational velocity of 1 RPM that overmixing occurred. Removing the mixing blade and fitting the hopper with an insert to convert it to a mass flow hopper apparently solved both problems.
As a predictive stress test to determine the likelihood of encountering a dissolution problem on scale-up attributed to lubricant overmixing, Harding et al.15 proposed the use of a Turbula high-intensity mixer. They reported a qualitative relationship between changes in the properties of a 500-g batch caused by mixing in a 2-L Turbula for 6 h and the effects of a 6-h run of a 10-kg batch of the same formulation in a Zanasi LZ-64 intermittent motion dosator machine.
Air compressors, vacuum pumps, and the drive motor all contribute to filling machine vibration and the greater vibration realized at higher running speeds may increase powder density and fill weight in dosator machines. Llusa et al.16 fitted a laser Doppler vibrometer to a research MG2 machine (Labby). They found that machine vibrations were not significant at a running speed of 500 cph, and powder density was not measurably affected. At 3000 cph, vibration and powder bed densification were significant and fill weight was significantly increased. The study was limited to a single capsule size (#3) and the filling of several grades of microcrystalline cellulose. No lubricants (i.e., magnesium stearate) were included, which could have modified their findings owing to the ability of magnesium stearate often to enhance the initial packing density of powder blends and form denser plugs, including those formed from microcrystalline cellulose. For example, Shah et al.7 found the bulk and tapped densities of microcrystalline cellulose (Avicel PH 101) to increase with the percent magnesium stearate within the concentration range of 0.1–0.5%. The weight and breaking strength of the plugs formed from these lubricated blends on an instrumented H&K 330 dosing disc machine were greater than were found with the unlubricated filler. The 0.1% level appeared optimal in that it resulted in maximum weight and plug breaking strength. At the 0.25% and 0.5% levels, breaking strength decreased with magnesium stearate concentration, but weight exhibited little change. The lubricant effects that contribute to plug breaking strength and those that tend to soften plugs appear competitive, with those contributing to softening dominating at the higher magnesium stearate levels. In another example using a tabletop plug-forming device, Jones17 found that plug density increased with mixing time when 1% magnesium stearate was added to either microcrystalline cellulose (Avicel PH 102) or pre-gelatinized starch (Starch 1500). Such densified formulations may have limited ability to undergo further vibrational densification in the machine. Nevertheless, the direct observation of significantly increased vibratory consolidation of a powder bed at the high running speed is a significant finding for dosator machines for which powder bed density is a significant determinant of fill weight. However, the significance of this finding should not be limited to dosator machines since vibratory consolidation can occur in both dosator and dosing disc machines. Considering the operational characteristics of these machines, vertical vibration may be more important in dosing disc machines. Beyond effects on fill weight, increased vibration at higher speeds could also encourage segregation in susceptible formulations. The reader is referred to Lin et al.18 for a useful analysis of the relationship between bulk powder properties and the segregation tendency of powder formulations from a Quality by Design perspective.
Differences in various parameters may be encountered when scaling up within the same subclass but across different manufacturers of the equipment, which have the potential to affect the success of the scale change. For example, using a filling machine simulator, Britten et al.19 found that plug ejection speed had little effect on plug properties, but higher compression speeds led to reduced plug consolidation and lower weights. Machines of the same subclass produced by different manufactures may exhibit differences in powder handling, mixing mechanisms, and throughput rates that could cause formulations to perform differently.
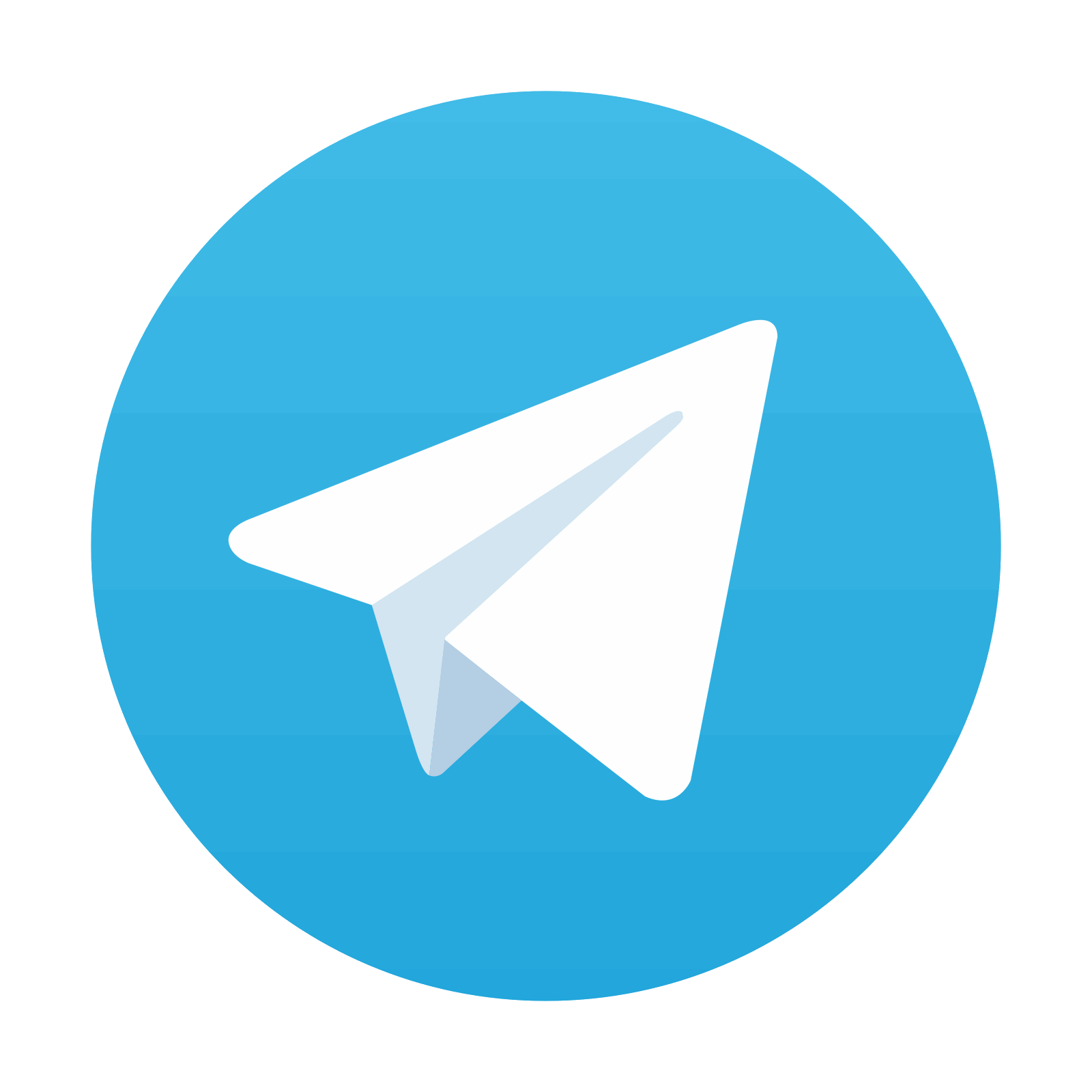
Stay updated, free articles. Join our Telegram channel

Full access? Get Clinical Tree
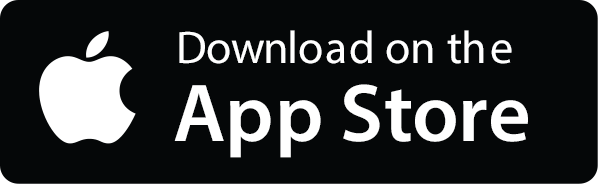
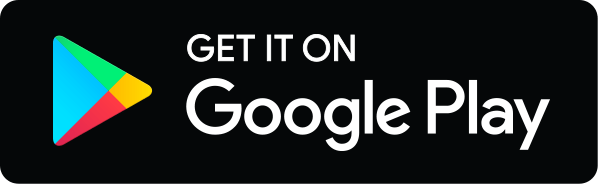