Purines and pyrimidines are required for synthesizing nucleotides and nucleic acids. These molecules can be synthesized either from scratch, de novo, or salvaged from existing bases. Dietary uptake of purine and pyrimidine bases is low because most of the ingested nucleic acids are metabolized by the intestinal epithelial cells.
The de novo pathway of purine synthesis is complex, consisting of 11 steps and requiring six molecules of adenosine triphosphate (ATP) for every purine synthesized. The precursors that donate components to produce purine nucleotides include glycine, ribose 5-phosphate, glutamine, aspartate, carbon dioxide, and N10-formyltetrahydrofolate (N10-formyl-FH4) (Fig. 39.1). Purines are synthesized as ribonucleotides, with the initial purine synthesized being inosine monophosphate (IMP). Adenosine monophosphate (AMP) and guanosine monophosphate (GMP) are each derived from IMP in two-step reaction pathways.
FIGURE 39.1 Origin of the atoms of the purine base. FH4, tetrahydrofolate; RP, ribose 5′-phosphate. FH4, tetrahydrofolate; RP, ribose 5′-phosphate.
The purine nucleotide salvage pathway allows free purine bases to be converted into nucleotides, nucleotides into nucleosides, and nucleosides into free bases. Enzymes included in this pathway are AMP and adenosine deaminase (ADA), adenosine kinase, purine nucleoside phosphorylase, adenine phosphoribosyltransferase (APRT), and hypoxanthine–guanine phosphoribosyltransferase (HGPRT). Mutations in a number of these enzymes lead to serious diseases. Deficiencies in purine nucleoside phosphorylase and ADA lead to immunodeficiency disorders. A deficiency in HGPRT leads to Lesch–Nyhan syndrome. The purine nucleotide cycle, in which aspartate carbons are converted to fumarate to replenish tricarboxylic acid (TCA)-cycle intermediates in working muscle, and the aspartate nitrogen is released as ammonia, uses components of the purine nucleotide salvage pathway.
Pyrimidine bases are first synthesized as the free base and then converted to a nucleotide. Aspartate and carbamoyl phosphate form all components of the pyrimidine ring. Ribose 5-phosphate, which is converted to 5′-phosphoribosyl-1′-pyrophosphate (PRPP), is required to donate the sugar phosphate to form a nucleotide. The first pyrimidine nucleotide produced is orotidine monophosphate (OMP). The OMP is converted to uridine monophosphate (UMP), which becomes the precursor for both cytidine triphosphate (CTP) and deoxythymidine monophosphate (dTMP) production.
The formation of deoxyribonucleotides requires ribonucleotide reductase activity, which catalyzes the reduction of ribose on nucleotide diphosphate substrates to 2′-deoxyribose. Substrates for the enzyme include adenosine diphosphate (ADP), guanosine diphosphate (GDP), cytidine diphosphate (CDP), and uridine diphosphate (UDP). Regulation of the enzyme is complex. There are two major allosteric sites. One controls the overall activity of the enzyme, whereas the other determines the substrate specificity of the enzyme. All deoxyribonucleotides are synthesized using this one enzyme.
The regulation of de novo purine nucleotide biosynthesis occurs at four points in the pathway. The enzymes PRPP synthetase, amidophosphoribosyltransferase, IMP dehydrogenase, and adenylosuccinate synthetase are regulated by allosteric modifiers, as they occur at key branch points through the pathway. Pyrimidine synthesis is regulated at the first committed step, which is the synthesis of cytoplasmic carbamoyl phosphate, by the enzyme carbamoyl phosphate synthetase II (CPSII).
Purines, when degraded, cannot generate energy, nor can the purine ring be substantially modified. The end product of purine ring degradation is uric acid, which is excreted in the urine. Uric acid has limited solubility, and if it were to accumulate, uric acid crystals would precipitate in tissues of the body that have a reduced temperature (such as the big toe). This condition of acute painful inflammation of specific soft tissues and joints is called gout. Pyrimidines, when degraded, however, give rise to water-soluble compounds, such as urea, carbon dioxide, and water and do not lead to a disease state if pyrimidine catabolism is increased.
THE WAITING ROOM 
The initial acute inflammatory process that caused Lotta T. to experience a painful attack of gouty arthritis responded quickly to colchicine therapy (see Chapter 10). Several weeks after the inflammatory signs and symptoms in her right great toe subsided, Lotta T. was placed on allopurinol (while continuing colchicine), a drug that reduces uric acid synthesis. Her serum uric acid level gradually fell from a pretreatment level of 9.2 mg/dL into the normal range (2.5 to 8.0 mg/dL). She remained free of gouty symptoms when she returned to her physician for a follow-up office visit.
I. Purines and Pyrimidines
As has been seen in previous chapters, nucleotides serve numerous functions in different reaction pathways. For example, nucleotides are the activated precursors required for DNA and RNA synthesis. Nucleotides form the structural moieties of many coenzymes (examples include reduced nicotinamide adenine dinucleotide [NADH], flavin adenine dinucleotide [FAD], and coenzyme A). Nucleotides are critical elements in energy metabolism (adenosine triphosphate [ATP], guanosine triphosphate [GTP]). Nucleotide derivatives are frequently activated intermediates in many biosynthetic pathways. For example, UDP-glucose and CDP-diacylglycerol are precursors of glycogen and phosphoglycerides, respectively. S-adenosylmethionine carries an activated methyl group. In addition, nucleotides act as second messengers in intracellular signaling (e.g., cyclic adenosine monophosphate [cAMP], cyclic guanosine monophosphate [cGMP]). Finally, nucleotides and nucleosides act as metabolic allosteric regulators. Think about all of the enzymes that have been studied that are regulated by levels of ATP, ADP, and AMP.
Dietary uptake of purine and pyrimidine bases is minimal. The diet contains nucleic acids, and the exocrine pancreas secretes deoxyribonuclease and ribonuclease, along with the proteolytic and lipolytic enzymes. This enables digested nucleic acids to be converted to nucleotides. The intestinal epithelial cells contain alkaline phosphatase activity, which converts nucleotides to nucleosides. Other enzymes within the epithelial cells tend to metabolize the nucleosides to uric acid (which is released into the circulation), or to salvage them for their own needs. Approximately 5% of ingested nucleotides make it into the circulation, either as the free base or as a nucleoside. Because of the minimal dietary uptake of these important molecules, de novo synthesis of purines and pyrimidines is required.
II. Purine Biosynthesis
The purine bases are produced de novo by pathways that use amino acids as precursors and produce nucleotides. Most de novo synthesis occurs in the liver (Fig. 39.2), and the nitrogenous bases and nucleosides are then transported to other tissues by red blood cells. The brain also synthesizes significant amounts of nucleotides. Because the de novo pathway requires at least six high-energy bonds per purine produced, a salvage pathway, which is used by many cell types, can convert free bases and nucleosides to nucleotides.
FIGURE 39.2 Overview of purine production, starting with glutamine, ribose 5-phosphate, and ATP. The steps that require ATP are also indicated in this figure. ADP, adenosine diphosphate; AMP, adenosine monophosphate; ATP, adenosine triphosphate; dADP, deoxyadenosine diphosphate; dATP, deoxyadenosine triphosphate; dGDP, deoxyguanosine diphosphate; dGTP, deoxyguanosine; triphosphate; FH4, tetrahydrofolate; GMP, guanosine monophosphate; GTP, guanosine triphosphate; IMP, inosine monophosphate; PRPP, 5-phosphoribosyl 1-pyrophosphate; RR, ribonucleotide reductase.
A. De Novo Synthesis of the Purine Nucleotides
1. Synthesis of Inosine Monophosphate
As purines are built on a ribose base (see Fig. 39.2), an activated form of ribose is used to initiate the purine biosynthetic pathway. 5′-Phosphoribosyl-1′-pyrophosphate (PRPP) is the activated source of the ribose moiety. It is synthesized from ATP and ribose 5′-phosphate (Fig. 39.3), which is produced from glucose through the pentose phosphate pathway (see Chapter 27). The enzyme that catalyzes this reaction, PRPP synthetase, is a regulated enzyme (see Section II.A.5); however, this step is not the committed step of purine biosynthesis. PRPP has many other uses, which are described as the chapter progresses.
FIGURE 39.3 Synthesis of PRPP. Ribose 5-phosphate is produced from glucose by the pentose phosphate pathway. AMP, adenosine monophosphate; ATP, adenosine triphosphate; PRPP, 5-phosphoribosyl 1-pyrophosphate.
In the first committed step of the purine biosynthetic pathway, PRPP reacts with glutamine to form 5′-phosphoribosyl 1′-amine (Fig. 39.4). This reaction, which produces nitrogen 9 of the purine ring, is catalyzed by glutamine phosphoribosylamidotransferase, a highly regulated enzyme.
FIGURE 39.4 The first step in purine biosynthesis. The purine base is built on the ribose moiety. The availability of the substrate PRPP is a major determinant of the rate of this reaction. PPi, pyrophosphate; PRPP, 5-phosphoribosyl 1-pyrophosphate.
In the next step of the pathway, the entire glycine molecule is added to the growing precursor. Glycine provides carbons 4 and 5 and nitrogen 7 of the purine ring (Fig. 39.5). This step requires energy in the form of ATP.
FIGURE 39.5 Incorporation of glycine into the purine precursor. The ATP is required for the condensation of the glycine carboxylic acid group with the 1′-amino group of phosphoribosyl 1-amine. ADP, adenosine diphosphate; ATP, adenosine triphosphate; Pi, inorganic phosphate.
Subsequently, carbon 8 is provided by N10-formyltetrahydrofolate (N10-formyl-FH4), nitrogen 3 by glutamine, carbon 6 by CO2, nitrogen 1 by aspartate, and carbon 2 by N10-formyl-FH4 (see Fig. 39.1). Note that six high-energy bonds of ATP are required (starting with ribose 5-phosphate) to synthesize the first purine nucleotide, IMP. This nucleotide contains the base hypoxanthine joined by an N-glycosidic bond from nitrogen 9 of the purine ring to carbon 1 of the ribose (Fig. 39.6). Hypoxanthine is not found in DNA, but it is the precursor for the other purine bases. However, hypoxanthine is found in the anticodon of tRNA molecules (see Chapter 14) and is a critical component for allowing wobble base pairs to form.
FIGURE 39.6 Structure of IMP. The base is hypoxanthine. IMP. inosine monophosphate.
2. Synthesis of Adenosine Monophosphate
IMP serves as the branch point from which both adenine and guanine nucleotides can be produced (see Fig. 39.2). Adenosine monophosphate is derived from IMP in two steps (Fig. 39.7). In the first step, aspartate is added to IMP to form adenylosuccinate, a reaction similar to the one catalyzed by argininosuccinate synthetase in the urea cycle. Note that this reaction requires a high-energy bond, donated by GTP. Fumarate is then released from the adenylosuccinate by the enzyme adenylosuccinate lyase to form AMP. This is similar to the aspartate-to-fumarate conversion seen in the urea cycle (see Chapter 38). In both cases, aspartate donates a nitrogen to the product, whereas the carbons of aspartate are released as fumarate.
FIGURE 39.7 The conversion of IMP to AMP. Note that GTP is required for the synthesis of AMP. The first enzyme is adenylosuccinate synthetase; the second enzyme is adenylosuccinate lyase. AMP, adenosine monophosphate; GTP, guanosine triphosphate; IMP, inosine monophosphate; GDP, guanosine diphosphate; Pi, inorganic phosphate; R5P, ribose 5-phosphate.
3. Synthesis of Guanosine Monophosphate
GMP is also synthesized from IMP in two steps (Fig. 39.8). In the first step, the hypoxanthine base is oxidized by IMP dehydrogenase to produce the base xanthine and the nucleotide xanthosine monophosphate (XMP). Glutamine then donates the amide nitrogen to XMP to form GMP in a reaction that is catalyzed by GMP synthetase. This second reaction requires energy, in the form of ATP.
FIGURE 39.8 The conversion of IMP to GMP. Note that ATP is required for the synthesis of GMP. AMP, adenosine monophosphate; ATP, adenosine triphosphate; GMP, guanosine monophosphate; IMP, inosine monophosphate; NAD, nicotinamide adenine dinucleotide; PPi, pyrophosphate; R5P, ribose 5-phosphate; XMP, xanthosine monophosphate.
4. Phosphorylation of AMP and GMP
AMP and GMP can be phosphorylated to the diphosphate and triphosphate levels. The production of nucleoside diphosphates requires specific nucleoside monophosphate kinases, whereas the production of nucleoside triphosphates requires nucleoside diphosphate kinases, which are active with a wide range of nucleoside diphosphates. The purine nucleoside triphosphates are also used for energy-requiring processes in the cell and also as precursors for RNA synthesis (see Fig. 39.2).
5. Regulation of Purine Synthesis
Regulation of purine synthesis occurs at several sites (Fig. 39.9). Four key enzymes are regulated: PRPP synthetase, amidophosphoribosyltransferase, adenylosuccinate synthetase, and IMP dehydrogenase. The first two enzymes regulate IMP synthesis; the last two regulate the production of AMP and GMP, respectively.
FIGURE 39.9 The regulation of purine synthesis. PRPP synthetase can be inhibited by ADP or GDP binding to a single allosteric site. Glutamine phosphoribosylamidotransferase contains adenine nucleotide– and guanine nucleotide–binding sites; the monophosphates are the most important, although the diphosphates and triphosphates will also bind to and inhibit the enzyme. Adenylosuccinate synthetase is inhibited by AMP; IMP dehydrogenase is inhibited by GMP. ADP, adenosine diphosphate; AMP, adenosine monophosphate; ATP, adenosine triphosphate; GDP, guanosine diphosphate; GTP, guanosine triphosphate; PRPP, 5-phosphoribosyl 1-pyrophosphate; XMP, xanthosine monophosphate.
A primary site of regulation is the synthesis of PRPP. PRPP synthetase is negatively affected by GDP and ADP. Thus, the binding of either an oxypurine (e.g., GDP) or an aminopurine (e.g., ADP) can occur, with the result being an inhibition of the enzyme. This enzyme is not the committed step of purine biosynthesis; PRPP is also used in pyrimidine synthesis and both the purine and pyrimidine salvage pathways.
The committed step of purine synthesis is the formation of 5′-phosphoribosyl 1′-amine by glutamine phosphoribosylamidotransferase. This enzyme is strongly inhibited by GMP and AMP (the end products of the purine biosynthetic pathway) by binding to distinct allosteric sites. The enzyme is also inhibited by the corresponding nucleoside diphosphates and triphosphates, but under cellular conditions, these compounds probably do not play a central role in regulation. The active enzyme is a monomer of 133,000 Da but is converted to an inactive dimer (270,000 Da) by binding of the end products. Cellular concentrations of PRPP and glutamine are usually below their Km for glutamine phosphoribosylamidotransferase. Thus, any situation that leads to an increase in their concentration can lead to an increase in de novo purine biosynthesis.
The enzymes that convert IMP to XMP and adenylosuccinate are both regulated. GMP inhibits the activity of IMP dehydrogenase, and AMP inhibits adenylosuccinate synthetase. Note that the synthesis of AMP is dependent on GTP (of which GMP is a precursor), whereas the synthesis of GMP is dependent on ATP (which is made from AMP). This serves as a type of positive regulatory mechanism to balance the pools of these precursors. When the levels of ATP are high, GMP will be made; when the levels of GTP are high, AMP synthesis will take place. GMP and AMP act as negative effectors at these branch points, a classic example of feedback inhibition.
B. Purine Salvage Pathways
Most of the de novo synthesis of the bases of nucleotides occurs in the liver, and to some extent in the brain, neutrophils, and other cells of the immune system. Within the liver, nucleotides can be converted to nucleosides or free bases, which can be transported to other tissues via the red blood cells in the circulation. In addition, the small amounts of dietary bases or nucleosides that are absorbed also enter cells in this form. Thus, most cells can salvage these bases to generate nucleotides for RNA and DNA synthesis. For certain cell types, such as lymphocytes, the salvage of bases is the major form of nucleotide generation.
The overall picture of salvage is shown in Figure 39.10. The pathways allow free bases, nucleosides, and nucleotides to be easily interconverted. The major enzymes required are purine nucleoside phosphorylase, phosphoribosyltransferases, and deaminases.
FIGURE 39.10 Salvage of bases. The purine bases hypoxanthine and guanine react with PRPP to form the nucleotides inosine and guanosine monophosphate, respectively. The enzyme that catalyzes the reaction is HGPRT. Adenine forms AMP in a reaction that is catalyzed by APRT. Nucleotides are converted to nucleosides by 5′-nucleotidase. Free bases are generated from nucleosides by purine nucleoside phosphorylase (although adenosine is not a substrate of this enzyme). Deamination of the base adenine occurs with AMP and adenosine deaminase. Of the purines, only adenosine can be phosphorylated directly back to a nucleotide, by adenosine kinase. ADP, adenosine diphosphate; APRT, adenine phosphoribosyltransferase; ATP, adenosine triphosphate; GMP, guanosine monophosphate; HGPRT, hypoxanthine–guanine phosphoribosyltransferase; IMP, inosine monophosphate; Pi, inorganic phosphate; PPi, pyrophosphate; PRPP, 5-phosphoribosyl 1-pyrophosphate.
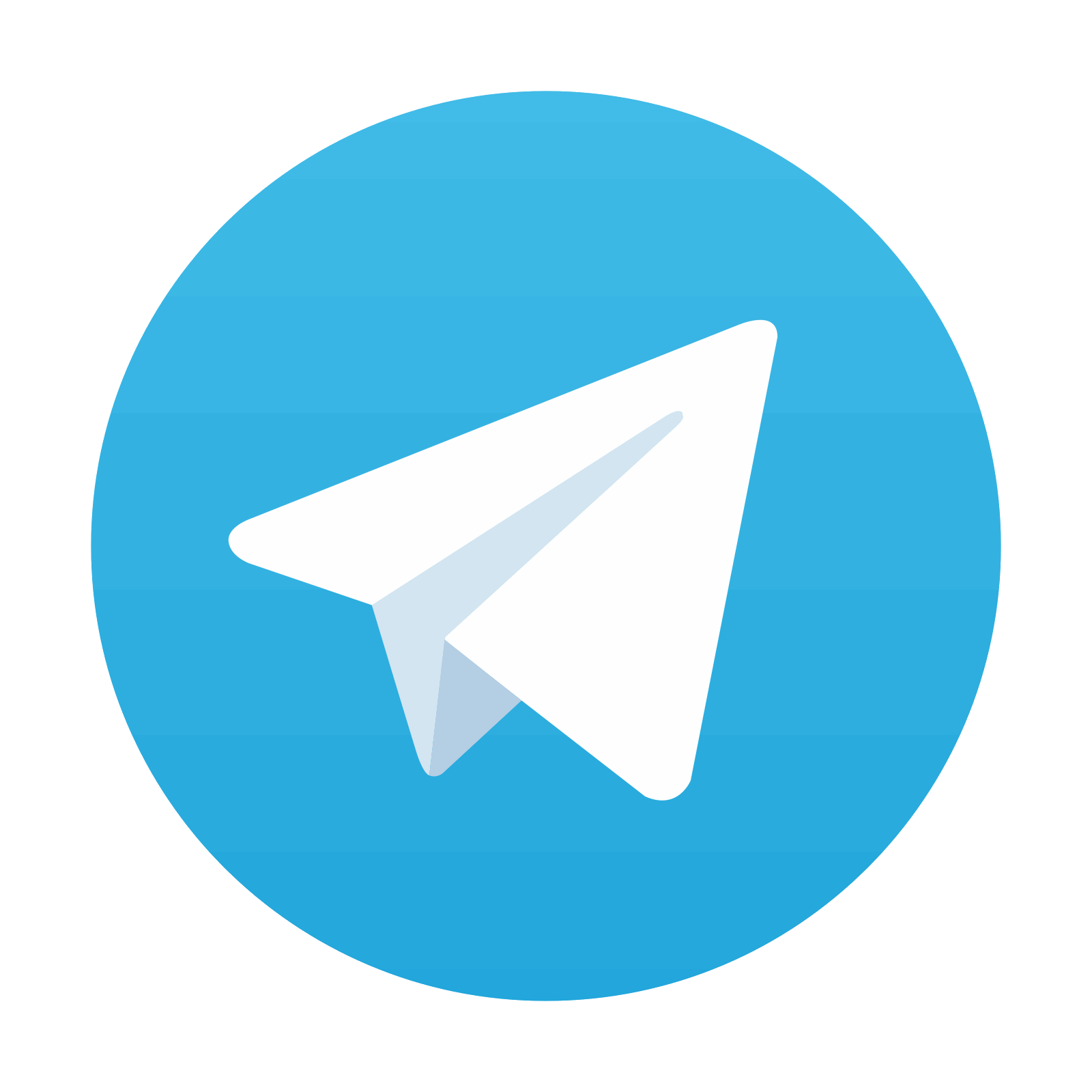
Stay updated, free articles. Join our Telegram channel

Full access? Get Clinical Tree
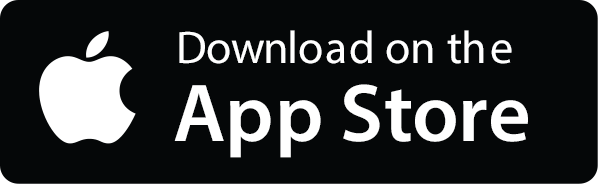
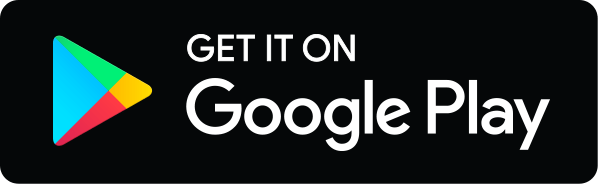