Pulmonary Pharmacology
This chapter discusses the pharmacotherapy of obstructive airways disease, particularly bronchodilators, which act mainly by reversing airway smooth muscle contraction, and anti-inflammatory drugs, which suppress the inflammatory response in the airways. The chapter focuses on the pulmonary pharmacology of β2 agonists and corticosteroids; their basic pharmacology is presented elsewhere (see Chapters 12 and 42). This chapter also discusses other drugs used to treat obstructive airway diseases, such as mucolytics and respiratory stimulants, and covers the drug therapy of cough, the most common respiratory symptom, as well as drugs used to treat pulmonary hypertension. Drugs used in the treatment of lung infections, including tuberculosis (see Chapter 56), are covered elsewhere.
MECHANISMS OF ASTHMA
Asthma is a chronic inflammatory disease of the airways that is characterized by activation of mast cells (generally present in increased numbers), infiltration of eosinophils, and T helper 2 (TH2)lymphocytes (Figure 36–1). Mast cell activation by allergens and physical stimuli releases bronchoconstrictor mediators, such as histamine, leukotriene D4, and PGD2, which cause bronchoconstriction, microvascular leakage, and plasma exudation (see Chapters 32 and 33). Many of the symptoms of asthma are due to airway smooth muscle contraction; thus, bronchodilators are important as symptom relievers. Whether airway smooth muscle is intrinsically abnormal in asthma is not clear, but increased contractility of airway smooth muscle may contribute to airway hyperresponsiveness, the physiological hallmark of asthma. The mechanism of chronic inflammation in asthma is still not well understood. It may initially be driven by allergen exposure, but it appears to become autonomous so that asthma is essentially incurable. The inflammation may be orchestrated by dendritic cells that regulate TH2 cells that drive eosinophilic inflammation and IgE formation by B lymphocytes. Airway epithelium plays an important role through the release of myriad inflammatory mediators and through the release of growth factors in an attempt to repair the damage caused by inflammation.
Figure 36–1 Cellular mechanisms of asthma. Myriad inflammatory cells are recruited and activated in the airways, where they release multiple inflammatory mediators, which can also arise from structural cells. These mediators lead to bronchoconstriction, plasma exudation and edema, vasodilation, mucus hypersecretion, and activation of sensory nerves. Chronic inflammation leads to structural changes, including subepithelial fibrosis (basement membrane thickening), airway smooth muscle hypertrophy and hyperplasia, angiogenesis, and hyperplasia of mucus-secreting cells.
Chronic inflammation may lead to structural changes in the airways, including an increase in the number and size of airway smooth muscle cells, blood vessels, and mucus-secreting cells. A characteristic histological feature of asthma is collagen deposition (fibrosis) below the basement membrane of the airway epithelium (see Figure 36–1).
MECHANISMS OF COPD
COPD involves inflammation of the respiratory tract with a pattern that differs from that of asthma. In COPD, there is a predominance of neutrophils, macrophages, and cytotoxic T-lymphocytes (Tcl cells). The inflammation predominantly affects small airways, resulting in progressive small airway narrowing and fibrosis (chronic obstructive bronchiolitis) and destruction of the lung parenchyma with destruction of the alveolar walls (emphysema) (Figure 36–2). These pathological changes result in airway closure on expiration, leading to air trapping and hyperinflation, particularly on exercise. This accounts for shortness of breath on exertion and exercise limitation that are characteristic symptoms of COPD.
Figure 36–2 Cellular mechanisms in chronic obstructive pulmonary disease. Cigarette smoke and other irritants activate epithelial cells and macrophages in the lung to release mediators that attract circulating inflammatory cells, including monocytes (which differentiate to macrophages within the lung), neutrophils, and T lymphocytes (TH1 and TC1 cells). Fibrogenic factors released from epithelial cells and macrophages lead to fibrosis of small airways. Release of proteases results in alveolar wall destruction (emphysema) and mucus hypersecretion (chronic bronchitis).
Bronchodilators reduce air trapping by dilating peripheral airways and are the mainstay of treatment in COPD. In contrast to asthma, the airflow obstruction of COPD tends to be progressive. A discrete pattern of inflammatory mediators and cytokines mediates the inflammation in the peripheral lung of COPD patients. In contrast to asthma, the inflammation of COPD is largely corticosteroid-resistant, and there are currently no effective anti-inflammatory treatments. Many patients with COPD have systemic manifestations (skeletal muscle wasting, weight loss, depression, osteoporosis, anemia) and comorbid diseases (ischemic heart disease, hypertension, congestive heart failure, diabetes). Whether these are due to spillover of inflammatory mediators from the lung or due to common causal mechanisms (such as smoking) is not yet clear, but it may be important to treat the systemic components in the overall management of COPD.
ROUTES OF DRUG DELIVERY TO THE LUNGS
INHALED ROUTE
Inhalation (Figure 36–3) is the preferred mode of delivery of many drugs with a direct effect on airways, particularly for asthma and COPD. The major advantage of inhalation is the delivery of drug to the airways in doses that are effective with a much lower risk of systemic side effects. This is particularly important with the use of inhaled corticosteroids (ICS), which largely avoids systemic side effects. In addition, inhaled bronchodilators have a more rapid onset of action than when taken orally.
Figure 36–3 Deposition of inhaled drugs (e.g., corticosteroids, β2 agonists). Inhalation therapy deposits drugs directly, but not exclusively, in the lungs. Distribution between lungs and oropharynx depends mostly on the particle size and the efficiency of the delivery method. Most material will be swallowed and absorbed, entering systemic circulation after undergoing the first-pass effect in the liver. Some drug will also be absorbed into the systemic circulation from the lungs. Use of a large-volume spacer will reduce the amount of drug deposited on oropharynx, thereby reducing amount swallowed and absorbed from GI tract, thus limiting systemic effects. MDI, metered-dose inhaler.
PARTICLE SIZE. The size of particles for inhalation is of critical importance in determining the site of deposition in the respiratory tract. The optimum size for particles to settle in the airways is 2-5 μm mass median aerodynamic diameter (MMAD). Larger particles settle out in the upper airways, whereas smaller particles remain suspended and are therefore exhaled. There is increasing interest in delivering drugs to small airways, particularly in COPD and severe asthma. This involves delivering drug particles of ~1 μm MMAD, which is now possible using drugs formulated in hydrofluoroalkane (HFA) propellant.
PHARMACOKINETICS. Of the total drug delivered, only 10-20% enters the lower airways with a conventional pressurized metered-dose inhaler. Drugs are absorbed from the airway lumen and have direct effects on target cells of the airway. Drugs may also be absorbed into the bronchial circulation and then distributed to more peripheral airways. Drugs with higher molecular weights tend to be retained to a greater extent in the airways. Nevertheless, several drugs have greater therapeutic efficacy when given by the inhaled route. More extensive pulmonary distribution of a drug with a smaller MMAD increases alveolar deposition and thus is likely to increase absorption from the lungs into the general circulation resulting in more systemic side effects.
DELIVERY DEVICES
Pressurized Metered-Dose Inhalers (pMDIs). Drugs are propelled from a canister with the aid of a propellant, previously with a chlorofluorocarbon (Freon) but now replaced by an HFA that is “ozone friendly.” These devices are convenient, portable, and typically deliver 100-400 doses of drug.
Spacer Chambers. Large-volume spacer devices between the pMDI and the patient reduce the velocity of particles entering the upper airways and the size of the particles by allowing evaporation of liquid propellant. This reduces the amount of drug that impinges on the oropharynx and gets swallowed, and increases the proportion of drug inhaled into the lower airways. Application of spacer chambers is useful in the reduction of the oropharyngeal deposition of ICS and the consequent reduction in the local and systemic side effects of these drugs. Spacer devices are also useful in delivering inhaled drugs to small children who are not able to use a pMDI.
Dry Powder Inhalers. Drugs may also be delivered as a dry powder using devices that scatter a fine powder dispersed by air turbulence on inhalation. Children <7 years of age find it difficult to use a dry powder inhaler (DPI). DPIs have been developed to deliver peptides and proteins, such as insulin (e.g., EXUBERA, AFRESA), systemically.
Nebulizers. Two types of nebulizer are available. Jet nebulizers are driven by a stream of gas (air or oxygen), whereas ultrasonic nebulizers use a rapidly vibrating piezo-electric crystal and thus do not require a source of compressed gas. The nebulized drug is inspired during tidal breathing, and it is possible to deliver much higher doses of drug compared with pMDI. Nebulizers are useful in treating acute exacerbations of asthma and COPD, for delivering inhaled drugs to infants and small children, and for giving drugs such as antibiotics when relatively high doses must be delivered.
ORAL ROUTE
Drugs for treatment of pulmonary diseases may also be given orally. The oral dose is much higher than the inhaled dose required to achieve the same effect (typically by a ratio of ~20:1), so that systemic side effects are more common. When there is a choice of inhaled or oral route for a drug (e.g.,β2 agonist or corticosteroid), the inhaled route is always preferable, and the oral route should be reserved for the few patients unable to use inhalers (e.g., small children, patients with physical problems such as severe arthritis of the hands). Theophylline is ineffective by the inhaled route; it must be given systemically. Corticosteroids may have to be given orally for parenchymal lung diseases (e.g., in interstitial lung diseases).
PARENTERAL ROUTE
The intravenous route should be reserved for delivery of drugs in the severely ill patient who is unable to absorb drugs from the GI tract. Side effects are generally frequent due to the high plasma concentrations.
BRONCHODILATORS
Bronchodilator drugs relax constricted airway smooth muscle in vitro and cause immediate reversal of airway obstruction in asthma in vivo. They also prevent bronchoconstriction (and thereby provide bronchoprotection). Three main classes of bronchodilator are in current clinical use:
• β2 Adrenergic agonists (sympathomimetics)
• Theophylline (a methylxanthine)
• Anticholinergic agents (muscarinic receptor antagonists)
Drugs such as cromolyn sodium, which prevent bronchoconstriction, have no direct bronchodilator action and are ineffective once bronchoconstriction has occurred. Anti-leukotrienes (leukotriene receptor antagonists and 5‼-lipoxygenase inhibitors) have a small bronchodilator effect in some asthmatic patients and appear to prevent bronchoconstriction. Corticosteroids, although gradually improving airway obstruction, have no direct effect on contraction of airway smooth muscle and are not therefore considered to be bronchodilators.
β2 ADRENERGIC AGONISTS
Inhaled β2 agonists are the bronchodilator treatment of choice in asthma because they are the most effective bronchodilators and have minimal side effects when used correctly. The basic pharmacology of these agents (albuterol, terbutaline, salmeterol, formoterol, indacaterol, and related compounds) is presented in Chapters 8 and 12.
MODE OF ACTION
Agonist stimulation of β2 receptors in airway smooth muscle results in the activation of the Gs-adenylyl cyclase-cAMP-PKA pathway and consequent phosphorylative events leading to bronchial smooth muscle relaxation (Figure 36–4), effectively reversing the Ca2+-stimulated events that initiate contraction.
Figure 36–4 Molecular actions of β2 agonists to induce relaxation of airway smooth muscle cells. A rise in [Ca2+]i initiates contraction by activating myosin light-chain kinase (MLCK), thereby enhancing the level of phosphorylation of myosin light chains and increasing the contractile interaction of actin and myosin. Stimulation of β2 receptors activates cyclic AMP-PKA pathway and reverses the contractile process by reducing [Ca2+]i, reducing MLCK activation, and promoting dephosphorylation of light chains. PKA phosphorylates a variety of target substrates, resulting in: opening of Ca2+-activated K+ channels (KCa) [which facilitates hyperpolarization], decreased phosphoinositide (PI) hydrolysis, increased Na+/Ca2+ exchange, increased Na+, Ca2+-ATPase activity, decreased MLCK activity, and increased MLC phosphatase activity. β2 Receptors may also couple to KCa via Gs. PDE, cyclic nucleotide phosphodiesterase.
β2 agonists may cause bronchodilation in vivo not only via a direct action on airways smooth muscle, but also indirectly by inhibiting the release of bronchoconstrictor mediators from inflammatory cells and of bronchoconstrictor neurotransmitters from airway nerves. These mechanisms include:
• Prevention of mediator release from isolated human lung mast cells (via β2 receptors)
• Prevention of microvascular leakage and thus the development of bronchial mucosal edema after exposure to mediators, such as histamine and leukotriene D4
• Increase in mucus secretion from submucosal glands and ion transport across airway epithelium (may enhance mucociliary clearance, reversing defective clearance found in asthma)
• Reduction in neurotransmission in human airway cholinergic nerves by an action at presynaptic β2 receptors to inhibit acetylcholine release
β2 agonists do not appear to have a significant inhibitory effect on the chronic inflammation of asthmatic airways, which is suppressed by corticosteroids. This may be related to the fact that effects of β2 agonists on macrophages, eosinophils, and lymphocytes are rapidly desensitized.
CLINICAL USE
SHORT-ACTING β2 AGONISTS. Inhaled short-acting β2 agonists are the most widely used and effective bronchodilators in the treatment of asthma due to their functional antagonism of bronchoconstriction. These agents also are effective in protecting against various challenges, such as exercise, cold air, and allergens. Inhalation is preferable to the oral administration because inhalation may be more effective and systemic side effects are less. Short-acting inhaled β2 agonists, such as albuterol, should be used “as required” by symptoms and not on a regular basis in the treatment of mild asthma; increased use indicates the need for more anti– inflammatory therapy.
Slow-release preparations (e.g., slow-release albuterol and bambuterol) may be indicated in nocturnal asthma; however, these agents have an increased risk of side effects. All short-acting β2 agonists currently available are usable by inhalation and orally, have a similar duration of action (~3-4 h; less in severe asthma), and similar side effects. Drugs in clinical use include albuterol (salbutamol), levalbuterol metaproterenol, terbutaline (in the U.S.), fenoterol, tulobuterol, rimiterol, and pirbuterol (in other countries).
LONG-ACTING INHALED β2 AGONISTS. The long-acting inhaled β2 agonists (LABA) salmeterol, formoterol, and arformoterol have a bronchodilator action of >12 h and protect against bronchoconstriction for a similar period. They improve asthma control (when given twice daily) compared with regular treatment with short-acting β2 agonists (4-6 times daily). Indacaterol is a once-daily “ultra-LABA” approved for treatment of COPD but not asthma; its duration of action exceeds those of salmeterol and formoterol.
In COPD, LABA are effective bronchodilators that may be used alone or in combination with anticholinergics or ICS. LABA improve symptoms and exercise tolerance by reducing both air trapping and exacerbations. In asthma patients, LABA should never be used alone because they do not treat the underlying chronic inflammation; rather, LABA should always be used in combination with ICS (preferably in a fixed-dose combination inhaler). LABA are an effective add-on therapy to ICS and are more effective than increasing the dose of ICS when asthma is not controlled at low doses. Tolerance to the bronchodilator effect of formoterol and the bronchoprotective effects of formoterol and salmeterol has been demonstrated but is of doubtful clinical significance. No significant clinical differences between salmeterol and formoterol have been found in the treatment of patients with severe asthma.
COMBINATION INHALERS. Combination inhalers that contain a LABA and a corticosteroid (e.g., fluticasone/salmeterol [ADVAIR], budesonide/formoterol [SYMBICORT]) are now widely used in the treatment of asthma and COPD. Combining LABA with a corticosteroid offers complementary synergistic actions. The combination inhaler is more convenient for patients, simplifies therapy, and improves compliance. These combination inhalers are also more effective in COPD patients than LABA and ICS alone.
SIDE EFFECTS. Unwanted effects are dose related and due to stimulation of extrapulmonary β receptors (Table 36–1 and Chapter 12). Side effects are not common with inhaled therapy but quite common with oral or intravenous administration, usually only after large systemic doses. Short-acting inhaled β2 agonists should only be used on demand for symptom control, and with an ICS if they are required more than 3 times weekly. LABA should only be used when ICS are also prescribed. All LABA approved in the U.S. carry a black box warning cautioning against overuse. There are less safety concerns with LABA use in COPD.
Table 36-1
Side Effects of β Agonists
METHYLXANTHINES
The methylxanthine, theophylline, is still widely used in developing countries because it is inexpensive. However, the frequency of side effects and the relative low efficacy of theophylline have led to reduced use because inhaled β2 agonists are far more effective as bronchodilators and ICS have a greater anti-inflammatory effect. In patients with severe asthma and COPD, theophylline remains a very useful drug.
MECHANISM OF ACTION. The mechanisms of action of theophylline are still uncertain. In addition to its bronchodilator action, theophylline has many nonbronchodilator effects that may be relevant to its effects in asthma and COPD (Figure 36–5). Several molecular mechanisms of action have been proposed:
Figure 36–5 Theophylline affects multiple cell types in the airway.
• Inhibition of cyclic nucleotide phosphodiesterases. Theophylline is a nonselective PDE inhibitor. PDE inhibition and the concomitant elevation of cellular cyclic AMP and cyclic GMP likely account for the bronchodilator action of theophylline (see Figure 36–4). The PDE isoenzymes recognized in smooth muscle relaxation include PDE3, PDE4, and PDE5.
• Adenosine receptor antagonism. Theophylline antagonizes adenosine receptors at therapeutic concentrations. Adenosine causes bronchoconstriction in airways from asthmatic patients by releasing histamine and leukotrienes. Antagonism of A1 receptors may be responsible for serious side effects, including cardiac arrhythmias and seizures.
• Interleukin-10 release
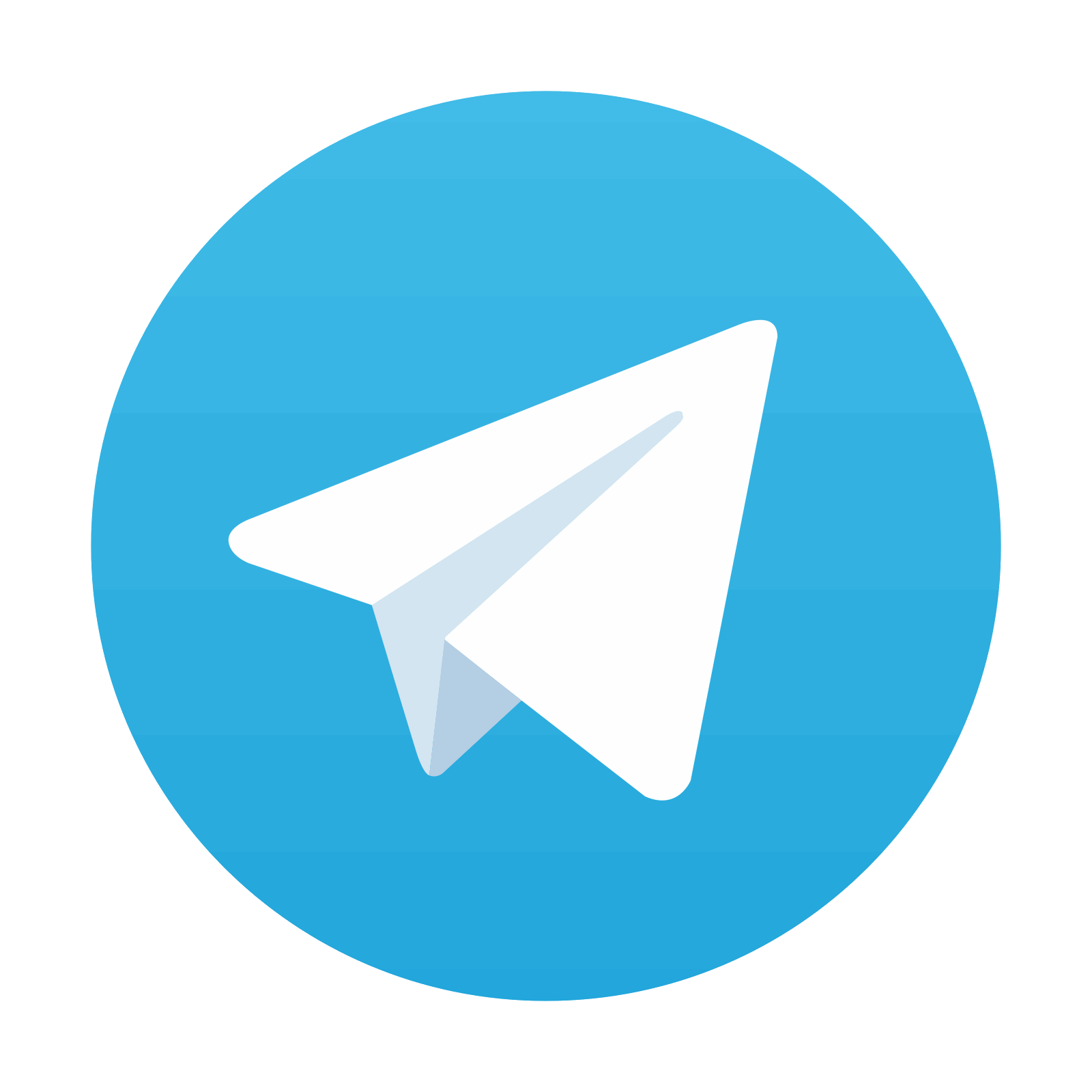
Stay updated, free articles. Join our Telegram channel

Full access? Get Clinical Tree
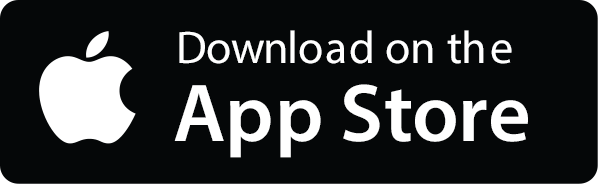
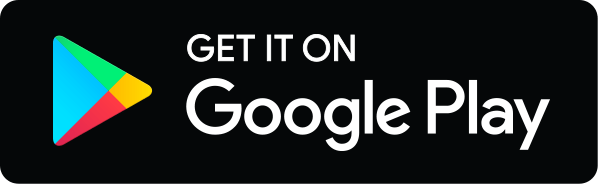