Hematopoietic Agents: Growth Factors, Minerals, and Vitamins
The finite life span of most mature blood cells requires their continuous replacement, a process termed hematopoiesis. New cell production must respond to basal needs and states of increased demand. Red blood cell production can increase >20-fold in response to anemia or hypoxemia, white blood cell production increases dramatically in response to a systemic infection, and platelet production can increase 10- to 20-fold when platelet consumption results in thrombocytopenia.
The regulation of blood cell production is complex. Hematopoietic stem cells are rare bone marrow cells that manifest self-renewal and lineage commitment, resulting in cells destined to differentiate into the 9 distinct blood-cell lineages. For the most part, this process occurs in the marrow cavities of the skull, vertebral bodies, pelvis, and proximal long bones; it involves interactions among hematopoietic stem and progenitor cells and the cells and complex macromolecules of the marrow stroma, and is influenced by a number of soluble and membrane-bound hematopoietic growth factors. Some of these hormones and cytokines have been identified and cloned, permitting their production in quantities sufficient for therapeutic use. Clinical applications range from the treatment of primary hematologic diseases to use as adjuncts in the treatment of severe infections and in the management of patients who are undergoing cancer chemotherapy or marrow transplantation.
Hematopoiesis also requires an adequate supply of minerals (e.g., iron, cobalt, and copper) and vitamins (e.g., folic acid, vitamin B12, pyridoxine, ascorbic acid, and riboflavin); deficiencies generally result in characteristic anemias or, less frequently, a general failure of hematopoiesis. Therapeutic correction of a specific deficiency state depends on the accurate diagnosis of the anemic state, and on knowledge about the correct dose, the use of these agents in appropriate combinations, and the expected response.
Hematopoietic Growth Factors
GROWTH FACTOR PHYSIOLOGY. Steady-state hematopoiesis encompasses the production of >400 billion blood cells each day. This production is tightly regulated and can be increased several-fold with increased demand. The hematopoietic organ also is unique in adult physiology in that several mature cell types are derived from a much smaller number of multipotent progenitors, which develop from a more limited number of pluripotent hematopoietic stem cells. Such cells are capable of maintaining their own number and differentiating under the influence of cellular and humoral factors to produce the large and diverse number of mature blood cells.
Stem cell differentiation can be described as a series of steps that produce so-called burst-forming units (BFUs) and colony-forming units (CFUs) for each of the major cell lines. These early progenitors (BFU and CFU) are capable of further proliferation and differentiation, increasing their number by some 30-fold. Subsequently, colonies of morphologically distinct cells form under the control of an overlapping set of additional growth factors (granulocyte colony-stimulating factor [G-CSF], macrophage colony-stimulating factor [M-CSF], erythropoietin, and thrombopoietin). Proliferation and maturation of the CFU for each cell line can amplify the resulting mature cell product by another 30-fold or more, generating >1000 mature cells from each committed stem cell.
Hematopoietic and lymphopoietic growth factors are glycoproteins produced by a number of marrow cells and peripheral tissues. They are active at very low concentrations and typically affect more than 1 committed cell lineage. Most interact synergistically with other factors and also stimulate production of additional growth factors, a process called networking. Growth factors generally exert actions at several points in the processes of cell proliferation and differentiation and in mature cell function. However, the network of growth factors that contributes to any given cell lineage depends absolutely on a nonredundant, lineage-specific factor, such that absence of factors that stimulate developmentally early progenitors is compensated for by redundant cytokines, but loss of the lineage-specific factor leads to a specific cytopenia.
Some of the overlapping and nonredundant effects of the more important hematopoietic growth factors are illustrated in Figure 37–1 and Table 37–1.
Figure 37–1 Sites of action of hematopoietic growth factors in the differentiation and maturation of marrow cell lines. A self-sustaining pool of marrow stem cells differentiates under the influence of specific hematopoietic growth factors to form a variety of hematopoietic and lymphopoietic cells. Stem cell factor (SCF), ligand (FL), interleukin-3 (IL-3), and granulocyte-macrophage colony-stimulating factor (GM-CSF), together with cell–cell interactions in the marrow, stimulate stem cells to form a series of burst-forming units (BFU) and colony-forming units (CFU): CFU-GEMM (granulocyte, erythrocyte, monocyte and megakaryocyte), CFU-GM (granulocyte and macrophage), CFU-Meg (megakaryocyte), BFU-E (erythrocyte), and CFU-E (erythrocyte). After considerable proliferation, further differentiation is stimulated by synergistic interactions with growth factors for each of the major cell lines—granulocyte colony-stimulating factor (G-CSF), monocyte/macrophage-stimulating factor (M-CSF), thrombo-poietin, and erythropoietin. Each of these factors also influences the proliferation, maturation, and in some cases the function of the derivative cell line (Table 37–1).
Table 37–1
Hematopoietic Growth Factors
ERYTHROPOIESIS-STIMULATING AGENTS
Erythropoiesis-stimulating agent (ESA) is the term given to a pharmacological substance that stimulates red blood cell production.
Erythropoietin is the most important regulator of the proliferation of committed erythroid progenitors (CFU-E) and their immediate progeny. In its absence, severe anemia is invariably present, commonly seen in patients with renal failure. Erythropoiesis is controlled by a feedback system in which a sensor in the kidney detects changes in oxygen delivery to modulate the erythropoietin secretion. The sensor mechanism is now understood at the molecular level.
Hypoxia-inducible factor (HIF-1), a heterodimeric (HIF-1α and HIF-1β) transcription factor, enhances expression of multiple hypoxia-inducible genes, such as vascular endothelial growth factor and erythropoietin. HIF-1α is labile due to its prolyl hydroxylation and subsequent polyubiquitination and degradation, aided by the von Hippel-Lindau (VHL)protein. During states of hypoxia, the prolyl hydroxylase is inactive, allowing the accumulation of HIF-1α and activating erythropoietin expression, which in turn stimulates a rapid expansion of erythroid progenitors. Specific alteration of VHL leads to an oxygen-sensing defect, characterized by constitutively elevated levels of HIF-1α and erythropoietin, with a resultant polycythemia.
Erythropoietin is expressed primarily in peritubular interstitial cells of the kidney. Erythropoietin contains 193 amino acids, of which the first 27 are cleaved during secretion. The final hormone is heavily glycosylated and has a molecular mass of ~30 kDa. After secretion, erythropoietin binds to a receptor on the surface of committed erythroid progenitors in the marrow and is internalized. With anemia or hypoxemia, synthesis rapidly increases by 100-fold or more, serum erythropoietin levels rise, and marrow progenitor cell survival, proliferation, and maturation are dramatically stimulated. This finely tuned feedback loop can be disrupted by kidney disease, marrow damage, or a deficiency in iron or an essential vitamin. With an infection or an inflammatory state, erythropoietin secretion, iron delivery, and progenitor proliferation all are suppressed by inflammatory cytokines, but this accounts for only part of the resultant anemia; interference with iron metabolism also is an effect of inflammatory mediator effects on the hepatic protein hepcidin.
PREPARATIONS. Available preparations of recombinant human erythropoietin (epoetin alfa) include EPOGEN, PROCRIT, and EPREX, supplied in single-use vials containing 2000-40,000 units/mL for intravenous or subcutaneous administration. When injected intravenously, epoetin alfa is cleared from plasma with a t1/2 of 4-8 h. However, the effect on marrow progenitors lasts much longer, and once-weekly dosing can be sufficient to achieve an adequate response. A novel erythropoiesis-stimulating protein, darbepoetin alfa (ARANESP), has been approved for clinical use in patients with indications similar to those for epoetin alfa. It is a genetically modified form of erythropoietin in which 4 amino acids have been mutated such that additional carbohydrate side chains are added during its synthesis, prolonging the circulatory survival of the drug to 24-26 h. Another erythropoiesis-stimulating peptide, Peginesatide (OMONTYS), was approved in 2012 for the treatment of anemia due to chronic kidney disease. Postmarketing reports of serious hypersensitivity reactions and anaphylaxis have necessitated a recall.
Recombinant human erythropoietin (epoetin alfa) is nearly identical to the endogenous hormone. The carbohydrate modification pattern of epoetin alfa differs slightly from the native protein, but this difference apparently does not alter kinetics, potency, or immunoreactivity of the drug. Modern assays can detect these differences and thereby identify athletes who use the recombinant product for “blood doping.”
THERAPEUTIC USES, MONITORING, AND ADVERSE EFFECTS. Recombinant erythropoietin therapy, in conjunction with adequate iron intake, can be highly effective in a number of anemias, especially those associated with a poor erythropoietic response. Epoetin alfa is effective in the treatment of anemias associated with surgery, AIDS, cancer chemotherapy, prematurity, and certain chronic inflammatory conditions. Darbepoetin alfa also has been approved for use in patients with anemia associated with chronic kidney disease.
During erythropoietin therapy, absolute or functional iron deficiency may develop. Functional iron deficiency (i.e., normal ferritin levels but low transferrin saturation) presumably results from the inability to mobilize iron stores rapidly enough to support the increased erythropoiesis. Supplemental iron therapy is recommended for all patients whose serum ferritin is <100 μg/L or whose serum transferrin saturation is <20%. During initial therapy and after any dosage adjustment, the hematocrit is determined once a week (HIV-infected and cancer patients) or twice a week (renal failure patients) until it has stabilized in the target range and the maintenance dose has been established; the hematocrit then is monitored at regular intervals. If the hematocrit increases by >4 points in any 2-week period, the dose should be decreased. Due to the time required for erythropoiesis and the erythrocyte half-life, hematocrit changes lag behind dosage adjustments by 2-6 weeks. The dose of darbepoetin should be decreased if the hemoglobin increase exceeds 1 g/dL in any 2-week period because of the association of excessive rate of rise of hemoglobin with adverse cardiovascular events.
During hemodialysis, patients receiving epoetin alfa or darbepoetin may require increased anticoagulation. The risk of thrombotic events is higher in adults with ischemic heart disease or congestive heart failure receiving epoetin alfa therapy with the goal of reaching a normal hematocrit (42%) than in those with a lower target hematocrit of 30%. ESA use is associated with increased rates of cancer recurrence and decreased on-study survival in patients in whom the drugs are administered for cancer-induced or for chemotherapy-induced anemia. The most common side effect of epoetin alfa therapy is aggravation of hypertension, which occurs in 20-30% of patients and most often is associated with a rapid rise in hematocrit. ESAs should not be used in patients with preexisting uncontrolled hypertension. Patients may require initiation of, or increases in, antihypertensive therapy. Hypertensive encephalopathy and seizures have occurred in chronic renal failure patients treated with epoetin alfa. Headache, tachycardia, edema, shortness of breath, nausea, vomiting, diarrhea, injection site stinging, and flu-like symptoms (e.g., arthralgias and myalgias) also have been reported in conjunction with epoetin alfa therapy.
Anemia of Chronic Renal Failure. Patients with anemia secondary to chronic kidney disease are ideal candidates for epoetin alfa therapy. The response in predialysis, peritoneal dialysis, and hemodialysis patients depends on the severity of the renal failure, the erythropoietin dose and route of administration, and iron availability. The subcutaneous route of administration is preferred over the intravenous route because absorption is slower and the amount of drug required is reduced by 20-40%. The dose of epoetin alfa should be adjusted to obtain a gradual rise in the hematocrit over a 2- to 4-month period to a final hematocrit of 33-36%. Treatment to hematocrit levels >36% is not recommended.
Patients are started on doses of 80-120 units/kg of epoetin alfa, given subcutaneously, 3 times a week. The final maintenance dose of epoetin alfa can vary from 10 units/kg to >300 units/kg, with an average dose of 75 units/kg, 3 times a week. Children <5 years of age generally require a higher dose. Resistance to therapy is common in patients who develop an inflammatory illness or become iron deficient, so close monitoring of general health and iron status is essential. Less common causes of resistance include occult blood loss, folic acid deficiency, carnitine deficiency, inadequate dialysis, aluminum toxicity, and osteitis fibrosa cystica secondary to hyperparathyroidism. Darbepoetin alfa is approved for use in patients who are anemic secondary to chronic kidney disease. The recommended starting dose is 0.45 µg/kg administered intravenously or subcutaneously once weekly, with dose adjustments depending on the response. Like epoetin alfa, side effects tend to occur when patients experience a rapid rise in hemoglobin concentration; a rise of <1 g/dL every 2 weeks generally is considered safe.
Anemia in AIDS Patients. Epoetin alfa therapy has been approved for the treatment of HIV-infected patients, especially those on zidovudine therapy. Excellent responses to doses of 100-300 units/kg, given subcutaneously 3 times a week, generally are seen in patients with zidovudine-induced anemia.
Cancer-Related Anemias. Epoetin alfa therapy, 150 units/kg 3 times a week or 450-600 units/kg once a week, can reduce the transfusion requirement in cancer patients undergoing chemotherapy. Therapeutic guidelines recommend the use of epoetin alfa in patients with chemotherapy-associated anemia when hemoglobin levels fall below 10 g/dL, basing the decision to treat less severe anemia (Hb, 10-12 g/dL) on clinical circumstances. For anemia associated with hematologic malignancies, the guidelines support the use of recombinant erythropoietin in patients with low-grade myelodysplastic syndrome. A baseline serum erythropoietin level may help to predict the response; most patients with blood levels >500 IU/L are unlikely to respond to any dose of the drug. Most patients treated with epoetin alfa experience an improvement in their anemia and their sense of well-being.
Recent case reports suggest a direct effect of both epoetin alfa and darbepoetin alfa in stimulation of tumor cells. A meta-analysis of a large number of patients and clinical trials estimates the risk at ~10% higher than nontreated cancer patients. This finding is being evaluated by the FDA and warrants serious attention.
Surgery and Autologous Blood Donation. Epoetin alfa has been used perioperatively to treat anemia (hematocrit 30-36%) and reduce the need for erythrocyte transfusion. Patients undergoing elective orthopedic and cardiac procedures have been treated with 150-300 units/kg of epoetin alfa once daily for the 10 days preceding surgery, on the day of surgery, and for 4 days after surgery. As an alternative, 600 units/kg can be given on days 21, 14, and 7 before surgery, with an additional dose on the day of surgery. Epoetin alfa also has been used to improve autologous blood donation.
Other Uses. Epoetin alfa has received orphan drug status from the FDA for the treatment of the anemia of prematurity, HIV infection, and myelodysplasia. In the latter case, even very high doses >1000 units/kg 2 to 3 times a week have had limited success. Highly competitive athletes have used epoetin alfa to increase their hemoglobin levels (“blood doping”) and improve performance. Unfortunately, this misuse of the drug has been implicated in the deaths of several athletes and is strongly discouraged.
MYELOID GROWTH FACTORS
The myeloid growth factors are glycoproteins that stimulate the proliferation and differentiation of one or more myeloid cell lines. Recombinant forms of several growth factors have been produced, including granulocyte-macrophage colony-stimulating factor (GM-CSF), G-CSF, IL-3, M-CSF or CSF-1, and stem cell factor (SCF) (see Table 37–1).
Myeloid growth factors are produced naturally by a number of different cells, including fibroblasts, endothelial cells, macrophages, and T cells (Figure 37–2). These factors are active at extremely low concentrations and act via membrane receptors of the cytokine receptor superfamily to activate the JAK/STAT signal transduction pathway. GM-CSF can stimulate proliferation, differentiation, and function of a number of the myeloid cell lineages (see Figure 37–1). It acts synergistically with other growth factors, including erythropoietin, at the level of the BFU. GM-CSF stimulates CFU-granulocyte(G)/erythrocyte(E)/monocyte(M)/megakaryocyte(Meg) [CFU-GEMM], CFU-GM, CFU-M, CFU-E, and CFU-Meg to increase cell production. GM-CSF also enhances the migration, phagocytosis, superoxide production, and antibody-dependent cell-mediated toxicity of neutrophils, monocytes, and eosinophils.
Figure 37–2 Cytokine-cell interactions. Macrophages, T cells, B cells, and marrow stem cells interact via several cytokines (IL-1, IL-2, IL-3, IL-4, IFN [interferon]-γ, GM-CSF, and G-CSF) in response to a bacterial or a foreign antigen challenge. See Table 37–1 for the functional activities of these various cytokines.
The G-CSF activity is restricted to neutrophils and their progenitors, stimulating their proliferation, differentiation, and function. It acts primarily on the CFU-G, although it also can play a synergistic role with IL-3 and GM-CSF in stimulating other cell lines. G-CSF enhances phagocytic and cytotoxic activities of neutrophils. G-CSF reduces inflammation by inhibiting IL-1, tumor necrosis factor, and interferon gamma. G-CSF also mobilizes primitive hematopoietic cells, including hematopoietic stem cells, from the marrow into the peripheral blood. This observation has virtually transformed the practice of stem cell transplantation, such that >90% of all such procedures today use G-CSF–mobilized peripheral blood stem cells as the donor product.
GRANULOCYTE-MACROPHAGE COLONY-STIMULATING FACTOR. Recombinant human GM-CSF (sargramostim) is a 127–amino acid glycoprotein. The primary therapeutic effect of sargramostim is to stimulate myelopoiesis.
The initial clinical application of sargramostim was in patients undergoing autologous bone marrow transplantation. By shortening the duration of neutropenia, transplant morbidity was significantly reduced without a change in long-term survival or risk of inducing an early relapse of the malignant process. The role of GM-CSF therapy in allogeneic transplantation is less clear. Its effect on neutrophil recovery is less pronounced in patients receiving prophylactic treatment for graft-versus-host disease (GVHD). However, it may improve survival in transplant patients who exhibit early graft failure. It also has been used to mobilize CD34-positive progenitor cells for peripheral blood stem cell collection for transplantation after myeloablative chemotherapy. Sargramostim has been used to shorten the period of neutropenia and reduce morbidity in patients receiving intensive cancer chemotherapy. It also stimulates myelopoiesis in some patients with cyclic neutropenia, myelodysplasia, aplastic anemia, or AIDS-associated neutropenia.
Sargramostim (LEUKINE) is administered by subcutaneous injection or slow intravenous infusion at doses of 125-500 µg/m2/day. Plasma levels of GM-CSF rise rapidly after subcutaneous injection and then decline with a t1/2 of 2-3 h. When given intravenously, infusions should be maintained over 3-6 h. With the initiation of therapy, there is a transient decrease in the absolute leukocyte count secondary to margination and sequestration in the lungs. This is followed by a dose-dependent, biphasic increase in leukocyte counts over the next 7-10 days. Once the drug is discontinued, the leukocyte count returns to baseline within 2-10 days. When GM-CSF is given in lower doses, the response is primarily neutrophilic, whereas monocytosis and eosinophilia are observed at larger doses. After hematopoietic stem cell transplantation or intensive chemotherapy, sargramostim is given daily during the period of maximum neutropenia until a sustained rise in the granulocyte count is observed. Frequent blood counts are essential to avoid an excessive rise in the granulocyte count. Higher doses are associated with more pronounced side effects, including bone pain, malaise, flu-like symptoms, fever, diarrhea, dyspnea, and rash. An acute reaction to the first dose, characterized by flushing, hypotension, nausea, vomiting, and dyspnea, with a fall in arterial oxygen saturation due to granulocyte sequestration in the pulmonary circulation occurs in sensitive patients. With prolonged administration, a few patients may develop a capillary leak syndrome, with peripheral edema and pleural and pericardial effusions. Other serious side effects include transient supraventricular arrhythmia, dyspnea, and elevation of serum creatinine, bilirubin, and hepatic enzymes.
GRANULOCYTE COLONY-STIMULATING FACTOR. Recombinant human G-CSF, filgrastim (NEUPOGEN), is a 175–amino acid glycoprotein. The principal action of filgrastim is the stimulation of CFU-G to increase neutrophil production (see Figure 37–1).
Filgrastim is effective in the treatment of severe neutropenia after autologous hematopoietic stem cell transplantation and high-dose cancer chemotherapy. Like GM-CSF, filgrastim shortens the period of severe neutropenia and reduces morbidity secondary to bacterial and fungal infections. G-CSF also is effective in the treatment of severe congenital neutropenias. Filgrastim therapy can improve neutrophil counts in some patients with myelodysplasia or marrow damage (moderately severe aplastic anemia or tumor infiltration of the marrow). The neutropenia of AIDS patients receiving zidovudine also can be partially or completely reversed. Filgrastim is routinely used in patients undergoing peripheral blood stem cell (PBSC) collection for stem cell transplantation. It promotes the release of CD34+ progenitor cells from the marrow, reducing the number of collections necessary for transplant. G-CSF–induced mobilization of stem cells into the circulation has been promoted as a way to enhance repair of other damaged organs in which PBSC might play a role.
Filgrastim is administered by subcutaneous injection or intravenous infusion over at least 30 min at doses of 1-20 ′g/kg/day. The usual starting dose in a patient receiving myelosuppressive chemotherapy is 5 µg/kg/day. The distribution and clearance rate from plasma (t1/2 of 3.5 h) are similar for both routes of administration. As with GM-CSF therapy, filgrastim given daily after hematopoietic stem cell transplantation or intensive cancer chemotherapy will increase granulocyte production and shorten the period of severe neutropenia. Frequent blood counts should be obtained to determine the effectiveness of the treatment and guide dosage adjustment. In patients who received intensive myelosuppressive cancer chemotherapy, daily administration of G-CSF for ≥14-21 days may be necessary to correct the neutropenia. Adverse reactions to filgrastim include mild to moderate bone pain in patients receiving high doses over a protracted period, local skin reactions following subcutaneous injection, and rare cutaneous necrotizing vasculitis. Patients with a history of hypersensitivity to proteins produced by Escherichia coli should not receive the drug. Mild to moderate splenomegaly has been observed in patients on long-term therapy.
Pegylated recombinant human G-CSF pegfilgrastim (NEULASTA) is available. The clearance of pegfilgrastim by glomerular filtration is minimized, thus making neutrophil-mediated clearance the primary route of elimination. Consequently the circulating t1/2 of pegfilgrastim is longer than that of filgrastim, allowing for more sustained duration of action and less frequent dosing. The recommended dose for pegfilgrastim is fixed at 6 mg administered subcutaneously.
THROMBOPOIETIC GROWTH FACTORS
INTERLEUKIN-11. Interleukin-11 is a cytokine that stimulates hematopoiesis, intestinal epithelial cell growth, and osteoclasto-genesis and inhibits adipogenesis. Interleukin-11 also enhances megakaryocyte maturation in vitro. Recombinant human IL-11 oprelvekin (NEUMEGA) leads to a thrombopoietic response in 5-9 days when administered daily to normal subjects.
The drug is administered to patients at 25-50 μg/kg per day subcutaneously with a t1/2 ~7 h. Oprelvekin is approved for use in patients undergoing chemotherapy for nonmyeloid malignancies with severe thrombocytopenia (platelet count <20,000/μL), and it is administered until the platelet count returns to >100,000/μL. The major complications of therapy are fluid retention and associated cardiac symptoms, such as tachycardia, palpitation, edema, and shortness of breath; this is a significant concern in elderly patients and often requires concomitant therapy with diuretics. Also reported are blurred vision, injection-site rash or erythema, and paresthesias.
THROMBOPOIETIN. Thrombopoietin, a glycoprotein produced by the liver, marrow stromal cells, and other organs, is the primary regulator of platelet production. Two forms of recombinant thrombopoietin have been developed for clinical use. One is a truncated version of the native protein, termed recombinant human megakaryocyte growth and development factor
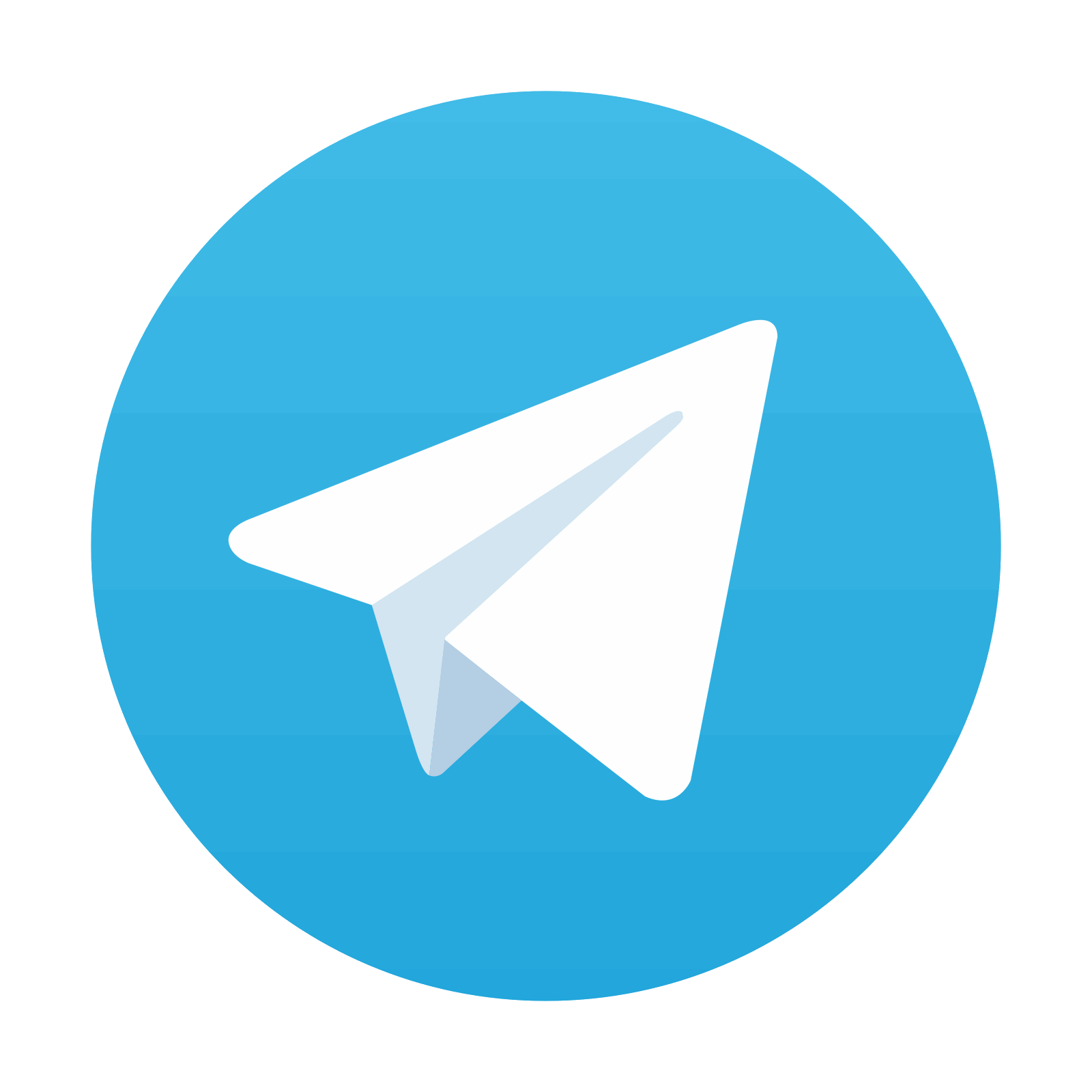
Stay updated, free articles. Join our Telegram channel

Full access? Get Clinical Tree
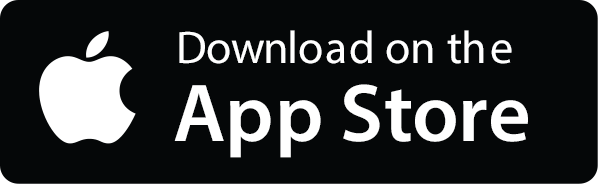
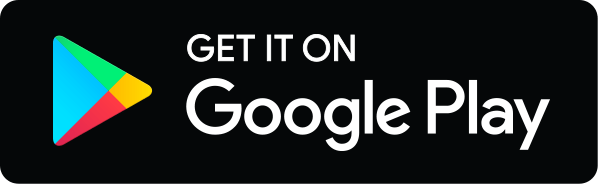