1 Introduction
Microorganisms are ubiquitous, and most of them are free-living and derive their nutrition from inert organic and inorganic materials. The association of humans with such microorganisms is generally harmonious, as the majority of those encountered are benign and, indeed, are often vital to commerce, health and a balanced ecosystem. The ability of bacteria and fungi to establish infections of plants, animals and humans varies considerably. Some are rarely, if ever, isolated from infected tissues, while opportunist pathogens (e.g. Pseudomonas aeruginosa or Staphylococcus epidermidis) can establish themselves only in compromised individuals. Only a few species of bac-teria may be regarded as obligate pathogens, for which animals or plants are the only reservoirs for their exist-ence (e.g. Neisseria gonorrhoeae, Mycobacterium tubercu-losis and Treponema pallidum). Viruses (Chapter 5), on the other hand, must parasitize host cells in order to replicate and are therefore inevitably associated with disease. Even among the viruses and obligate bacterial pathogens the degree of virulence varies, in that some (particularly the bacteria) are able to coexist with the host without causing overt disease (e.g. Staph. aureus), while others will always cause some detriment to the host (e.g. rabies virus). Organisms such as these invariably produce their effects, directly or indirectly, by actively growing on or in the host tissues.
Other groups of microorganisms may cause disease through ingestion of substances (toxins) produced during microbial growth on foods (e.g. Clostridium botulinum, botulism; Bacillus cereus, vomiting). In this case, the organisms themselves do not have to proliferate in the host for the effects of the toxin to be manifested.
Animals and plants constantly interact with bacteria present within their environment. For an infection to develop, such microorganisms must remain associated with host tissues and increase their numbers more rapidly than they can be either eliminated or killed. This balance relates to the ability of the bacterium to mobilize nutrients and multiply in the face of innate defences and a developing immune response by the now compromised host.
The greater the number of bacterial cells associated with the initial challenge to the host, the greater will be the chance of disease. If the pathogen does not arrive at its ‘p ortal of entry’ to the body or directly at its target tissues in sufficient number, then an infection will not ensue. The minimum number of viable microorganisms that is required to cause infection and thereby disease is called the minimum infective number (MIN). The MIN varies markedly between the various pathogens and is also affected by the general health and immune status of the individual host. The course of an infection can be considered as a sequence of separate events that includes initial contact with the pathogen, its consolidation and spread between and within organs and its eventual elimi-nation (Figure 7.1). Growth and consolidation of the microorganisms at the portal of entry commonly involves the formation of a microcolony (biofilm, see Chapters 3 and 8). Biofilms and microcolonies are collections of microorganisms that are attached to surfaces and enveloped within exopolymers (biofilm matrix) composed of polysaccharides, glycoproteins and/or proteins. Growth within the matrix not only protects the pathogens against opsonization and phagocytosis by the host but also mod-ulates their microenvironment and reduces the effective-ness of many antibiotics. The localized high cell densities present within the biofilm communities also initiate pro-duction, by the colonizing organism, of extracellular virulence factors such as toxins, proteases and siderophores (low molecular weight ligands responsible for the solubi-lization and transport of iron(III) in microbial cells). These are associated with a phenomenon termed quorum sensing (see section 4.2) and help the pathogen to combat the host’s innate defences and also promote the acquisition of nutrients.
Viruses are incapable of growing extracellularly and must therefore rapidly gain entry to cells (normally epi-thelial) at their initial site of entry. Once internalized in the non-immune host, they are to a large extent protected against the non-specific host defences. Following these initial consolidation events, the organisms may expand into surrounding tissues and/or disperse, via the blood, plasma, lymph or nerves, to distant tissues in order to establish secondary sites of infection or to consolidate further. In some instances microorganisms are able to colonize the host indefinitely and remain viable for many years (e.g. the herpesvirus varicella zoster which causes chickenpox, and herpes simplex virus 1 responsible for cold sores), but more generally they succumb to the heightened defences of the host, and in order to survive must either infect other individuals or survive in the general environment.
Even though some parts of the human body are free from microorganisms (axenic state), the body harbours millions of mutualistic and commensal symbionts. Each of us unwittingly carries a population of bacterial cells on our skin and in our mouth and digestive tract that out-numbers cells carrying our own genome by approxi-mately ten to one. Mutualistic relationships occur when both organisms (microbial and host cell) benefit from the interactions, whereas commensalism is a state in which one member of the relationship benefits without signifi-cantly affecting the other. Microorganisms that colonize body surfaces (internal and external) without normally causing disease constitute the microbiome. The microbi-ome comprises a number of distinct microbiotas which are characteristic of the region of the body colonized.
The microbiome is present throughout life and may comprise predominantly bacteria, with some fungi and protozoa, the majority of which are commensal. The microbiome begins to develop when the amniotic membrane surrounding the unborn child ruptures, allow-ing contact with vaginal, faecal and skin-associated microorganisms from the mother during childbirth. Microorganisms enter the infant’s mouth and nose during passage through the birth canal, colonization of the upper respiratory tract occurs with the first breath of air, and the beginning of the colon microbiota occurs during feeding. The development of the resident micro-biota is therefore initiated during the first few months of life. By comparison, transient microbiotas remain in the body for only a few hours, days or months. They are found in the same locations as the resident flora, but cannot persist because of their inability to compete with the microbiome, to resist elimination by the body’s defence mechanisms or to tolerate the chemical and physical changes encountered.
Changes in relative abundance of normal microbiota, for whatever reason (e.g. major changes to diet, antibiotic treatment, hormonal changes, chemo-or radiotherapy), may allow one or more members of the microbiome to become opportunistic pathogens. For example, reduc-tions in numbers of protective lactobacilli within the vagina brought about through antibiotic use can allow Candida albicans, a minority member of the normal microbiota, to grow more prolifically, resulting in an opportunistic vaginal yeast infection such as vaginal candidiasis. Following appropriate treatment, the normal flora is typically re-established in adults. In some areas ofthe body, such as the gastrointestinal tract, it is claimed that the recolonization by desired species can be encouraged by administration of probiotics. These are live cultures of intestinal bacteria that are marketed as conferring a health benefit and preventing digestive problems. Prebiotics, (normally fibre-like carbohydrates) that represent preferred or selective growth substrates for probiotic bacteria already present within the digestive tract are also of demonstrable benefit.
3.1 Skin
The part of the body that is most widely exposed to microorganisms is the skin. Intact skin is usually imper-vious to microorganisms, and its surface is of acid pH and contains relatively few nutrients that are favourable for microbial growth. The vast majority of organisms falling on to the skin surface will die, while the survivors must compete with the commensal microflora for nutrients in order to grow. These commensals, which include coryneform bacteria, staphylococci and yeasts, derive nutrients from compounds like urea, hormones (e.g. testosterone) and fatty acids found in the apocrine and epocrine secretions. Such organisms are highly adapted to growth in this environment and will normally prevent the establishment of chance contaminants of the skin. Infections of the skin itself, such as ringworm (Trichophyton mentagrophytes) and warts (human papil-lomavirus, HPV), rarely, if ever, involve penetration of the epidermis. Infection can, however, occur through the skin following trauma such as burns, cuts and abrasions and, in some instances, through insect or animal bites or the injection of contaminated medicines. In recent years extensive use of intravascular and extravascular medical devices and implants has led to an increase in the occur-rence of hospital-acquired infection. Commonly, these infections involve the growth of skin commensals such as Staph. epidermidis when associated with devices that pen-etrate the skin barrier. The organism grows as an adhesive biofilm on the surfaces of the device, and infection arises either from contamination of the device during its implantation or by growth along it of the organism from the skin. In such instances the biofilm sheds bacterial cells to the body and may give rise to a bacteraemia (the pres-ence of bacteria in the blood).
The weak spots, or Achilles heels, of the body occur where the skin ends and mucous epithelial tissues begin (mouth, anus, eyes, ears, nose and urinogenital tract). These mucous membranes present a much more favourable environment for microbial growth than the skin, in that they are warm, moist and rich in nutrients. Such membranes, nevertheless, possess certain characteristics that allow them to resist infection. Most of them, for example, possess their own highly adapted microbiotas (see section 2) which reduce the chances of infection by any invading organisms through a process termed coloni-zation resistance. The resident flora varies greatly between different sites of the body, but is usually common to particular host species. Each site can be additionally pro-tected by physicochemical barriers such as extreme acid pH in the stomach and bile salts in the large bowel, the presence of freely circulating non-specific antibodies and/or opsonins, and/or by macrophages and phagocytes (see Chapter 9). All infections start from contact between these tissues and the potential pathogen. Contact may be direct, from an infected individual to a healthy one; or indirect, and may involve inanimate vectors such as soil, food, drink, air and airborne particles. These may directly contact the body or be ingested or inhaled or enter wounds via infected bed linen and clothing. Indirect contact may also involve animal vector intermediates (carriers).
3.2 Respiratory tract
Air contains a large amount of suspended organic matter and, in enclosed occupied spaces, may hold up to 1000 microorganisms/m 3. Almost all of these airborne organ-isms are non-pathogenic bacteria and fungi, of which the average person inhales approximately 10 000/day. The respiratory tract is protected against this assault by a mucociliary blanket that envelops the upper respiratory tract and nasal cavity. Both present a tortuous path down which microbial particles travel and impact on these sur-faces to become trapped within an enveloping blanket of mucus. Beating cilia move the mucus coating to the back of the throat where it, together with adherent particles, is swallowed. The alveolar regions of the lower respiratory tract have additional protection in the form of alveolar macrophages. To be successful, a pathogen must avoid being trapped in the mucus and swallowed; if deposited in the alveolar sacs it must avoid phagocytosis by macro-phages, or if phagocytosed it must resist subsequent digestion by them. The possession of surface adhesins, specific for epithelial receptors, aids attachment of the invading microorganism and avoidance of removal by the mucociliary blanket. Other strategies include the export of ciliostatic toxins (i.e. C orynebacterium diphtheriae) that paralyse the cilial bed. As the primary defence of the respiratory tract is the mucociliary blanket, it is easy to envisage how infection with respiratory viruses (i.e. influ-enza virus, which kills respiratory epithelia) or the chronic inhalation of tobacco smoke, which increases mucin production and decreases the proportion of cili-ated epithelial cells, increases the susceptibility of individuals to infection.
3.3 Intestinal tract
The intestinal tract must contend with whatever it is given in terms of food and drink. The extreme acidity and presence of digestive enzymes in the stomach will kill many of the bacteria challenging it, and the gastrointes-tinal tract carries its own commensal flora of yeast and lactobacilli that afford protection by, for example, com-peting with potential pathogens like Helicobacter pylori. Bile salts are mixed with the semidigested solids exiting from the stomach into the small intestine. These salts not only neutralize the stomach acids but also represent bio-logical detergents or surfactants that are able to solubilize the outer membrane of many Gram-negative bacteria. Consequently, the small intestine is normally colonized by lower numbers of bacteria than the colon. The lower gut on the other hand is highly populated by commensal microorganisms (10 12 g −1 gut tissue) that are often associ-ated with the intestinal wall, either embedded in layers of protective mucus or attached directly to the epithelial cells or attached to particulate food residues. The patho-genicity of incoming bacteria and viruses depends on their ability to survive passage through the stomach and duodenum and their capacity for attachment to, or pen-etration of, the gut wall, despite competition from the commensal flora and the presence of secretory antibodies (Chapter 9).
3.4 Urinogenital tract
In healthy individuals, the bladder, ureters and urethra are sterile, and sterile urine constantly flushes the urinary tract. Organisms invading the urinary tract must avoid being detached from the epithelial surfaces and washed out during urination. Because the male urethra is long (c.2 0 cm), bacteria must be introduced directly into the bladder, possibly through catheterization, in order to ini-tiate an infection. The female urethra is much shorter (c. 5 cm) and is more readily traversed by microorganisms that are normally resident within the vaginal vault. Bladder infections are therefore much more common in the female. Spread of the infection from the bladder to the kidneys can easily occur through reflux of urine into the ureter. As for the implantation of devices across the skin barrier (above), long-term catheterization of the bladder will promote the occurrence of bacteriuria (the presence of bacteria in the urine) with all of the associated complications.
Lactic acid in the vagina gives it an acidic pH (5.0); this, together with other products of metabolism, inhibits colonization by most bacteria, except some lactobacilli that constitute the commensal flora. Other types of bac-teria are unable to establish themselves in the vagina unless they have become extremely specialized. These species of microorganism tend to be associated with sexually transmitted infections.
3.5 Conjunctiva
The conjunctiva is usually free of microorganisms and protected by the continuous flow of secretions from lach-rymal and other glands, and by frequent mechanical cleansing of its surface by the eyelid periphery during blinking. Lachrymal fluids contain a number of inhibitory compounds, together with lysozyme, that can enzymically degrade the peptidoglycan of Gram-positive bacteria such as staphylococci. Damage to the conjunctiva, caused through mechanical abrasion or reductions in tear flow, will increase microbial adhesion and allow colonization by opportunist pathogens. The likelihood of infection is thus promoted by the use of soft and hard contact lenses, physical damage, exposure to chemicals, or damage and infection of the eyelid border (blepharitis).
To be successful, a pathogen must be able to survive at its initial portal of entry, frequently in competition with the commensal flora and generally while subject to the attention of macrophages and wandering white blood cells. Such survival invariably requires the organism to attach itself firmly to the epithelial surface, eventually forming a biofilm. The initial attachment must be highly specific in order to displace the commensal microflora, and sub-sequently governs the course of an infection. Attachment can be mediated through provision, on the bacterial surface, of adhesive substances such as mucopeptide and mucopolysaccharide slime layers, fimbriae (Chapter 3), pili (Chapter 3) and agglutinins (Chapter 9). These are often highly specific in their binding characteristics, dif-ferentiating, for example, between the tips and bases of villi in the large bowel and the epithelial cells of the upper, mid and lower gut. Secretory antibodies, which are directed against such adhesions, block the initial attachment of the organism and thereby confer resistance toinfection.
The outcome of the encounter between the tissues and potential pathogens is governed by the ability of the microorganism to multiply at a faster rate than it is removed from those tissues. Factors that influence this are the organism’s rate of growth, the initial number arriving at the site and their ability to resist the efforts of the host tissues at removing/starving/killing them. The outcome of an encounter between a microorganism and a host can therefore be described as a balance between the accumulation of the pathogen and its elimination by the host.
The definition of virulence (i.e. the degree of pathogenicity caused by a microorganism) for pathogenic microorganisms must include the MIN. This will vary between individuals, but will invariably be lower in com-promised hosts such as those who are catheterized, dia-betics, smokers and cystic fibrosis patients, and those suffering trauma such as malnutrition, chronic infection or physical damage. A number of the individual factors that contribute towards virulence are discussed below.
4.1 Nutrient acquisition
Because the initial inoculum of a pathogen is usually too small to cause immediate damage to the host, it must acquire sufficient nutrients to allow it to multiply and increase in number. Not all nutrients, vitamins or growth factors are soluble and present in adequate quantities to allow pathogens to multiply. Moreover, trace elements may also be in short supply and can influence establish-ment of the pathogen. One such example is that of iron (Fe 3+) which is essential for microbial growth and func-tion. Normally this is complexed with host iron-binding proteins such as lactoferrin or transferrin, resulting in insufficient iron being made available to the pathogen. In order to survive and multiply, pathogens need to be able to compete with the host (and normal microbiota) for iron. Some bacteria can do this through production of iron-chelating compounds called siderophores which have a greater affinity for iron than the host’s iron-binding proteins, while others secrete hydrolytic enzymes that release iron from the host. Some organisms, such as Ps. aeruginosa, possess three or more different mechanisms of iron capture, giving them great versatility.
4.2 Biofilms
Cell attachment, and subsequent biofilm formation, is a means by which pathogens can remain in a favourable environment (i.e. one where there are plenty of nutrients) without getting washed away. As a consequence, bacterial cell numbers and activities can become quite high. Biofilms can form on any surface (e.g. soft tissue, bone, medical implants) and may contain only one or two species (e.g. S taph. aureus mediated osteomyelitis) or more commonly several species of bacteria (e.g. dental plaque). Hence, biofilms may be considered as a func-tional microbial community. Within a biofilm intracel-lular signalling molecules (e.g. N-acyl homoserinelactones) are produced that when sufficient (threshold) concentrations are reached, upregulate biofilm-specific genes. This process is known as quorum sensing, and is responsible for the formation and maintenance of the biofilm.
Once formed, biofilms can be extremely difficult, if not impossible, to remove. Their size and morphology can help protect the underlying cells, resisting physical forces or removal, phagocytosis and penetration of toxic mole-cules such as antibiotics. In addition, biofilms allow cells to live in close proximity to each other, thereby facilitating intercellular communication and genetic exchange. Finally, due to their profound resistance, biofilms provide foci of infection which often can only be removed by surgery.
4.3 Resistance to host defences
Most bacterial infections confine themselves to the surface of epithelial tissue (e.g. B ordetella pertussis, Corynebacterium diphtheriae, Vibrio cholerae). This is, to a large extent, a reflection of their inability to combat that host ’ s deeper defences. Survival at these sites is largely due to firm attachment to the epithelial cells. Such organisms manifest disease through the production and release of toxins (see below).
Other groups of organisms regularly establish systemic infections after traversing the epithelial surfaces (e.g. Brucella abortus, Salmonella enterica serovar Typhi, Streptococcus pyogenes). This property is associated with their abilities either to gain entry into susceptible cells and thereby enjoy protection from the body’s defences, or to be phagocytosed by macrophages or polymorphs and yet resist their lethal action and multiply within them. Other organisms are able to multiply and grow freely in the body’s extracellular fluids. Microorganisms have evolved a number of different strategies that allow them to suppress the host’ s normal defences and thereby survive in the tissues.
4.3.1 Modulation of the inflammatory response
Growth of microorganisms releases cellular products into their surrounding medium, many of which cause non-specific inflammation associated with dilation of blood vessels. This increases capillary flow and access of phago-cytes to the infected site. Increased lymphatic flow from the inflamed tissues carries the organisms to lymph nodes where further antimicrobial and immune forces come into play (Chapter 9). Many of the substances that are released by microorganisms in this fashion are chem-otactic towards polymorphs that tend, therefore, to become concentrated at the site of infection: this is in addition to the inflammation and white blood cell accu-mulation that is associated with antibody binding and complement fixation (Chapter 9). Many organisms have adapted mechanisms that allow them to overcome these initial defences. Thus, virulent strains of Staph. aureus produce a mucopeptide (peptidoglycan), which suppresses early inflammatory oedema, and related factors that suppress the chemotaxis of polymorphs.
4.3.2 Avoidance of p hagocytosis
Resistance to phagocytosis is sometimes associated with specific components of the cell wall and/or with the pres-ence of capsules surrounding the cell wall. Classic exam-ples of these are the M-proteins of the streptococci, the polysaccharide capsules of the pneumococci and the alginate-like slime associated with Ps. aeruginosa infec-tions of the cystic-fibrotic lung. The acidic polysaccharide K-antigens of Escherichia coli and S. enterica serovar Typhi behave similarly, in that (1) they can mediate attachment to the intestinal epithelial cells, and (2) they render phagocytosis more difficult. Generally, possession of an extracellular capsule and/or slime will reduce the likelihood of phagocytosis.
Microorganisms are more readily phagocytosed when coated with antibody (opsonized). This is due to the presence on the white blood cells of receptors for the Fc fragment of IgM and IgG (discussed in Chapter 9). Avoidance of opsonization will clearly enhance the chances of survival of a particular pathogen. A substance called protein A is released from actively growing strains of Staph. aureus, which acts by non-specific binding to IgG at the Fc region (see also Chapter 9), at sites both close to, and remote from, the bacterial surface. This blocks the Fc region of bound antibody masking it from phagocytes. Protein A-IgG complexes remote from the infection site will also bind complement, thereby deplet-ing it from the plasma and negating its actions near to the infection site.
4.3.3 Survival following phagocytosis
Death of microorganisms following phagocytosis can be avoided if the microorganisms are not exposed to the killing and digestion processes within the phagocyte. This is possible if fusion of the lysosomes with phagocytic vacuoles can be prevented. Such a strategy is employed by virulent Mycobacterium tuberculosis, although the precise mechanism is unknown. Other bacteria seem able to grow within the vacuoles despite lysosomal fusion (Listeria monocytogenes, S. enterica serovar Typhi). This can be attributed to cell wall components that prevent access of the lysosomal substances to the bacterial membranes (e.g. Brucella abortus, mycobacteria) or to the production of extracellular catalase which neutralizes the hydrogen peroxide liberated in the vacuole (e.g. staphylococci).
If microorganisms are able to survive and grow within phagocytes, they will escape many of the other body defences such as the lymph nodes, and be distributed around the body. As the lifespan of phagocytes is relatively short, such bacteria will eventually be delivered to the liver and gastrointestinal tract where they are ‘recycled ’.
4.3.4 Killing of phagocytes
An alternative strategy is for the microorganism to kill the phagocyte. This can be achieved by the production of leucocidins (e.g. staphylococci, streptococci) which promote the discharge of lysosomal substances into the cytoplasm of the phagocyte rather than into the vacuole, thus directing the phagocyte’s lethal activity towards itself.
Once established, the course of a bacterial infection can proceed in a number of ways. These can be related to the relative ability of the organism to penetrate and invade surrounding tissues and organs. The vast majority of pathogens, being unable to combat the defences of the deeper tissues, consolidate further on the epithelial surface. Others, including most viruses, penetrate the epithelial layers, but no further, and can be regarded as partially invasive. A small group of pathogens are fully invasive. These permeate the subepithelial tissues and are circulated around the body to initiate secondary sites of infection remote from the initial portal of entry (Figure 7.1).
Other groups of organisms may cause disease through ingestion by the victim of substances produced during microbial growth on foods. Such diseases may be regarded as intoxications rather than as infections and are consid-ered later (section 6.1.1). Treatment in these cases is usually an alleviation of the harmful effects of the toxin rather than elimination of the pathogen from the body.
5.1 Non-invasive pathogens
Bordetella pertussis (the aetiological agent of whooping cough) is probably the best described of these pathogens. This organism is inhaled and rapidly localizes on the mucociliary blanket of the lower respiratory tract. This localization is very selective and is thought to involve agglutinins on the organism’s surface. Toxins produced by the organism inhibit ciliary movement of the epithelial surface and thereby prevent removal of the bacterial cells to the gut. A high molecular weight exotoxin is also pro-duced during the growth of the organism which, being of limited diffusibility, pervades the subepithelial tissues to produce inflammation and necrosis. Corynebacterium diphtheriae (the causal organism of diphtheria) behaves similarly, attaching itself to the epithelial cells of the res-piratory tract. This organism produces a diffusible toxin of low molecular weight, which enters the blood circula-tion and brings about a generalized toxaemia.
In the gut, many pathogens adhere to the gut wall and produce their effect via toxins that pervade the surround-ing gut wall or enter the systemic circulation. V. cholerae and some enteropathic E. coli strains localize on the gut wall and produce toxins that increase vascular permeabil-ity. The end result is a hypersecretion of isotonic fluids into the gut lumen, acute diarrhoea and, as a conse-quence, dehydration that may be fatal in young or elderly people. In all these instances, binding to epithelial cells is not essential but increases permeation of the toxin and prolongs the presence of the pathogen.
5.2 Partially invasive pathogens
Some bacteria, and most viruses, are able to attach to the mucosal epithelia and then penetrate rapidly into the epithelial cells. These organisms multiply within the pro-tective environment of the host cell, eventually killing it and inducing disease through erosion and ulceration of the mucosal epithelium. Typically, members of the genera Shigella and Salmonella utilize such mechanisms in infections of the gastrointestinal tract. These bacteria attach to the epithelial cells of the large and small intes-tines, respectively, and, following their entry into these cells by induced pinocytosis, multiply rapidly and pene-trate laterally into adjacent epithelial cells. The mecha-nisms for such attachment and movement are unknown but involve a transition from a non-motile to motile phe-notype. Some species of salmonellae produce, in addi-tion, exotoxins that induce diarrhoea (section 6.1.4). There are innumerable serotypes of Salmonella, which are primarily parasites of animals but are important to humans in that they colonize farm animals such as pigs and poultry and ultimately infect foods derived from them. Salmonella food poisoning (salmonellosis), therefore, is commonly associated with inadequately cooked meats, eggs and also with cold meat products that have been incorrectly stored following contact with the uncooked product. Dependent upon the severity of the lesions induced in the gut wall by enteric pathogens, red blood cells and phagocytes pass into the gut lumen, along with plasma, and cause the classic ‘bloody flux ’ of bacil-lary dysentery. Similar erosive lesions are produced by some enteropathic strains of E. coli.
Viral infections such as influenza and the ‘common cold’ (in reality 300-400 different strains of rhinovirus) infect epithelial cells of the respiratory tract and nasophar-ynx, respectively. Release of the virus, after lysis of the host cells, is to the void rather than to subepithelial tissues. The residual uninfected epithelial cells are rapidly infected, resulting in general degeneration of the tracts. Such damage not only predisposes the respiratory tract to infection with opportunist pathogens such as N. men-ingitidis and Haemophilus influenzae but also causes the associated fever.
5.3 Fully invasive pathogens
Invasive pathogens either aggressively invade the tissues surrounding the primary site of infection (active spread) or are passively transported around the body in the blood, lymph, cerebrospinal, axonal or pleural fluids (passive spread). Some, especially aggressive organisms, move both passively and actively, setting up multiple, expansive secondary sites of infection in various organs.
5.3.1 Active spread
Active spread of microorganisms through normal sub-epithelial tissues is difficult in that the gel-like nature of the intercellular materials physically inhibits bacterial movement. Induced death and lysis of the tissue cells produces, in addition, a highly viscous fluid, partly due to undenatured DNA. Physical damage, such as wounds, is rapidly sealed with fibrin clots, thereby reducing the effective routes for spread of opportunist pathogens. Organisms such as Strep. pyogenes, Cl. perfringens and, to some extent, the staphylococci, are able to establish them-selves in tissues by virtue of their ability to produce a wide range of extracellular enzyme toxins. These are associated with killing of tissue cells, degradation of intracellular materials and mobilization of nutrients. A selection of such toxins will be considered briefly.
• Haemolysins are produced by most of the pathogenic staphylococci and streptococci. They have a lytic effect on red blood cells, releasing iron-containing nutrients.
• Fibrinolysins are produced by both staphylococci (staphylokinase) and streptococci (streptokinase). These toxins indirectly activate plasminogen and so dissolve fibrin clots that the host forms around wounds and lesions to seal them. The production of fibrinolysins therefore increases the likelihood of the infection spreading. Streptokinase may be employed clinically in conjunction with streptodornase (Chapter 26) in the treatment of thrombosis.
• Collagenases and hyaluronidases are produced by most of the aggressive invaders of tissues. These are able to dissolve collagen fibres and the hyaluronic acids that function as intercellular cements; this causes the tissues to break up and produce oedematous lesions.
• Phospholipases are produced by organisms such as Cl. perfringens (α-toxin). These toxins kill tissue cells by hydrolysing the phospholipids that are present in cell membranes.
• Amylases, peptidases and deoxyribonucleases mobilize many nutrients that are released from lysed cells. They also decrease the viscosity of fluids present at the lesion by depolymerization of their biopolymer substrates.
Organisms possessing the above toxins, particularly those also possessing leucocidins, are likely to cause expanding oedematous lesions at the primary site of infection. In the case of Cl. perfringens, a soil microorgan-ism that has become adapted to a saprophytic mode of life, infection arises from an accidental contamination of deep wounds when a process similar to that seen during the decomposition of a carcass ensues (gangrene). This organism is most likely to spread through tissues when blood circulation, and therefore oxygen tension, in the affected areas is minimal.
Abscesses formed by streptococci and staphylococci can be deep-seated in soft tissues or associated with infected wounds or skin lesions; they become localized through the deposition of fibrin capsules around the infection site. Fibrin deposition is partly a response of the host tissues, but is also partly a function of enzyme toxins such as coagulase. Phagocytic white blood cells can migrate into these abscesses in large numbers to produce significant quantities of pus. Such pus, often carrying the infective pathogen, might be digested by other phago-cytes in the late stages of the infection or discharged to the exterior or to the capillary and lymphatic network. In the latter case, blocked capillaries might serve as sites for secondary lesions. Toxins liberated from the microorganisms during their growth in such abscesses can freely diffuse to the rest of the body to set up a generalized toxaemia.
S. enterica serovar Typhi, S. enterica serovar Paratyphi and S. enterica serovar Typhimurium are serotypes of Salmonella (section 4.2) that are not only able to pene-trate into intestinal epithelial cells and produce exotoxins, but are also able to penetrate beyond into subepithelial tissues. These organisms therefore produce a characteris-tic systemic disease (typhoid and enteric fever), in addi-tion to the usual symptoms of salmonellosis. Following recovery from such infection the organism is commonly found associated with the gallbladder. In this state, the recovered person will excrete the organism and become a reservoir for the infection of others.
5.3.2 Passive spread
When invading microorganisms have crossed the epithe-lial barriers they will almost certainly be taken up with lymph in the lymphatic ducts and be delivered to filtration and immune systems at the local lymph nodes. Sometimes this serves to spread infections further around the body. Eventually, spread may occur from local to regional lymph nodes and thence to the blood-stream. Direct entry to the bloodstream from the primary portal of entry is rare and will only occur when the organism damages the blood vessels or if it is injected directly into them. This might be the case following an insect bite or surgery. Bacteraemia such as this will often lead to secondary infections remote from the original portal of entry.
Damage caused to the host organism through infection can be direct and related to the destructive presence of microorganisms (or to their production of toxins) in particular target organs; or it can be indirect and related to interactions of the antigenic components of the pathogen with the host’s immune system. Effects can therefore be closely related to, or remote from, the infected organ.
Symptoms of the infection can in some instances be highly specific, relating to a single, precise pharmacologi-cal response to a particular toxin; or they might be non-specific and relate to the usual response of the body to particular types of trauma. Damage induced by infection will therefore be considered in these categories.
6.1 Direct damage
6.1.1 Specific effects
For the host, the consequences of infection depend to a large extent upon the tissue or organ involved. Soft tissue infections of skeletal muscle are likely to be less damaging than, for instance, infections of the heart muscle and central nervous system. Infections associated with the epithelial cells that make up small blood vessels can block or rupture them to produce anoxia or necrosis in the tissues that they supply. Cell and tissue damage is generally the result of a direct local action by the microorganisms, usually concerning action at the cyto-plasmic membranes. The target cells are usually phago-cytes and are generally killed (e.g. by Brucella, Listeria, Mycobacterium). Interference with membrane function through the action of enzymes such as phospholipase causes the affected cells to leak. When lysosomal membranes are affected, the lysosomal enzymes disperse into the cells and tissues causing them, in turn, to autolyse. This is mediated through the vast battery of enzyme toxins available to these organisms (section 4). If these toxins are produced in sufficient concentration they may enter the circulatory systems to produce a generalized toxaemia. During their growth, other pathogens liberate toxins that possess very precise, singular pharmacological actions. Diseases mediated in this manner include diph-theria, tetanus and scarlet fever.
In diphtheria, the organism C. diphtheriae confines itself to epithelial surfaces of the nose and throat and produces a powerful toxin which affects an elongation factor involved in eukaryotic protein biosynthesis. The heart and peripheral nerves are particularly affected, resulting in myocarditis (inflammation of the myocar-dium) and neuritis (inflammation of a nerve). Little damage is produced at the infection site.
Tetanus occurs when Cl. tetani, ubiquitous in the soil and the faeces of herbivores, contaminates wounds, especially deep puncturetype lesions. These might be the result of a minor trauma such as a splinter, or a major one such as a motor vehicle accident. At these sites, tissue necrosis,and possibly also microbial growth, reduce the oxygen tension to allow this anaerobe to multiply. Its growth is accompanied by the production of a highly potent toxin that passes up peripheral nerves and diffuses locally within the central nervous system. The toxin has a strychnine-like action and affects normal function at the synapses. As the motor nerves of the brainstem are the shortest, the cranial nerves are the first affected, with twitches of the eyes and spasms of the jaw (lockjaw).
A related organism, Cl. botulinum, produces a similar toxin that may contaminate food if the organism has grown in it and if conditions are favourable for anaerobic growth. Meat pastes and p ât és are likely sources. This toxin interferes with acetylcholine release at cholinergic synapses and also acts at neuromuscular junctions. Death from this toxin eventually results from respiratory failure.
Many other organisms are capable of producing intox-ication following their growth on foods. Most common among these are the staphylococci and strains of B. cereus. Some strains of Staph. aureus produce an enterotoxin which acts on the vomiting centres of the brain. Nausea and vomiting therefore follow ingestion of contaminated foods and the delay between eating and vomiting varies between 1 and 6 hours depending on the amount of toxin ingested. B. cereus also produces an emetic toxin but its actions are delayed and vomiting can follow up to 20 hours after ingestion. The latter organism is often associated with rice products and will propagate when the rice is cooked (spore activation) and subsequently reheated after a period of storage.
Scarlet fever is produced following infection with certain strains of Strep. pyogenes. These organisms produce a potent toxin that causes an erythrogenic skin rash that then accompanies the more usual effects of a streptococcal infection.
6.1.2 Non-specific effects
If the infective agent damages an organ and affects its functioning, this can manifest itself as a series of secondary disease features that reflect the loss of that function to the host. Thus, diabetes may result from an infection of the islets of Langerhans, paralysis or coma from infec-tions of the central nervous system, and kidney malfunc-tion from loss of tissue fluids and its associated hyperglycaemia. In this respect virus infections almost inevitably result in the death and lysis of the host cells. This will result in some loss of function by the target organ. Similarly, exotoxins and endotoxins can also be implicated in non-specific symptoms, even when they have well-defined pharmacological actions. Thus, a number of intestinal pathogens (e.g. V. cholerae, E. coli) produce potent exotoxins that affect vascular permeability. These generally act through adenylate cyclase, raising the intracellular levels of cyclic AMP (adenosine mono-phosphate). As a result of this the cells lose water and electrolytes to the surrounding medium, the gut lumen. A common consequence of these related, yet distinct, toxins is acute diarrhoea and haemoconcentration. Kidney malfunction might well follow and in severe cases lead to death. Symptomologically there is little difference between these conditions and the food poisoning induced by ingestion of staphylococcal enterotoxin (above).
6.2 Indirect damage
Inflammatory materials are released not only from necrotic cells but also directly from the infective agent. Endotoxins are derived from constituents of the bacterial cell rather than being deliberately exported cellular prod-ucts. Thus, during the growth and autolysis of Gram-negative bacteria components of their cell envelopes, such as lipopolysaccharide (see Chapter 3) are shed to the environment. Endotoxins tend to be less toxic than exo-toxins and have much less precise pharmacological actions. Indeed, it is not always clear to what extent these can be related to actions by the host or by the pathogen. Reactions include local inflammation, elevations in body temperature, aching joints, and head and kidney pain. Inflammation causes swelling, pain and reddening of the tissues, and sometimes loss of function of the organs affected. These reactions may sometimes be the major sign and symptom of the disease.
While various toxic effects have been attributed to these endotoxins, their role in the establishment of the infection, if any, remains unclear. The most notable effect of these materials is their ability to induce a high body temperature (pyrogenicity) (Chapters 3 and 22). The pyrogenic effect of lipopolysaccharide relates to the action of the lipid A component directly upon the hypothalamus and also to its direct action on macro-phages and phagocytes. Elevation of body temperature follows within 1-2 hours. In infections such as meningitis the administration of antibiotics may cause such a release of pyrogen that the resultant inflammation and fever is fatal. In such instances antibiotics are co-administered with steroids to counter this effect. The pyrogenic effects of lipid A are unaffected by moist heat treatment (auto-clave). Growth of Gram-negative organisms such as Pseudomonas aeruginosa in stored water destined for use in terminally sterilized products will cause the final product to be pyrogenic. Processes for the destruction of pyrogen associated with glassware, and tests for the absence of pyrogen in water and product, therefore form an important part of parenteral drug manufacture (Chapter 22).
Many microorganisms minimize the effects of the host’s defence system against them by mimicking the antigenic structure of the host tissue. The eventual immunological response of the host to infection then leads to its autoimmune self-destruction. Thus, infections with Mycoplasma pneumoniae can lead to production of antibody against normal group O erythrocytes, with concomitant haemolytic anaemia.
If antigen released from the infective agent is soluble, antigen-antibody complexes are produced. When anti-body is present at a concentration equal to or greater than the antigen, such as in the case of an immune host, these complexes precipitate and are removed by macrophages present in the lymph nodes. When antigen is present in excess, the complexes, being small, continue to circulate in the blood and are eventually filtered off by the kidneys, becoming lodged in kidney glomeruli and in the joints. Localized inflammatory responses in the kidneys are sometimes then initiated by the complement system (Chapter 9). Eventually the filtering function of the kidneys becomes impaired, producing symptoms of chronic glomerulonephritis.
7 Recovery from infection: exit of microorganisms
The primary requirement for recovery is that multiplication of the infective agent is brought under control, so that it ceases to spread around the body and that the damaging consequences of its presence are arrested and repaired. Such control is brought about by the combined functions of the phagocytic, immune and complement systems. A successful pathogen will not seriously debili-tate its host; rather, the continued existence of the host must be ensured in order to maximize the dissemination of the pathogen within the host population. From the microorganism’s perspective the ideal situation is where it can persist permanently within the host and be constantly released to the environment. Although this is the case for a number of virus infections (chickenpox, herpes) and for some bacterial ones, it is not common. Generally, recovery from infection is accompanied by complete destruction of the organism and restoration of a sterile tissue. Alternatively, the organism might return to a commensal relationship with the host on the epithelial and skin surface.
Where the infective agent is an obligate pathogen, a means must exist for it to infect other individuals before its eradication from the host organism. The route of exit is commonly related to the original portal of entry (Figure 7.1). Thus, pathogens of the intestinal tract are liberated in the faeces and might easily contaminate food and drinking water. Infective agents of the respiratory tract might be exhaled during coughing, sneezing or talking, survive in the associated water droplets, and infect nearby individuals through inhalation. Infective agents transmitted by insect and animal vectors may be spread through those same vectors, the insects/animals having been themselves infected by the diseased host. For some ‘fragile’ organisms (e.g. N. gonorrhoeae, T. palli-dum), direct contact transmission is the only means of spread between individual hosts. In these cases, intimate contact between epithelial membranes, such as occurs during sexual contact, is required for transfer to occur. For opportunist pathogens, such as those associated with wound infections, transfer is less important because the pathogenic role is minor. Rather, the natural habitat of the organism serves as a constant reservoir for infection.
8 Epidemiology of infectious disease
Spread of a microbial disease through a population of individuals can be considered as vertical (transferred from one generation to another) or horizontal (transfer occurring within genetically unrelated groups). The latter can be divided into common source outbreaks, relating to infection of a number of susceptible individuals from a single reservoir of the infective agent (i.e. infected foods), or propagated source outbreaks, where each individual provides a new source for the infection of others.
Common source outbreaks are characterized by a sharp onset of reported cases over the course of a single incubation period (Figure 7.2) and relate to a common experience of the infected individuals (e.g. a contami-nated food product). The number of cases will persist until the source of the infection is removed. If the source remains (i.e. a reservoir of insect vectors) then the disease becomes endemic to the exposed population, with a constant rate of infection. Propagated source outbreaks, on the other hand, are brought about by person-to-person spread, and show a gradual increase in reported cases over a number of incubation periods and eventually decline when most of the susceptible individuals in the population have been affected (Figure 7.2). Factors that contribute to propagated outbreaks of infectious disease are the infectivity of the agent (I), the population density (P) and the numbers of susceptible individuals in it (F). The likelihood of an epidemic occurring is given by the product of these three factors (i.e. FIP). An increase in any one of them might initiate an outbreak of the disease in epidemic proportions. Thus, reported cases of particu-lar diseases show periodicity, with outbreaks of epidemic proportion occurring only when FIP exceeds certain critical threshold values, related to the infectivity of the agent. Outbreaks of measles and chickenpox therefore tend to occur annually in the late summer among children attending school for the first time. This has the effect of concentrating all susceptible individuals in one, often confined, space at the same time. The proportion of susceptible individuals can be reduced through rigorous vaccination programmes (Chapter 9). Provided that the susceptible population does not exceed the threshold FIP value, then herd immunity against epidemic spread of the disease will be maintained.
Figure 7.2 Comparison of timescale for common source outbreak and propagated source outbreak infections.
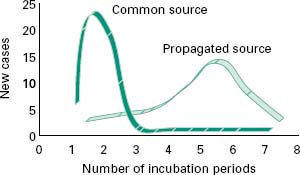
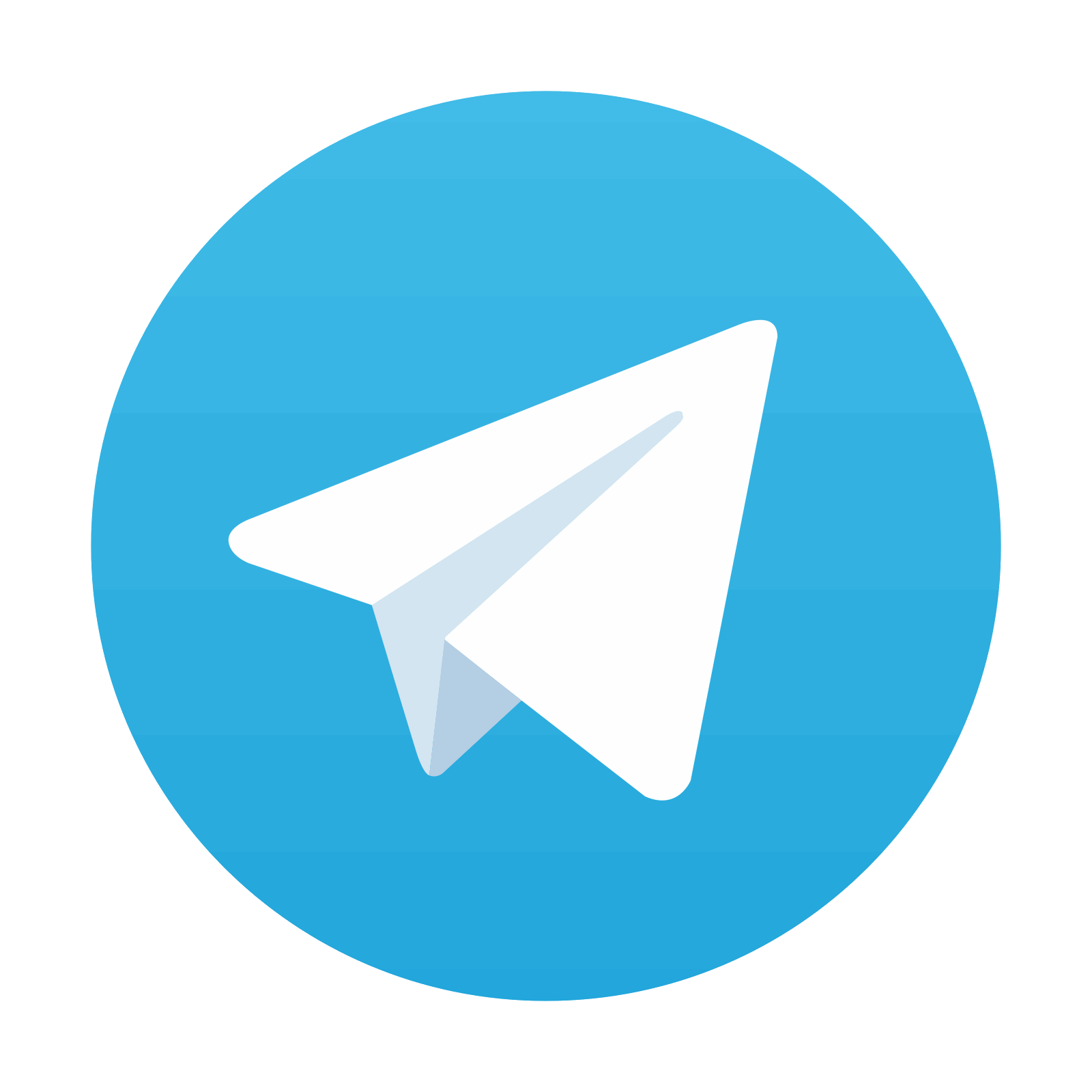