Chapter 45 Principles of Antimicrobial Use
Abbreviations | |
---|---|
CNS | Central nervous system |
CSF | Cerebrospinal fluid |
DNA | Deoxyribonucleic acid |
IM | Intramuscular |
IV | Intravenous |
MBC | Minimal bactericidal concentration |
MIC | Minimal inhibitory concentration |
MRSA | Methicillin-resistant Staphylococcus aureus |
PBP | Penicillin binding proteins |
PO | Oral |
RNA | Ribonucleic acid |
Viruses, bacteria, other unicellular organisms, and multicellular organisms in the environment can also live in the human body. This relationship can produce desirable and undesirable responses within the host. In a healthy person, normal bacteria in the gastrointestinal tract have beneficial effects, assisting in the production of vitamins and the breakdown of foods. However, when nonbeneficial, pathogenic organisms enter the body through oral ingestion, inhalation, trauma, surgical procedures, or any body opening, the result is an unwanted host-pathogen response, termed an infection. The responses to an infection can occur either directly through the release of toxins or antigens by the invading organism or indirectly through tissue invasion or generation of an inflammatory response by the body. Many infections develop as a result of noninfectious primary diseases that suppress the patient’s natural immune response. However, on a global scale, parasitic infections are the greatest cause of morbidity and mortality worldwide.
Table 45-1 lists the types of pathogenic organisms that invade the human body and cause unwanted biological responses. Treatment of the problems generated by these invasions is approached in two ways: (1) destruction or removal of invading organisms, and (2) alleviation of symptoms. The availability of drugs for successful eradication of invading organisms varies considerably with the type and location of the organisms within the human host.
TABLE 45–1 Pathological Organisms that Can Live in a Parasitic Invader-Host Relationship in Humans, Listed in Order of Increasing Complexity
Cell Type | Organism | Typical Size (nm) |
---|---|---|
Acellular | Viruses | 20-200 |
Unicellular | Chlamydia (P) | 1000 |
Mycoplasma (P) | 1000 | |
Rickettsia (P) | 1000 | |
Bacteria (P) | 1000 | |
Fungi: yeasts (E) | 3000-5000 | |
Protozoa (E) | ||
Multicellular | Fungi: molds (E) | 2000-10,000 and larger |
Helminths (E) |
P, Prokaryotes (no nuclear membrane); E, eukaryotes (with a nucleus).
The only acellular organisms known to induce infectious diseases in humans are viruses and proteinaceous agents lacking nucleic acids called prions. Other small molecular weight, acellular nucleic acids called viroids can exist in humans but probably do not contribute to disease. Bacteria are unicellular, nonnuclear organisms. Higher orders of size and complexity are found in unicellular, nucleated fungi (including yeast and filamentous forms) and protozoa. Major differences include the addition of a membrane-enclosed nucleus and mitochondria within the cell. More complex fungi are found in the multicellular, nucleated molds (see Chapter 50). Still higher orders of parasitic organisms are helminths (worms), which are estimated to infect 40% to 60% of the world’s population and are a medical problem in both industrialized and developing countries (see Chapter 52).
drugs available to halt proliferation of, or eliminate, clinically important viruses, although this situation is improving. Vaccines have provided the most effective protection against viruses; however, prophylactic use of some antiviral drugs has also proven effective in preventing certain viral infections (see Chapter 51).
The development of antimicrobial therapy is considered by many to be one of the most important advances in the history of medicine. Major historical events leading to the development of antimicrobial therapy are listed in Box 45-1. The term antibiotic traditionally refers to substances produced by microorganisms to suppress the growth of other microorganisms. The term antimicrobial agent is broader in meaning, because it encompasses drugs synthesized in the laboratory and those natural antibiotics produced by microorganisms. Some natural antibiotics produced originally by microbial fermentation are now produced by chemical synthesis. Many agents are semisynthetic; that is, the key portion of the compound is produced by microbial fermentation, and various moieties are attached synthetically. Thus the distinction between the terms antibiotics and antimicrobial agents is somewhat blurred and has little meaning today.
BOX 45–1 History of Antimicrobial Therapy
Early 17th Century | The first recorded successful use of antimicrobial therapy involving the use of an extract from cinchona bark for the treatment of malaria. |
1909 | Paul Ehrlich’s quest for a “magic bullet” that would bind specifically to particular sites on parasitic organisms leads to an arsenic derivative, salvarsan, with modest activity against syphilis. He also suggested that antimicrobial drugs would be most useful if the sites of action were not present in the organs and tissues of the human host. |
1929 | Alexander Fleming discovers penicillin. |
1935 | Discovery of prontosil, a forerunner of sulfonamides. |
1940 | Florey and Chain first use penicillin clinically. |
The efficacy and relative safety of these drugs has led to their widespread use and overuse, with up to 50% of antimicrobials consumed in the United States considered unnecessary. Because of their ability to alter microbial flora and lead to antibiotic-resistant microorganisms, antimicrobials are fundamentally different from other types of drugs. It is important to always remember that the pathogenicity of a bacterial species may mutate with time as new strains appear, existing strains develop resistance, old strains disappear, and new problems emerge with old strains thought to be relatively benign. This complicates planning drug treatment and requires the clinician to stay informed and use these agents wisely.
Antimicrobial agents can be classified into major groups according to the point in the cellular biochemical pathways at which they exert their primary mechanism of action (Fig. 45-1). These are:
Agents that inhibit the synthesis of cell walls include the β-lactams, such as penicillins, cephalosporins, monobactams, and carbapenems, and others, such as vancomycin. Inhibitors of cytoplasmic membranes include the polymyxins and daptomycin. In fungi the cell wall is damaged by the polyene antifungal drugs or by the azoles. Inhibitors of nucleic acid synthesis include the quinolones, which inhibit deoxyribonucleic acid (DNA) gyrase, and the ribonucleic acid (RNA) polymerase inhibitor rifampin. Protein synthesis is inhibited by aminoglycosides, tetracyclines, chloramphenicol, erythromycin, clindamycin, linezolid, and streptogramins. These agents are usually bacteriostatic, except the aminoglycosides and sometimes streptogramins, which can be bactericidal. Finally, folate antagonists such as sulfonamides and trimethoprim interfere with cell metabolism. These antimicrobial drugs are listed by their mechanisms of action in Table 45-2.
TABLE 45–2 Classification of Antimicrobial Agents by Mechanism of Action
Mechanism of Action | Agent | Discussed in Chapter |
---|---|---|
Inhibition of synthesis or damage to cell wall | Penicillins | 46 |
Cephalosporins | 46 | |
Monobactams | 46 | |
Carbapenems | 46 | |
Bacitracin | 46 | |
Vancomycin | 46 | |
Inhibition of synthesis or damage to cytoplasmic membrane | Polymyxins | 48 |
Amphotericin B | 50 | |
Modification of synthesis or metabolism of nucleic acids | Quinolones | 48 |
Rifampin | 49 | |
Nitrofurans | 48 | |
Inhibition or modification of protein synthesis | Aminoglycosides | 47 |
Tetracyclines | 47 | |
Chloramphenicol | 47 | |
Erythromycin | 47 | |
Clindamycin | 47 | |
Streptogramins | 47 | |
Linezolid | 47 | |
Mupirocin | 47 | |
Modification of energy metabolism | Sulfonamides | 48 |
Trimethoprim | 48 | |
Dapsone | 49 | |
Isoniazid | 49 |
DRUG SELECTION FOR INDIVIDUAL PATIENTS
Major factors to be considered when selecting an antimicrobial agent are outlined in Box 45-2. An understanding of these factors assists with rational selection of an appropriate compound. It is important to keep in mind that each factor is important, but their relative significance may vary from case to case.
BOX 45–2 Factors to Consider When Selecting Antimicrobial Agents for Therapy in Patients
Cost |
Toxicity |
Host factors |
Identification of the Pathogen
For many infections an attempt should be made to identify the possible pathogen or pathogens before initiating antimicrobial therapy. Specimens for direct inspection and culture should be obtained before therapy is started, because drug therapy can decrease the yield of culture. Gram staining is the fastest, simplest, and most inexpensive method to identify bacteria and fungi. Because a Gram stain can be accomplished quickly, the results can be used to guide the initial antimicrobial choice. Body fluids that are normally sterile should be Gram stained. In addition, stains of wound exudates, sputum, and fecal material can sometimes yield information about the infecting microorganisms.
Susceptibility of Infecting Microorganisms
Susceptibility testing is often performed by automated systems based on the broth dilution method in which antibiotics are tested in serial dilutions that encompass the concentrations normally achieved in humans. This method detects the lowest concentration of antimicrobial agent that prevents visible growth after incubation for 18 to 24 hours, referred to as the minimal inhibitory concentration (MIC). The technique is shown in Figure 45-2, A. Deciding whether the results demonstrate that the organisms are susceptible requires an understanding of the pharmacokinetics of the antimicrobial agent, correlations between clinical outcomes and MIC data, and knowledge of the relationship between resistance mechanisms and MICs. Determining the MIC “cutoff” for susceptibility is often not straightforward and is the purview of an international committee. This same test procedure can be extended further to determine the minimal bactericidal concentration (MBC), or minimal concentration that kills 99.9% of cells. In this test samples are removed from the antibiotic-containing tubes in which there is no visible microbial growth and plated on agar that contains no additional antibiotic. The MBC is the lowest concentration of antibiotic in the original tube from which bacteria do not grow on the agar (see Fig. 45-2, B). MBC determinations are no longer used regularly in most clinical laboratories.
Another method for determining bacterial susceptibility to antibiotics is the disk diffusion method, in which disks impregnated with the drugs to be tested are placed on an agar plate freshly inoculated with the bacterial strain in question. After the plates have been incubated for 18 to 24 hours, bacterial growth occurs everywhere except near the disks, where a “zone of inhibition” may be present. If sufficiently large, the zone indicates that the bacterial strain is susceptible to the antibiotic in the disk. This test is simple to perform but is only semiquantitative and not useful for determining the susceptibility of many slow-growing or fastidious organisms; it has been replaced by automated broth dilution systems in many laboratories. The test procedure and typical results are shown in Figure 45-3. A newer and related method is the E-test, which uses a strip containing the antibiotic in a concentration gradient along with a numerical scale instead of a disk. The point where the zone of inhibition touches the strip indicates the MIC (Fig. 45-4).
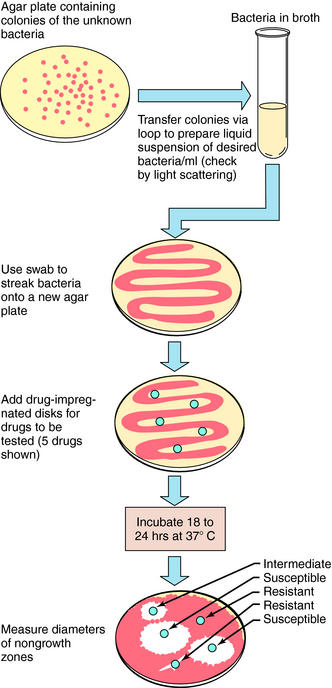
FIGURE 45–3 Disk diffusion method for testing bacteria for susceptibility to specific antimicrobial agents.
Need for Bactericidal versus Bacteriostatic Agents
Antimicrobial agents can be bactericidal (i.e., the organisms are killed) or bacteriostatic (i.e., the organisms are prevented from growing) (Fig. 45-5). A given agent may show bactericidal actions under certain conditions but bacteriostatic actions under others, depending on the concentration of drug and the target bacteria. A bacteriostatic agent often is adequate in uncomplicated infections, because the host defenses will help eradicate the microorganism. For example, in pneumonococcal pneumonia, bacteriostatic agents suppress the multiplication of the pneumococci, and the pneumococci are destroyed by interaction with alveolar macrophages and polymorphonuclear leukocytes. For a neutropenic individual, such a bacteriostatic agent might prove ineffective, and a bactericidal agent would be necessary. Thus the status of the host influences whether a bactericidal or bacteriostatic agent is selected.
Pharmacokinetic and Pharmacodynamic Factors
The amount of antimicrobial agent that reaches the extravascular tissues and fluids where the infection is usually present depends on basic pharmacokinetic principles (see Chapters 2 and Chapter 3). Considerable variation exists among the many antimicrobial agents, and the pharmacokinetic profile of the antimicrobials is an important factor in selecting the proper drug.
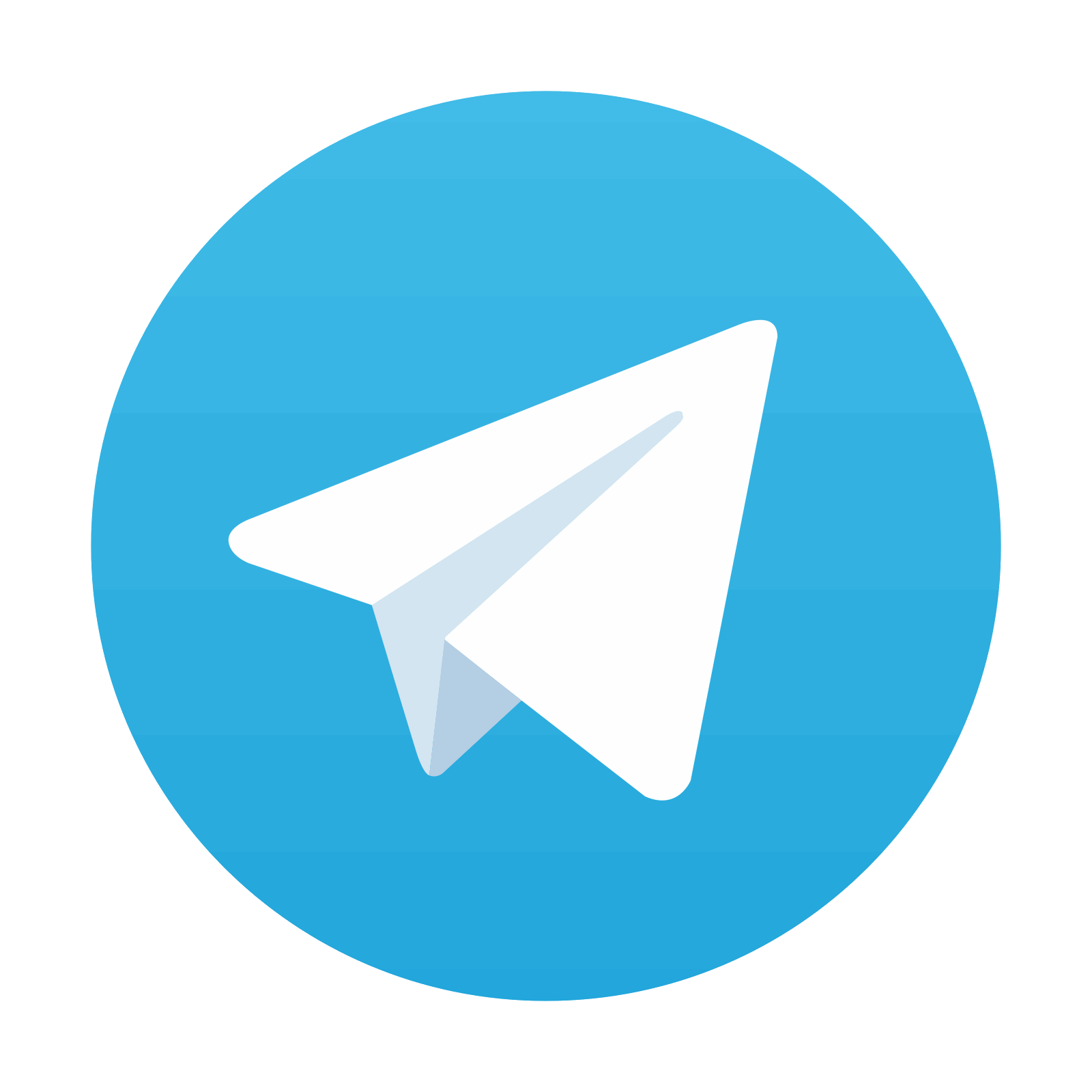
Stay updated, free articles. Join our Telegram channel

Full access? Get Clinical Tree
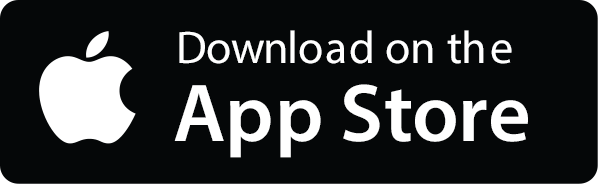
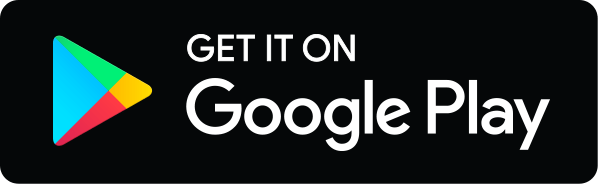
