David M. Dudzinski and Charles N. Serhan
Lipoxins, Aspirin-Triggered Lipoxins, Resolvins/Protectins/ Maresins, and Lipoxin-Stable Analogues | |
Autacoids are substances that are rapidly synthesized in response to specific stimuli, act quickly in the local environment, and remain active for only a short time before degradation. Eicosanoids represent a chemically diverse family of autacoids that are mostly derived from arachidonic acid. Research on eicosanoids has defined their vital roles in inflammatory, neoplastic, and cardiovascular physiology and pathophysiology. Numerous pharmacologic interventions in eicosanoid pathways—including the nonsteroidal anti-inflammatory drugs (NSAIDs), cyclooxygenase-2 (COX-2) inhibitors, leukotriene inhibitors, and others—are useful in the clinical management of inflammation, pain, and fever. Given the diverse bioactivities of eicosanoids, future research in eicosanoid physiology and pharmacology may lead to the development of new therapeutics for the inflammatory and autoimmune bases of asthma, glomerulonephritis, cancer, wound healing, cardiovascular diseases, and other clinical conditions.
Ms. G, a 44-year-old Native American female, visits her physician because of joint pain and progressive fatigue. Her history reveals general joint stiffness and pain for the past 3 weeks. The pain is worst in the metacarpophalangeal and proximal interphalangeal joints and is especially prominent in the early morning. Ms. G is advised to take ibuprofen as needed, and this medication provides relief of her pain for some time.
Six months later, Ms. G has continued to take ibuprofen daily and she notes indigestion and new vomiting of “coffee grounds”-like material. Her physician recommends cessation of ibuprofen and an upper gastrointestinal endoscopic examination, which reveals gastric mucosal erosion and hemorrhage. Her physician is also concerned about interval progression in Ms. G’s joint stiffness and pain and refers her to a rheumatology clinic. She reports to the rheumatologist that her pain has progressed to include both feet, both hands and wrists, both elbows, some cervical vertebrae, and the left shoulder. Over the past few months, she has hours of morning pain, has noted difficulty with basic household tasks, and has avoided physical activity. On examination, the metacarpophalangeal and proximal interphalangeal joints of both hands are found to be swollen, tender, and warm; Ms. G also has characteristic ulnar deviation of her fingers and “swan-neck” deformities. Skin nodules are apparent on the extensor surface of both forearms. Laboratory tests show an elevated erythrocyte sedimentation rate (ESR), low hemoglobin, and positive rheumatoid factor. Synovial fluid aspirate is notable for leukocytosis. Hand radiographs show erosion and osteopenia.
Because the symptoms, examination, laboratory studies, and radiographs are consistent with a diagnosis of moderately to severely active rheumatoid arthritis, Ms. G is initially started on a course of celecoxib (a COX-2 selective inhibitor), etanercept (a TNF-α antagonist), and prednisone (a glucocorticoid). Over the subsequent months, the joint pain, swelling, and tenderness decrease noticeably. Joint function in the hands is restored, and Ms. G is able to resume some physical activity.
Questions
1. Which eicosanoid mediators may be causing Ms. G’s joint pains?
2. By what mechanism do glucocorticoids such as prednisone affect eicosanoid levels and/or bioactivity?
3. By what mechanism did ibuprofen cause Ms. G’s gastric erosion and hemorrhage?
4. What are the potential concerns regarding long-term use of celecoxib?
5. By what mechanism does etanercept affect eicosanoid levels and/or bioactivity?
PHYSIOLOGY OF EICOSANOID METABOLISM
Eicosanoids are centrally involved in a number of metabolic pathways that exhibit diverse roles in inflammation and cellular signaling. The vast majority of these pathways center on reactions involving the metabolism of arachidonic acid (Fig. 43-1). The following section considers the biochemical steps leading to arachidonic acid generation and then discusses the cyclooxygenase, lipoxygenase, epoxygenase, and isoprostane pathways of arachidonic acid metabolism. The word eicosanoid arises from the Greek root meaning twenty, and the term classically refers to unbranched 20-carbon molecules derived from arachidonic acid oxygenation. The term eicosanoid also applies broadly to various other molecules—such as resolvins, protectins, and maresins—that are derived from docosahexaenoic acid, a 22-carbon precursor. The term docosanoids is sometimes also used to describe these 22-carbon structures.
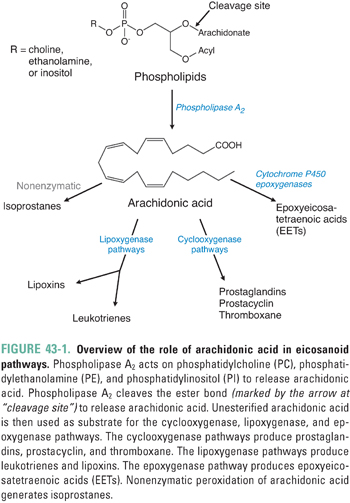
Generation of Arachidonic Acid and Omega-3 Fatty Acids
Arachidonic acid (all-cis-5,8,11,14-eicosatetraenoic acid) is the common precursor to the vast majority of eicosanoids (Fig. 43-1). Arachidonic acid must be biosynthesized from the essential fatty acid precursor linoleic acid (all-cis-9,12-octadecadienoic acid), which humans can obtain only from dietary sources. Eicosapentaenoic acid (all-cis-5,8,11,14,17-eicosapentaenoic acid; EPA) and docosahexaenoic acid (all-cis-4,7,10,13,16,19-docosahexaenoic acid; DHA) are the precursors to resolvins, protectins, and maresins. Humans can obtain EPA and DHA either from dietary sources or from biotransformation of the essential fatty acid precursor α-linolenic acid (all-cis-9,12,15-octadecatrienoic acid). α-Linolenic acid, EPA, and DHA are also called omega-3 fatty acids because they contain a double bond between the third and fourth carbons from the terminal (ω) end of the molecule.
Within cells, arachidonic acid does not exist as a free fatty acid but rather is esterified to the sn2 position of membrane phospholipids, predominantly phosphatidylcholine and phosphatidylethanolamine. Arachidonic acid is released from cellular phospholipids by the enzyme phospholipase A2 (Fig. 43-1), which hydrolyzes the acyl ester bond. This important reaction, which represents the first step in the arachidonic acid cascade, is the overall rate-determining step in the generation of eicosanoids.
There are membrane-bound and soluble isoforms of phospholipase A2, classified as secretory (sPLA2) and cytoplasmic (cPLA2), respectively. The numerous phospholipase A2 isoforms are differentiated based on molecular weight, pH sensitivity, regulation and inhibition characteristics, calcium requirements, and substrate specificity. The existence of multiple isoforms allows for calibrated regulation of the enzyme in different tissues to achieve selective biological responses. Phospholipase A2 isoforms relevant to inflammation are stimulated by cytokines (such as TNF-α, GM-CSF, and IFN-γ) and growth factors (such as epidermal growth factor [EGF] and the MAP kinase–protein kinase C [MAPK–PKC] cascade). Although glucocorticoids were once thought to inhibit phospholipase A2 directly, it has now been shown that this action is mediated by inducing the synthesis of lipocortins, a family of phospholipase A2-regulatory proteins. One of the lipocortins, annexin 1, mediates some of the anti-inflammatory actions of glucocorticoids (see below).
Unesterified intracellular arachidonic acid is converted by cyclooxygenase, lipoxygenase, or the cytochrome-containing epoxygenase enzymes; the specific enzyme dictates the particular class of local eicosanoids that are generated. The cyclooxygenase pathway leads to the formation of prostaglandins, prostacyclin, and thromboxanes; the lipoxygenase pathways lead to leukotrienes and lipoxins; and the epoxygenase pathways lead to epoxyeicosatetraenoic acids (Fig. 43-1).
Cyclooxygenases (also known as prostaglandin H synthases) are glycosylated, homodimeric, membrane-bound, heme-containing enzymes that are ubiquitous in animal cells from invertebrates to humans. Two cyclooxygenase isoforms, denoted COX-1 and COX-2, are found in humans. Although COX-1 and COX-2 share ~60% sequence homology and near-superimposable three-dimensional structures, the genes are located on different chromosomes, and the enzymes differ in cellular, genetic, physiologic, pathologic, and pharmacologic profiles (Tables 43-1 and 43-2). Each cyclooxygenase enzyme catalyzes two sequential reactions. The first reaction, the cyclooxygenase step, is the oxygen-dependent cyclization of arachidonic acid to prostaglandin G2 (PGG2); the second reaction, the peroxidase step, is the reduction of PGG2 to PGH2 (Fig. 43-2).
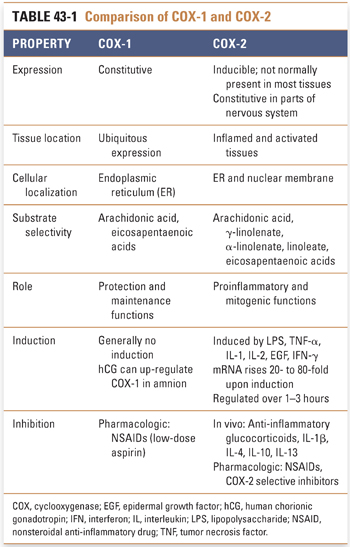
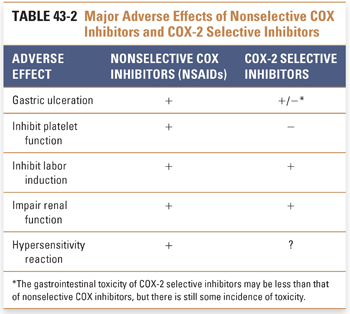
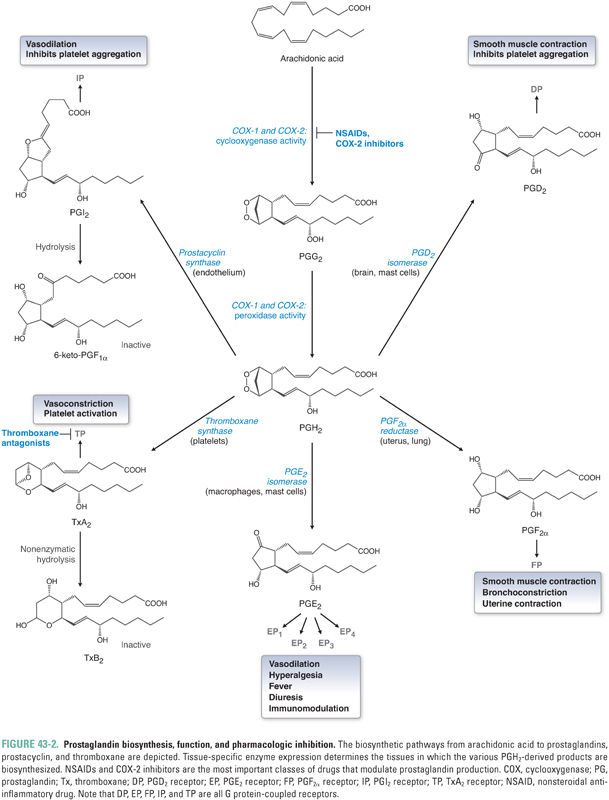
As a result of differences in cellular localization, regulatory profile, tissue expression, and substrate requirements, COX-1 and COX-2 ultimately produce different sets of eicosanoid products that are involved in different pathways and functions. Constitutively expressed COX-1 is believed to function in physiologic, or “housekeeping,” activities such as vascular homeostasis, maintenance of renal and gastrointestinal blood flow, renal function, intestinal mucosal proliferation, platelet function, and antithrombogenesis. A number of “as-needed,” or specialized, functions are attributed to the products of the inducible COX-2 enzyme, including roles in inflammation, fever, pain, transduction of painful stimuli in the spinal cord, mitogenesis (particularly in the gastrointestinal epithelium), renal adaptation to stresses, deposition of trabecular bone, ovulation, placentation, and uterine contractions of labor. The role of constitutive COX-2 expression in areas of the nervous system, such as the hippocampus, hypothalamus, and amygdala, remains to be elucidated.
Protein kinetic studies suggest that there may be a third functional cyclooxygenase isoform. The putative COX-3 isoform may be a product of the same gene as COX-1 but with different protein characteristics, possibly because of alternative mRNA splicing or post-translational modification.
Prostaglandins represent a large family of structurally similar compounds that have potent and specific biological actions. The name of the family derives from their initial identification in the genitourinary system of male sheep. Prostaglandins all share a chemical structure, called a prostanoid, consisting of a 20-carbon carboxylic acid characterized by a cyclopentane ring and a 15-hydroxyl group (Fig. 43-3).
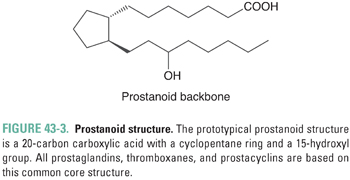
Prostaglandins are divided into three major subseries: PG1, PG2, and PG3. The subscript numeral indicates the number of double bonds present in the molecule. The PG2 series is the most biologically prevalent in humans because these are the direct derivatives of arachidonic acid, which is an eicosatetraenoic acid (C20:4, with the notation indicating the number of carbon atoms and the number of double bonds in the fatty acid). The PG1 series derive from the arachidonic acid precursor dihomo-γ-linolenic acid (DHGLA), an eicosatrienoic acid, while the PG3 series derive from an eicosapentaenoic acid (EPA, C20:5). (As alluded to earlier, protectins, D-series resolvins, and maresins derive from docosahexaenoic acid, DHA, C22:6.) Prostaglandin PGH2 represents the critical juncture downstream of cyclooxygenase (Fig. 43-2), because it is the immediate precursor to PGD2, PGE2, PGF2α, thromboxane A2 (TxA2), and prostacyclin (PGI2). The distribution of these eicosanoids in various tissues is determined by the expression pattern of the different downstream enzymes of prostaglandin synthesis (i.e., PG synthases) (Fig. 43-2).
The prostaglandins are important in many physiologic processes, many of which are not directly related to inflammation. These functions are highlighted in Table 43-3. Note the especially important housekeeping functions of PGE2, broadly referred to as cytoprotective roles, in which organs such as gastric mucosa and renal parenchyma are shielded from the effects of ischemia by PGE2-mediated vasodilation and overall regulation of blood flow. PGE2 is also involved in inflammatory cell activation, and PGE2 that is biosynthesized by COX-2 and PGE2 synthase in cells near the hypothalamus appears to have a role in fever.
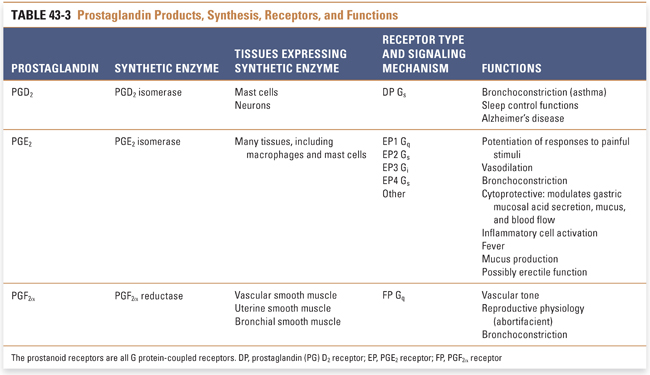
Platelets express high levels of the enzyme thromboxane synthase but do not contain prostacyclin synthase. Therefore, TxA2 is the chief eicosanoid product of platelets. TxA2 has a half-life of only 10–20 seconds before it is nonenzymatically hydrolyzed to inactive TxB2. TxA2, which signals via a seven transmembrane G protein-coupled receptor (GPCR) Gq mechanism, is a potent vasoconstrictor as well as a promoter of platelet adhesion and aggregation. In contrast, the vascular endothelium lacks thromboxane synthase but expresses prostacyclin synthase. Therefore, PGI2 is the primary eicosanoid product of the vascular endothelium. PGI2, which signals via Gs, functions as a vasodilator, venodilator, and inhibitor of platelet aggregation. In other words, PGI2 is the physiologic antagonist of TxA2. The vasodilatory actions of PGI2, like those of PGE2, also confer cytoprotective properties.
The local balance between TxA2 and PGI2 levels contributes to regulation of arterial resistance (and thus blood pressure) and to thrombogenesis. Imbalances can lead to hypertension, ischemia, thrombosis, coagulopathy, myocardial infarction, and stroke. In certain populations of the northern latitudes (including Inuit, Greenland, Irish, and Danish populations), the incidence of heart disease, stroke, and thromboembolic disorders is less than in other populations. The diet of these northern peoples is richer in fish oils and, as a result, contains relatively larger amounts of marine oils (including EPA and DHA). Analogous to the conversion of arachidonic acid into TxA2 and PGI2, EPA is converted into TxA3 and PGI3. Importantly, the vasoconstricting and platelet aggregating effects of TxA3 are relatively weak. As a result, the thromboxane–prostacyclin balance could be tipped toward vasodilation, platelet inhibition, and antithrombogenesis. This is one possible contributor to the observation that these northern populations have a lower incidence of heart disease and is cited as one rationale for increasing dietary fish consumption. Novel marine oil-derived mediators that possess potent anti-inflammatory and pro-resolving actions have also recently been discovered (see “Lipoxins, Resolvins, Protectins, and Maresins” section).
Besides the cyclooxygenase pathway, the other major metabolic fate of arachidonic acid is the lipoxygenase pathway, which leads to the formation of leukotrienes and lipoxins. Lipoxygenases are enzymes that catalyze the insertion of molecular oxygen into arachidonic acid, using non-heme iron to generate specific hydroperoxides. Three lipoxygenases, 5-lipoxygenase, 12-lipoxygenase, and 15-lipoxygenase (5-LOX, etc.), are the major LOX isoforms found in humans (Table 43-4). The lipoxygenases are numbered based on the carbon position in arachidonic acid on which they catalyze the insertion of molecular oxygen. The immediate products of lipoxygenase reactions are hydroperoxyeicosatetraenoic acids (HPETEs). HPETEs can be reduced to the corresponding hydroxyeicosatetraenoic acids (HETEs) by glutathione peroxidase-dependent enzymes. 5-HPETE formed by 5-LOX is the direct precursor to leukotriene A4 (LTA4), which itself is the precursor to all bioactive leukotrienes (Fig. 43-4). Lipoxygenases are also involved in converting 15-HETE and LTA4 to lipoxins (Fig. 43-5).
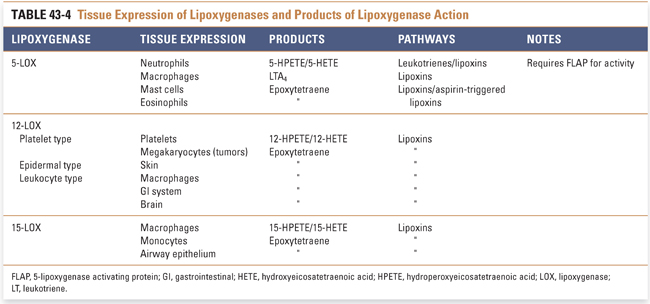
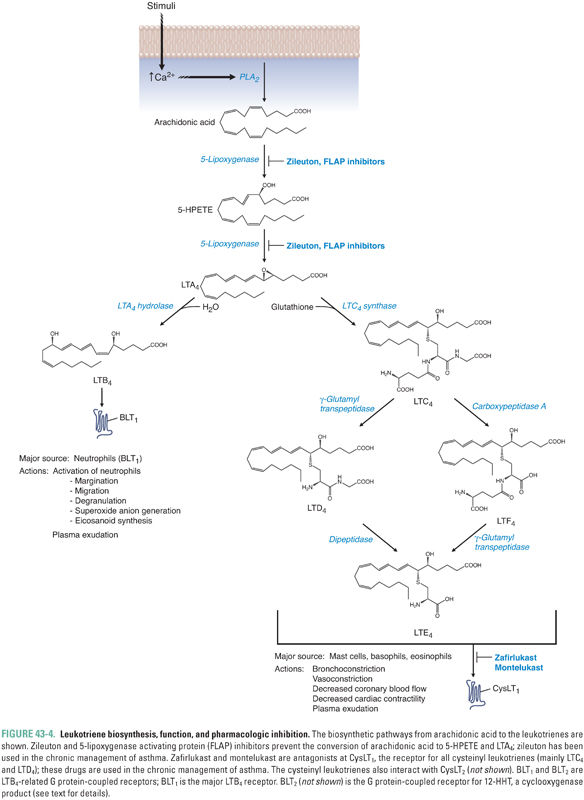
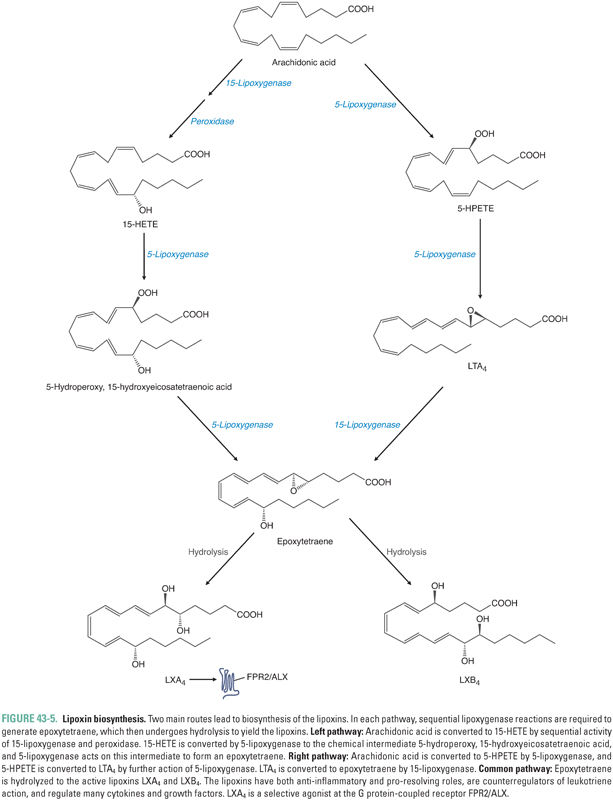
5-LOX requires translocation to the nuclear membrane for enzymatic activity. The protein 5-lipoxygenase-activating protein (FLAP) helps 5-LOX translocate to the nuclear membrane, form an active enzyme complex, and accept the arachidonic acid substrate from phospholipase A2.
Leukotriene biosynthesis begins with the 5-LOX-mediated conversion of 5-HPETE to leukotriene A4 (LTA4). Therefore, 5-LOX catalyzes the first two steps in leukotriene biosynthesis (Fig. 43-4). It is not known whether 5-HPETE diffuses out of the 5-LOX catalytic site between these steps or remains bound to the same 5-LOX enzyme for both reactions.
LTA4 is next converted to either LTB4 or LTC4. The enzyme LTA4 hydrolase converts LTA4 to LTB4 in neutrophils and erythrocytes. The conversion of LTA4 to LTC4 occurs in mast cells, basophils, eosinophils, and macrophages by the addition of a γ-glutamylcysteinylglycine tripeptide (glutathione). LTC4, LTD4, LTE4, and LTF4, which represent the cysteinyl leukotrienes, are interconverted by removal of amino acid portions of the γ-glutamylcysteinylglycine tripeptide (Fig. 43-4).
LTB4 acts via two G protein-coupled receptors, BLT1 and BLT2. Binding of LTB4 to BLT1, which is expressed mainly in tissues involved in host defense and inflammation (leukocytes, thymus, spleen), leads to proinflammatory sequelae such as neutrophil chemotaxis, aggregation, and transmigration across epithelium and endothelium. LTB4 up-regulates neutrophil lysosomal function and generates reactive oxygen species (ROS), enhances cytokine production, and potentiates the actions of natural killer (NK) cells. BLT2 has been found to bind the COX product 12-HHT (12-hydroxy-5,8,10-heptadecatrienoic acid) and evoke chemotaxis of leukocytes and may play a role in tumorigenesis.
The cysteinyl leukotrienes (LTC4 and LTD4) bind to CysLT1 receptors to cause vasoconstriction, bronchospasm, and increased vascular permeability. Cysteinyl leukotrienes are responsible for the hyperreactivity to stimuli and the airway and vascular smooth muscle contraction that occur in asthmatic, allergic, and hypersensitivity processes. Together, both arms of the leukotriene pathways (i.e., LTB4 and LTC4/LTD4) play key roles in psoriasis, asthma, arthritis, and various inflammatory responses. They are also key mediators in vascular disease and are likely to be important in atherosclerosis and obesity.
Lipoxins, Resolvins, Protectins, and Maresins
Lipoxins (lipoxygenase interaction products) are derivatives of arachidonic acid containing four conjugated double bonds and three hydroxyl groups. The two main lipoxins, LXA4 and LXB4 (Fig. 43-5), balance the proinflammatory actions of leukotrienes and cytokines and are thus important in coordinating resolution of inflammation.
At sites of inflammation, there is typically an inverse relationship between the amounts of lipoxin and leukotriene present. This observation has led to the suggestion that lipoxins may act as counterregulatory signals or negative regulators of leukotriene action. LXA4 receptors are present on neutrophils and in the lung, spleen, and blood vessels. Lipoxins blunt neutrophil chemotaxis, adhesion, and transmigration through endothelium (by decreasing P-selectin expression), limit eosinophil recruitment, stimulate vasodilation (by inducing synthesis of PGI2 and PGE2), inhibit LTC4– and LTD4-stimulated vasoconstriction, inhibit LTB4 inflammatory effects, and inhibit the function of NK cells. Lipoxins stimulate the uptake and clearance of apoptotic neutrophils by macrophages and thereby mediate resolution of the inflammatory response. Because lipoxin production appears to be important in the resolution of inflammation, an imbalance in lipoxin–leukotriene homeostasis may be a key factor in the pathogenesis of inflammatory disease. For example, it is possible that Ms. G’s chronic joint inflammation involves an imbalance in the relative amounts of leukotrienes and lipoxins in her affected joints.
Metabolomic approaches have identified endogenous families of omega-3-derived mediators, called resolvins, protectins, and maresins, that control both the magnitude and duration of inflammation (Fig. 43-6). Together with lipoxins, they constitute a chemical genus of specialized pro-resolving mediators (SPM). Resolvins, protectins, and maresins are biosynthesized from essential omega-3 fatty acid precursors, especially EPA and DHA.
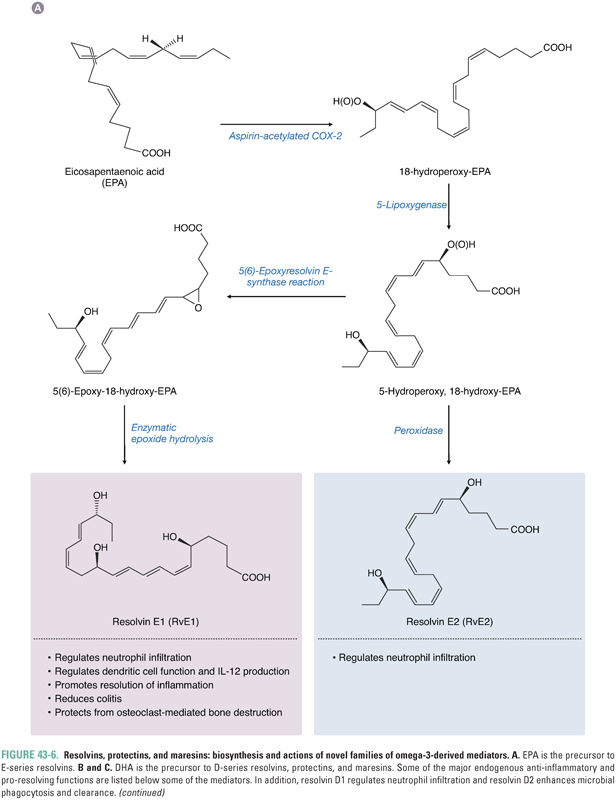
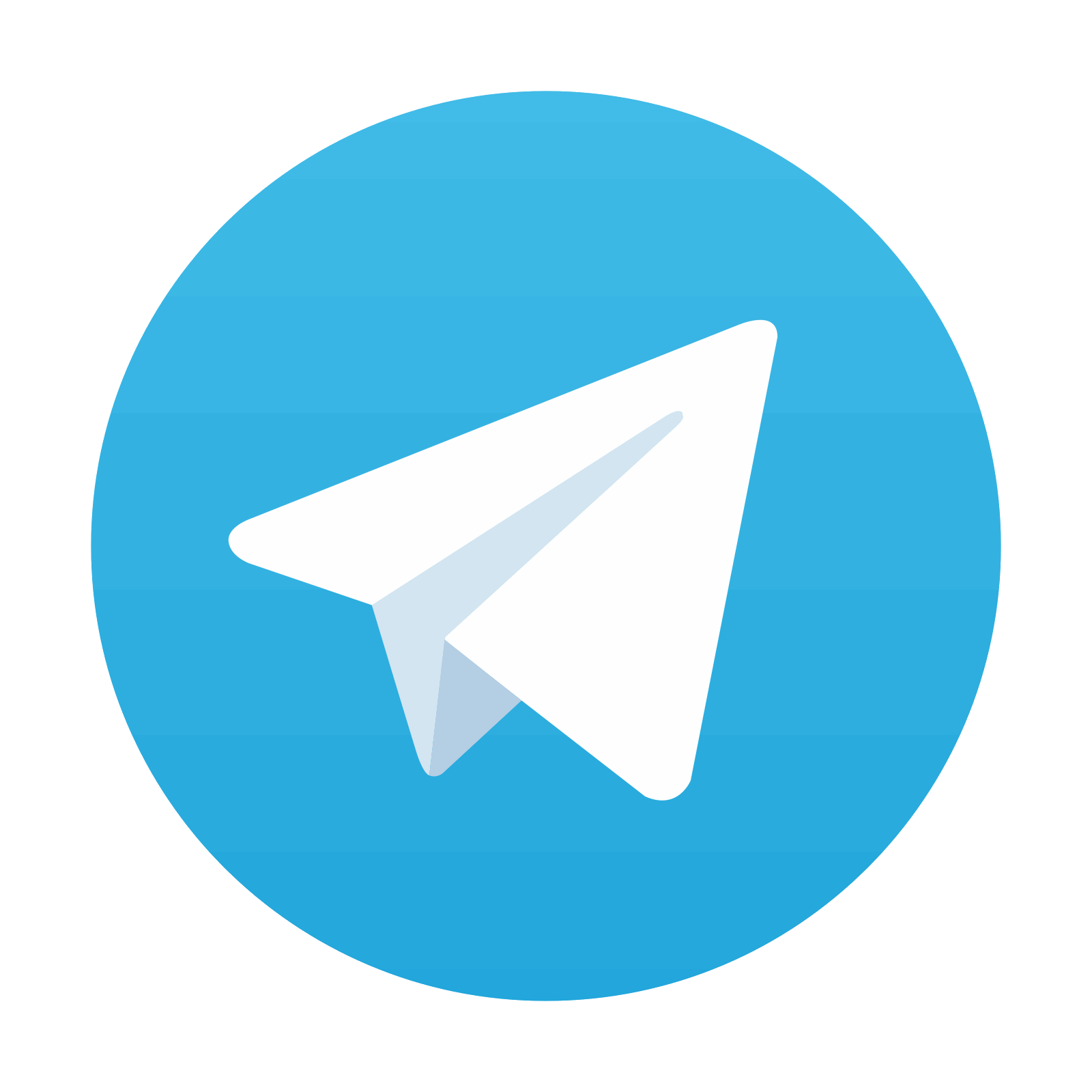
Stay updated, free articles. Join our Telegram channel

Full access? Get Clinical Tree
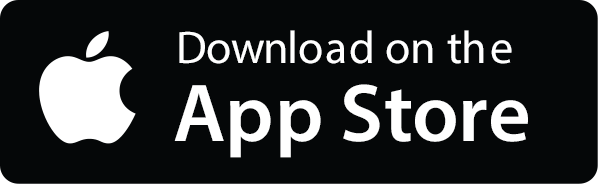
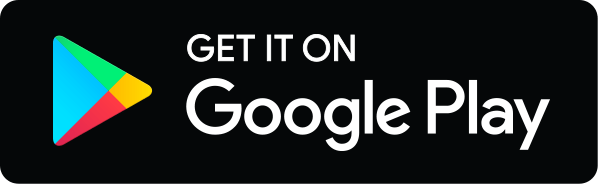