16 Parasite survival strategies and persistent infections
Strategies to evade natural non-adaptive defences such as the phagocyte
• Killing or avoiding being killed by phagocytes. Successful parasites have evolved numerous ingenious antiphagocytic devices. Antiphagocytic devices (Fig. 16.1) range from killing or inhibiting the phagocyte itself, via more subtle ways of eluding contact, to protection against intracellular death allowing the microorganism to survive within the phagocyte – a very serious challenge to the host.
• Interfering with ciliary action (see Fig. 13.3).
• Interfering with the activation of complement. Microorganisms can acquire or mimic complement regulators, actively inhibit complement components, or enzymatically destroy complement components. A variety of microbes can bind complement regulators, including E. coli, streptococci and Candida albicans. The smallpox and vaccinia viruses produce proteins that mimic host complement regulators. Staph. aureus, streptococci, herpes simplex virus, Schistosoma and Trypanosoma express complement inhibitors. Proteases that destroy complement components are produced by Pseudomonas, Serratia marcescans and Schistosoma mansoni. Other strategies are to physically block complement lysis – the insertion of the C567 complex is prevented by the long side chains of the cell wall polysaccharides of smooth strains of Salmonellae and by the capsules of staphylococci, which do not activate complement, and the cell wall of Gram-positive bacteria prevents lysis by the complement membrane attack complex (Fig. 16.2). However, some pathogens take the opposite approach, choosing to enter host cells by exploiting opsonization with complement components – HIV-1 and Mycobacterium tuberculosis exploit the CR3 receptor in this way.
• Producing iron-binding molecules. Nearly all bacteria need iron, but the host’s iron-binding proteins such as transferrin limit the availability of this element. Accordingly, certain bacteria (e.g. Neisseria) produce their own powerful iron-binding proteins to circumvent the shortage
• Blocking interferons. Host cells respond to double-stranded DNA (dsRNA) from infecting microbes (including all viruses), by forming interferons alpha and beta. These are produced rapidly, within 24–h, after infection and are part of the non-adaptive response. Certain viruses are either poor inducers of interferons (hepatitis B) or produce molecules that block the action of interferons in cells (hepatitis B, HIV, adenoviruses, Epstein–Barr virus, vaccinia virus). Interferon gamma (IFNγ), an essential part of the adaptive response, is also affected.
Strategies to evade adaptive defences
Strategies to evade adaptive defences are more sophisticated than those for evading innate defences
• The polysaccharide capsules of bacteria prevent non-immune contact between phagocytes and the bacterial cell wall, but are quickly recognized as foreign by B-cell surface receptors (immunoglobulin), leading to the formation of antibody with consequent opsonization and phagocytosis of the bacteria
• Many microorganisms such as bacteria and fungi can resist intracellular destruction by macrophages, but their peptides are presented in association with major histocompatibility complex (MHC) molecules on the macrophage surface, and their presence is detected by T cells. A new set of cytotoxic and other immune mechanisms is then brought into action.
Parasite survival strategies
Parasite survival strategies can take as many forms as there are parasites, but they can be usefully classified according to the immune component that is evaded and the means selected to do this (see Ch. 12). As a result, the microbe is able to undergo what are often quite lengthy periods of growth and spread during the incubation period before being shed and transmitted to the next host, as occurs in hepatitis B and tuberculosis. Shedding of the microbe for just a few extra days after clinical recovery gives more extensive transmission in the community, and this is a worthwhile result for the microbe.
Viruses are particularly good at thwarting immune defences
Viruses are able to thwart immune defences for a number of reasons:
• Their invasion of tissues and cells is often ‘silent’. Unlike most bacteria, they do not form toxins, and as long as they do not cause extensive cell destruction there is no sign of illness until the onset of immune and inflammatory responses, sometimes several weeks after infection, as occurs in hepatitis B virus and EBV infections.
• Viruses such as rubella virus, wart viruses, hepatitis B virus and EBV can infect cells for long periods without adverse effects on cell viability.
Some microbes are able to persist in the host
• those that are shed more or less continuously, such as the Epstein–Barr virus (EBV) into saliva, hepatitis B virus into blood, and eggs into faeces in various helminth infections
• those that are shed intermittently, such as herpes simplex virus (HSV), polyomaviruses, typhoid bacilli, tubercle bacilli and malaria parasites.
Virus latency is a type of persistence and is based on an intimate molecular relationship with the infected cell. The viral genome continues to be present in the host without producing antigens or infectious material, and only does so very occasionally, when the virus reactivates (becomes patent) (Box 16.1).
Box 16.1 Lessons in Microbiology
Trail of illness from a slippery cook
Mary had recovered fully from an attack of typhoid earlier in life, but she had gallstones and this enabled the bacteria to persist in her gallbladder for many years, appearing intermittently in the faeces. About 5% of cases become carriers, either in gallbladder or urinary bladder, and they play a central role as foci of infection. Nowadays, Mary would have to have acquired her original infection in a region such as the Indian subcontinent where typhoid is endemic. Each year, there are up to 22 million typhoid cases worldwide, 300 of which are in the USA, most being travellers to the Indian subcontinent (see also Ch. 22).
Strategies for evading host defences cause a rapid ‘hit-and-run’ infection
Colonizing privileged sites keeps the microbe out of reach of circulating lymphocytes
The vast numbers of microbes that colonize the skin and the intestinal lumen, together with those that are shed directly into external secretions, are effectively out of reach of circulating lymphocytes. They are exposed to secretory antibodies, which although able to bind to the microbe (e.g. influenza virus) and render it less infectious, are generally unable to kill the microbe or control its replication in or on the epithelial surface (Figs 16.3, 16.4). A local inflammatory response, however, can enhance host defences.
Additional privileged sites can be created by the infectious organism itself. A good example is the hydatid cyst that develops in liver, lung or brain around growing colonies of the tapeworm Echinococcus granulosus (Fig. 16.5), inside which the worms can survive even though the blood of the host contains protective levels of antibody.
Perhaps the most highly privileged site of all is host DNA, and this is occupied by the retroviruses. Retroviral RNA is transcribed by the reverse transcriptase into DNA as a necessary part of the replicative cycle, and this then becomes integrated into the DNA of the host cell (see Ch. 21). Once integrated, and as long as there is no cell damage and viral products are not expressed on the cell surface where they can be recognized by immune defences, the virus enjoys total anonymity. This is what makes complete cure and complete removal of virus from a patient infected with HIV such a daunting task. The intragenomic site becomes even more privileged if the egg or sperm is infected. The viral genome will then be present in all embryonic cells and transferred from one generation to another as if it were the host’s own DNA. Luckily, this does not happen with HIV or with human T-cell lymphotropic virus (HTLV) 1 and 2. However, the ‘endogenous’ retroviruses of humans present in profusion as DNA sequences in our genome, but not expressed as antigens, come into this category. They are part of our inheritance. This surely represents the ultimate, the final logical step in parasitism, at the borderline between infection and heredity.
Mimicry sounds like a useful strategy, but does not prevent the host from making an antimicrobial response
If the microbe can in some way avoid inducing an immune response, this can be regarded as a ‘concealment’ of its antigens. One method is by mimicking host antigens, as such self antigens are not recognized as foreign. Numerous examples are known of parasite-derived molecules that resemble those of the host (Table 16.1). In the case of viral proteins, mimicry based on amino acid sequence homology (sharing of 8–10 consecutive amino acids) is seen to be common when computer comparisons are made between viral and host proteins. Perhaps the most celebrated example, however, is the cross-reaction between group A beta-haemolytic streptococci and human myocardium. This cross-reaction underlies the development of rheumatic heart disease, following repeated streptococcal infection because of antibody made against the cross-reacting determinant meromyosin (Fig. 16.6). The fact that the host makes such autoantibodies shows that in this case mimicry does not protect the bacteria. The conclusion is that, although mimicry sounds like a useful strategy for microbes and occurs quite frequently, it is probably an accident rather than a sinister microbial strategy. It does not prevent the host from making an antimicrobial and autoimmune response.
Table 16.1 Some examples of mimicry or uptake of host antigens by parasites
Microbe’s strategy | Parasite | Corresponding host antigen |
---|---|---|
Mimicry | Epstein–Barr virus | Human fetal thymusa |
Streptococci | Cardiac muscle (meromyosin) | |
Mycobacterium tuberculosis | 65 kDa heat shock protein | |
Neisseria meningitidis | Embryonic brain, vitronectin | |
Treponema | Cardiolipinb | |
Mycoplasma pneumoniae | Erythrocytesc | |
Plasmodium falciparum | Spondind | |
Trypanosoma cruzi | Heart myosin, nerve | |
Schistosoma | Glutathione transferase | |
Antigen uptake | Neisseria meningitides | Vitronectin |
Haemophilus influenzae | Vitronectin | |
Cytomegalovirus | β2-microglobulin | |
Schistosoma | Glycolipids, HLA I, HLA II | |
Filarial nematodes | Albumin | |
Ascaris | Blood group antigens |
a Also cross-reacts with erythrocytes of certain species and is the basis for the Paul Bunnell (heterophil antibody) test.
b Basis for Wasserman-type antibody test for syphilis.
c Basis for cold agglutinin test, but may result from damage to erythrocytes rather than direct mimicry.
d The homologous malaria protein thrombospondin-related anonymous protein (TRAP) shares sequence with the circumsporozoite protein that mediates binding to hepatocytes. HLA, human leukocyte antigen; Ig, immunoglobulin.
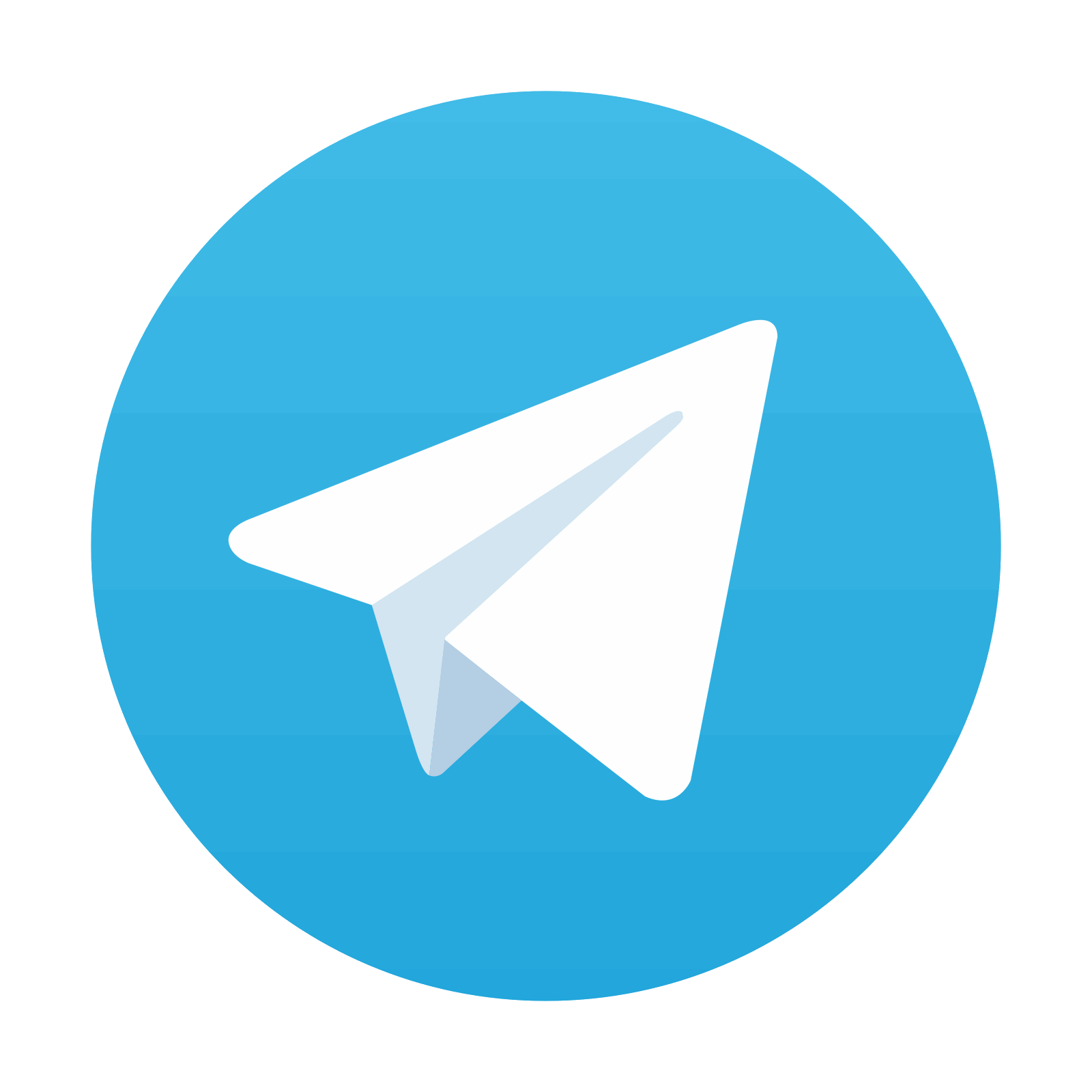
Stay updated, free articles. Join our Telegram channel

Full access? Get Clinical Tree
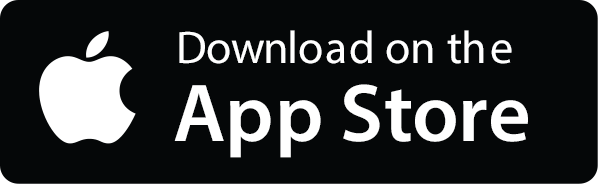
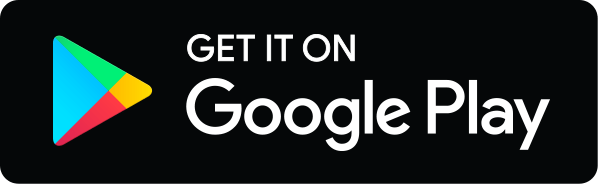