Pain
Joni D. Marsh
Key Questions
• How is neurotransmission of pain signals modulated at the receptor, spinal cord, and brain?
• How do acute pain and chronic pain differ with regard to cause and clinical manifestations?
• Why are some painful sensations perceived at a distance from the site of injury (referred)?
http://evolve.elsevier.com/Copstead/
Pain is a complex physiologic and perceptual phenomenon. Because pain is very much a subjective experience, defining and assessing it are difficult. Merskey defined pain as “an unpleasant sensory and emotional experience associated with actual or potential tissue damage or described in terms of such damage.”1 McCaffery offered a clinically useful definition: “Pain is whatever the experiencing person says it is, existing whenever the experiencing person says it does.”2 Accurate assessment and optimal management of pain are extremely important not only because relief of pain and suffering is ethically desirable but also because unrelieved pain is physiologically harmful. Studies have documented the benefits of adequate pain control on the rate of recovery, health care costs, and postoperative morbidity. In fact, The Joint Commission (TJC) has developed standards of care regarding the assessment and management of pain that must be followed by all accredited agencies. Pain has been referred to as the fifth vital sign.
Physiology of Pain
The physiologic mechanisms involved in the pain phenomenon are termed nociception. Nociception can be divided into four stages: transduction, transmission, perception, and modulation. Transduction is the process of converting painful stimuli to neuronal action potentials at the sensory receptor. Transmission refers to the movement of action potentials along neurons that make their way from the peripheral receptor to the spinal cord and then centrally to the brain. Perception occurs when the brain receives pain signals and interprets them as painful. The complex mechanism whereby synaptic transmission of pain signals is altered is called modulation. It is clinically useful to conceptualize pain physiology according to these four processes because each stage provides an opportunity for intervention in the pain experience (Figure 47-1).
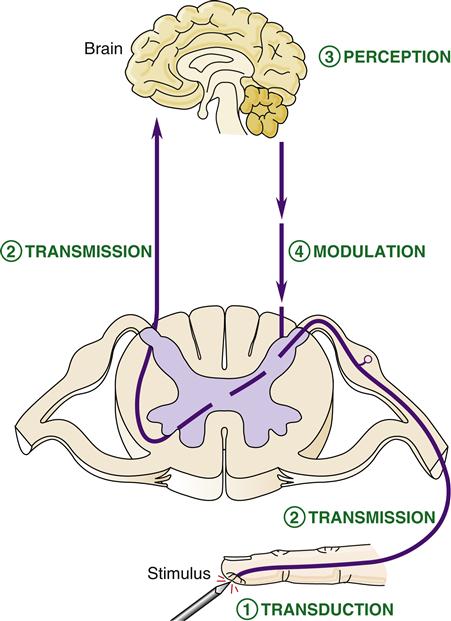
Transduction
Most pain begins in the periphery when free nerve endings called nociceptors are stimulated. Nociceptors transduce noxious stimuli into neuronal action potentials that progress centrally to the spinal cord and then the brain. Nociceptors are found in skin; muscle; connective tissue; the circulatory system; and the abdominal, pelvic, and thoracic viscera. Stimulation can be the result of direct damage to nerve endings, or it can result from release of chemicals at the site of injury.
Numerous substances participate in the initiation of nociceptive impulses. Some of these substances are released as a direct result of tissue injury, whereas others may be produced as part of the inflammatory response to the injury. Important chemical mediators of pain include K+, H+, lactate, histamine, serotonin, bradykinins, and prostaglandins.3 These chemicals alter the membrane potential of the pain receptor, and if depolarization is sufficient, action potentials are generated. When these impulses are conducted centrally, the second step (transmission) is initiated.
Prostaglandin involvement in the process of nociceptor stimulation is of particular interest because prostaglandin inhibitors such as aspirin and other nonsteroidal antiinflammatory drugs (NSAIDs) are commonly used to manage pain. Prostaglandins are formed when cells are damaged and an enzyme, phospholipase A, breaks down phospholipids in the cell membrane and converts them to arachidonic acid4 (Figure 47-2). Arachidonic acid undergoes further breakdown by the enzyme cyclooxygenase to form prostaglandins. Sensitization by prostaglandins lowers the threshold of nociceptive fibers so that stimuli that would not cause pain under normal circumstances are now pain producing. NSAIDs prevent prostaglandin production by inhibiting the action of cyclooxygenase.
Transmission
Stimulated nociceptors transmit impulses to the central nervous system (CNS) by means of specialized sensory fibers. The primary sensory fibers involved in the transmission of nociceptive impulses are the Aδ and C fibers.3 The characteristics and functions of these fibers are summarized in Table 47-1. In general, the larger, myelinated Aδ fibers transmit the nociceptive impulses very quickly as an initial response to tissue injury. The nature of the pain carried by the fast-traveling Aδ fibers is characterized as sharp, stinging, and highly localized. In contrast, unmyelinated C fibers transmit pain more slowly. Pain transmitted by C fibers is poorly localized and has a dull or aching quality that lingers long after the initial sharp pain abates. The majority of pain sensations travel via C fibers and project to areas of the brain that evoke emotional responses such as displeasure and anxiety.
TABLE 47-1
FEATURE | Aδ FIBERS | C FIBERS |
Structure | Myelinated | Unmyelinated |
Amount | 10% | 90% |
Source | Thermal, mechanical stimuli | Polymodal stimuli (mechanical, thermal, chemical) |
Speed | Fast traveling, 5-10 m/sec | Slower traveling, 0.6-2 m/sec |
Sensory quality of pain mediated | Sharp, stinging, cutting, pinching | Dull, burning, aching |
Most sensory afferent pain fibers enter the spinal cord by way of the posterior nerve roots (Figure 47-3). The cell bodies of pain neurons are located in the dorsal root ganglion. As the afferent neurons enter the dorsal horn, collateral branches spread up and down the spinal cord for two to three segments by way of the tract of Lissauer. These spinal connections are important for reflex postural adjustments when a painful body part is suddenly withdrawn from the painful stimulus.
Sensory afferent neurons synapse with interneurons, anterior motor neurons, and sympathetic preganglionic neurons in specific regions of the spinal cord (see Figure 47-3). Aδ fibers and C fibers carry excitatory impulses from cutaneous pain receptors in small, localized areas of the skin to interneurons in lamina I. Many of the neurons originating in lamina I cross the spinal cord to activate neurons in the anterolateral tract.
Laminae II and III represent a key anatomic region of the cord involved in pain transmission known as the substantia gelatinosa. The substantia gelatinosa is characterized by multiple synaptic connections among primary sensory afferent neurons, interneurons, and anterolateral ascending fibers. There is much opportunity at this point for pain signal transmission to be modulated by other sensory input or from CNS activity. Pain signals can be either enhanced or blocked at these synapses.
Another key synaptic area involved in nociception is lamina V. Numerous Aδ and C fibers deliver somatic input from mechanical, thermal, and chemical receptors in the periphery to lamina V. Sensory afferent neurons from visceral receptors also terminate in lamina V. The convergence of both somatic and visceral fibers in lamina V may help explain the phenomenon of referred pain, in which pain from a visceral organ is perceived at the body surface.3,5 The remaining, deeper laminae VI to VIII receive sensory input from muscles, joints, and visceral afferent fibers.
A number of neurotransmitters and neuropeptides are involved in synaptic transmission in the spinal cord. Substance P is a well-known example. Others include excitatory amino acids (glutamate), γ-aminobutyric acid (GABA), cholecystokinin, and calcitonin gene–related peptide. These neurotransmitters bind to the next neurons in the pathway and thereby initiate action potentials. The pain signal is propelled along its pathway toward the brain. Interruption of these synaptic processes can inhibit pain transmission. The synapses in the spinal cord are extremely important points of pain modulation by both endogenous and exogenous means.
The excitatory neurotransmitter glutamate is involved in carrying the nociceptive message from primary afferent fibers to secondary neurons. Glutamate binding to its N-methyl-D-aspartate (NMDA) receptors on the postsynaptic neuron is thought to induce a kind of synaptic memory in the pain pathway. Excessive or repeated stimulation of C fibers sensitizes the spinal cord neurons so that even mild stimulation may be perceived as painful.5,6 This phenomenon has been termed “wind-up” and may be an important mechanism in the development of chronic pain syndromes. Drugs that inhibit glutamate production may impede the wind-up response, thereby controlling pain before synaptic memory of the pain develops in the pain pathways.
Pain signals transmitted by the spinal interneurons are then conducted to the brain by ascending spinal pathways (Figure 47-4). The major pathway for pain signal transmission up the spinal cord is the anterolateral tract, so named because it travels in the anterolateral portion of the white matter of the spinal column. This tract is also called the spinothalamic tract in some texts and has two divisions: the neospinothalamic tract and the paleospinothalamic tract. Both divisions cross at the spinal segment and carry pain signals up the contralateral (opposite) side of the cord. Thus nociceptor input from the right side of the body travels in the anterolateral tracts on the left side of the cord, whereas pain signals from the left side of the body travel on the right side of the cord.
The neospinothalamic division has fewer synapses in the cord and projects first to the thalamus and then to the primary somatosensory cortex. Aδ fiber signals are transmitted in this tract and reach the brain quickly to provide specific information about pain location with little emotional connotation. C fiber impulses travel mainly in the paleospinothalamic division, which makes a greater number of synapses and reaches the brain more slowly. The paleospinothalamic tract projects to widespread brain areas and stirs aversive emotional responses. The paleospinothalamic tract travels with the neospinothalamic tract in the anterolateral portion of the spinal cord to the level of the medulla and then sends diffuse projections to the reticular formation, the mesencephalon, and, finally, the thalamus. From the thalamus, further projections to the cerebral cortex, limbic system, and basal ganglia occur. The pain sensation from C fibers is poorly localized, longer lasting, and more distressing than Aδ fiber pain. Pain impulses entering the brainstem reticular activating formation, thalamus, and other lower brain centers cause conscious perception of pain, but the cortex is important in interpreting pain quality.3
The brain can localize a pain sensation to a particular part of the body because nociceptor pathways are kept in specific anatomic order in the cord and somatosensory cortex. Each spinal nerve contains the nociceptor fibers for a particular area of the body surface, called a sensory dermatome (Figure 47-5). Dermatomal maps are useful for locating a source of neurologic pain. Pain that follows a dermatomal distribution is due to spinal nerve compression or trauma and is called a radiculopathy. Vertebral disk disease is a common cause of radiculopathy. Peripheral neuropathies, in contrast, do not follow a dermatomal pattern. Examples of peripheral neuropathies are carpal tunnel syndrome (median nerve) and diabetic neuropathy, which often affects both legs in a stocking-like pattern.
Perception
Perception is the result of neural processing of pain sensations in the brain. Perception includes an awareness and interpretation of the meaning of the sensation. Pain perception is influenced by attention, distraction, anxiety, fear, fatigue, and previous experience and expectations. Pain perception is not localized to a specific brain area.3 Complete removal of the somatic sensory areas of the cerebral cortex does not destroy an animal’s ability to perceive pain.3 Numerous neuronal networks are necessary to localize, process, and interpret painful sensations. The primary somatosensory cortex, association cortex, frontal lobe, and limbic structures all participate in this processing.
Pain perception can be described in terms of pain threshold and pain tolerance. Pain threshold is the level of painful stimulation required to be perceived and is remarkably similar from one individual to another. Pain tolerance is the degree of pain that one is willing to bear before seeking relief. Pain tolerance varies widely among individuals and within the same individual under differing conditions. Age, culture, family upbringing, gender, and previous pain experience influence tolerance to pain. Environmental factors, including noise, bright light, and interrupted sleep, may affect pain tolerance.
Pain expression is the way in which the pain experience is communicated to others. Pacing, writhing, jaw clenching, facial grimacing, muscle guarding, crying, moaning, groaning, and verbal descriptions may be used to express pain. Thus the highly variable nature of pain expression among individuals makes accurate pain assessment difficult.
Modulation
Modulation of pain signals occurs at multiple sites along the pain pathway. A fair amount is known about pain modulation at the spinal cord, where neurons from nociceptors, somatosensory receptors, and descending neurons from the CNS all converge and interact. Modulation also occurs at the peripheral nociceptor ending and within the brain; however, these mechanisms are less well understood.
Attempts to decrease the perception of painful stimuli may be initiated spontaneously by the person experiencing pain. Rubbing, pressing, or shaking the painful area may reduce the intensity of pain. In 1965, Melzack and Wall proposed the gate control theory to explain how stimulation of large “touch” neurons could inhibit the transmission of nociceptor impulses.7 This theory was very useful in focusing efforts to understand pain signal processing at the spinal cord level.
Central to the gate control theory is the capacity for interneurons in the spinal cord to modify the transmission of nociceptor impulses. The original gate control theory suggested that impulses carried by large myelinated cutaneous fibers (Aβ) could “close the gate” on nociceptor impulses so that pain signals would be blocked in the spinal cord and not allowed to progress centrally to the brain. The physiologic process underlying the closing-the-gate mechanisms has been the subject of much study. Numerous interneurons, neurotransmitters, and neuropeptides have been implicated in this complex gating mechanism.
Descending pathways from the brain to the dorsal horn region of the spinal cord are important modulators of the pain response (Figure 47-6). These descending pathways originate in a brainstem nucleus called the raphe magnus and project to the dorsal horn regions of laminae I, II, and IV.3 Neurotransmitters released by these neurons can inhibit synaptic transmission of pain signals. One way to inhibit synaptic transmission is through presynaptic inhibition of substance P release from nociceptor neurons (Figure 47-7). Opioids such as endorphins are thought to be the mediators of presynaptic inhibition. A similar inhibitory effect can be achieved by administering opioid drugs, such as morphine, that bind to opioid receptors and mimic the effect of endorphins.
The raphe magnus receives input from two other brain areas important in the pain response: the periaqueductal gray (PAG) area in the midbrain and the rostral pons in the brainstem. The PAG area has a high concentration of endogenous opioids (endorphins and enkephalins) that are known to produce analgesic effects similar to narcotic drugs. Stimulation of the PAG area causes release of these endogenous opioids and also sends nerve impulses to the raphe magnus. Serotonin (5-hydroxytryptamine, 5HT) is the neurotransmitter that conveys analgesic signals from the PAG area to the raphe magnus. This finding helps explain the pain-relieving action of drugs that enhance serotonin activity in the brain, such as tricyclic antidepressants.
The neurons projecting to the raphe magnus from the rostral pons secrete norepinephrine as the neurotransmitter. Stimulation of these neurons also produces an analgesic effect. Clonidine, a drug that mimics the effect of norepinephrine in the brain, has been shown to have pain-relieving properties.
The descending pathways from the brain provide an important means for gating the flow of pain impulses from the periphery to the brain. The PAG area is apprised of the flow of pain signals because it receives input by way of the thalamus and limbic structures.
Pain modulation occurs not only at the cord level but also in the brain itself. Opioids produced in the brain are thought to be important modulators of pain perception. Specific opioid receptors were identified within the brain in the early 1970s.8 Also discovered around this time were the naturally occurring morphine-like substances termed endorphins. The word endorphin is a combination of two words: endogenous (coming from within the body) and morphine (from the Latin word morpheus, meaning “sleep inducing”). The term endorphin actually refers to two groups of naturally occurring peptides: enkephalins (which are pentapeptides) and three types of endorphin polypeptides (α-, β-, and γ-endorphin). Of these, most is known about β-endorphin.
During times of stress, pain, or emotion, the brain apparently creates its own analgesia through the secretion of endogenous opioids—a process known as stress-induced analgesia. Stress-induced analgesia is reversed by naloxone, a drug that blocks opioid receptors, thus supporting the role of endogenous opioids in the process.9 As previously described, the PAG area produces large quantities of endogenous opioids that are thought to inhibit pain signal perception within the brain. High concentrations of β-endorphin are also found in the pituitary gland, and it is likely that the release of pituitary stress hormones (adrenocorticotropic hormone) is accompanied by the release of endorphins. The adrenal glands also produce endogenous opioids as a sympathetic response to stress. Endorphins released into the bloodstream by the pituitary gland and adrenal gland have their effects in the periphery because they cannot effectively cross the blood-brain barrier.
Opioids have different effects depending on the types of receptors they activate. Four types of opioid receptors have been identified: mu (μ), kappa (κ), sigma (σ), and delta (δ) (Table 47-2). The distribution of the specific opioid receptors varies throughout the body. The μ and κ receptors have analgesic activities. The μ receptors are found in high concentration in the brain, where they are thought to modulate pain perception. The κ receptors are concentrated primarily in the spinal cord, where they contribute to pain modulation by CNS descending pathways. Each opioid receptor subtype is associated with a number of undesirable side effects. Depending on the affinity for certain receptors, different drugs may have differing analgesic potency and side effect profiles (Table 47-3).
TABLE 47-2
OPIOID RECEPTOR | ACTIVITY |
Mu (μ) | |
Kappa (κ) | ![]() Stay updated, free articles. Join our Telegram channel![]() Full access? Get Clinical Tree![]() ![]() ![]() |