O2 is both essential to human life and toxic. We are dependent on O2 for oxidation reactions in the pathways of adenosine triphosphate (ATP) generation, detoxification, and biosynthesis. However, when O2 accepts single electrons, it is transformed into highly reactive oxygen radicals that damage cellular lipids, proteins, and DNA. Damage by reactive oxygen radicals contributes to cellular death and degeneration in a wide range of diseases (Table 25.1).
TABLE 25.1 Some Disease States Associated with Free-Radical Injury
Atherogenesis | Cerebrovascular disorders |
Chronic Obstructive Pulmonary Disease (COPD) | Ischemia/reperfusion injury |
Duchenne-type muscular dystrophy | Neurodegenerative disorders |
Pregnancy/pre-eclampsia | Amyotrophic lateral sclerosis (Lou Gehrig disease) |
Retrolental fibroplasia | |
Cervical cancer | Alzheimer disease |
Alcohol-induced liver disease | Down syndrome |
Hemodialysis | Ischemia-reperfusion injury following stroke |
Diabetes | OXPHOS diseases (mitochondrial DNA disorders) |
Acute renal failure | Multiple sclerosis |
Aging | Parkinson disease |
OXPHOS, oxidative phosphorylation.
Radicals are compounds that contain a single electron, usually in an outside orbital. Oxygen is a biradical, a molecule that has two unpaired electrons in separate orbitals (Fig. 25.1). Through a number of enzymatic and nonenzymatic processes that routinely occur in cells, O2 accepts single electrons to form highly reactive oxygen species (ROS). The ROS formed by the reduction of O2 are the radical superoxide (O2−), the nonradical hydrogen peroxide (H2O2), and the hydroxyl radical (OH•).
FIGURE 25.1 O2 is a biradical. It has two antibonding electrons with parallel spins (parallel arrows). It has a tendency to form toxic reactive oxygen species (ROS), such as superoxide (O2−), the nonradical hydrogen peroxide (H2O2), and the hydroxyl radical (OH•).
ROS may be generated nonenzymatically or enzymatically as accidental by-products or major products of reactions. Superoxide may be generated nonenzymatically from CoQ, or metal-containing enzymes (e.g., cytochrome P450, xanthine oxidase, and NADPH oxidase). The highly toxic hydroxyl radical is formed nonenzymatically from superoxide in the presence of Fe2+ or Cu+ by the Fenton reaction, and from hydrogen peroxide in the Haber–Weiss reaction.
Oxygen radicals and their derivatives can be deadly to cells. The hydroxyl radical causes oxidative damage to proteins and DNA. It also forms lipid peroxides and malondialdehyde from membrane lipids containing polyunsaturated fatty acids. In some cases, free-radical damage is the direct cause of a disease state (e.g., tissue damage initiated by exposure to ionizing radiation). In neurodegenerative diseases, such as Parkinson disease, or in ischemia-reperfusion injury, ROS may perpetuate the cellular damage caused by another process.
Oxygen radicals are joined in their destructive damage by the free-radical nitric oxide (NO) and the reactive oxygen species hypochlorous acid (HOCl). NO combines with O2 or superoxide to form reactive nitrogen–oxygen species (RNOS), such as the nonradical peroxynitrite or the radical nitrogen dioxide. RNOS are present in the environment (e.g., cigarette smoke) and generated in cells. During phagocytosis of invading microorganisms, cells of the immune system produce O2−, HOCl, and NO through the actions of NADPH oxidase, myeloperoxidase, and inducible nitric oxide synthase, respectively. In addition to killing phagocytosed invading microorganisms, these toxic metabolites may damage surrounding tissue components.
Cells protect themselves against damage by ROS and other radicals through repair processes, compartmentalization of free-radical production, defense enzymes, and endogenous and exogenous antioxidants (free-radical scavengers). The defense enzyme superoxide dismutase (SOD) removes the superoxide free radical. Catalase and glutathione peroxidase remove hydrogen peroxide and lipid peroxides. Vitamin E, vitamin C, and plant flavonoids act as antioxidants. Oxidative stress occurs when the rate of ROS generation exceeds the capacity of the cell for their removal (Fig. 25.2).
FIGURE 25.2 Oxidative stress. Oxidative stress occurs when the rate of ROS and RNOS production overbalances the rate of their removal by cellular defense mechanisms. These defense mechanisms include a number of enzymes and antioxidants. Antioxidants usually react nonenzymatically with ROS. RNOS, reactive oxygen species; ROS, reactive nitrogen–oxygen species.
THE WAITING ROOM 
Two years ago, Les G., a 62-year-old man, noted an increasing tremor of his right hand when sitting quietly (resting tremor). The tremor disappeared if he actively used this hand to do purposeful movement. As this symptom progressed, he also complained of stiffness in his muscles that slowed his movements (bradykinesia). His wife noticed a change in his gait; he had begun taking short, shuffling steps and leaned forward as he walked (postural imbalance). He often appeared to be staring ahead with a rather immobile facial expression. She noted a tremor of his eyelids when he was asleep and, recently, a tremor of his legs when he was at rest. Because of these progressive symptoms and some subtle personality changes (anxiety and emotional lability), she convinced Les G. to see their family doctor.
The doctor suspected that her patient probably had idiopathic parkinsonism (Parkinson disease) and referred Les G. to a neurologist. In Parkinson disease, neurons of the substantia nigra pars compacta, containing the pigment melanin and the neurotransmitter dopamine, degenerate.
Cora N. had done well since the successful lysis of blood clots in her coronary arteries with the use of intravenous recombinant tissue plasminogen activator (TPA) (see Chapters 20 and 22). This therapy quickly relieved the crushing chest pain (angina) she experienced when she won the lottery. At her first office visit after her discharge from the hospital, her cardiologist told her that in the hospital she had developed multiple premature contractions of the ventricular muscle of her heart as the clots were being lysed. This process could have led to a life-threatening arrhythmia known as ventricular tachycardia or ventricular fibrillation. However, her arrhythmia responded quickly to pharmacological suppression and did not recur during the remainder of her hospitalization.
I. O2 and the Generation of Reactive Oxygen Species
The generation of reactive oxygen species (ROS) from O2 in our cells is a natural, everyday occurrence. The electrons that contribute to their formation are usually derived from reduced electron carriers of the electron transport chain (ETC). ROS are formed as accidental products of nonenzymatic and enzymatic reactions. Occasionally, they are deliberately synthesized in enzyme-catalyzed reactions. Ultraviolet radiation and pollutants in the air can increase the formation of toxic oxygen-containing compounds.
A. The Radical Nature of O2
A radical, by definition, is a molecule that has a single unpaired electron in an orbital. A free radical is a radical that is capable of independent existence. (Radicals formed in an enzyme active site during a reaction, for example, are not considered free radicals unless they can dissociate from the protein to interact with other molecules.) Radicals are highly reactive and initiate chain reactions by extracting an electron from a neighboring molecule to complete their own orbitals. Although the transition metals (e.g., Fe, Cu, and Mo) have single electrons in orbitals, they are not usually considered free radicals because they are relatively stable, do not initiate chain reactions, and are bound to proteins in the cell.
The oxygen molecule is a biradical, which means it has two single electrons in different orbitals. These electrons cannot both travel in the same orbital because they have parallel spins (they spin in the same direction). Although oxygen is very reactive from a thermodynamic standpoint, its single electrons cannot react rapidly with the paired electrons found in the covalent bonds of organic molecules. As a consequence, O2 reacts slowly through the acceptance of single electrons in reactions that require a catalyst (such as a metal-containing enzyme).
Because the two unpaired electrons in oxygen have the same (parallel) spin, they are called antibonding electrons. In contrast, carbon–carbon and carbon–hydrogen bonds each contain two electrons, which have antiparallel spins and form a thermodynamically stable pair. As a consequence, O2 cannot readily oxidize a covalent bond because one of its electrons would have to flip its spin around to make new pairs. The difficulty in changing spins is called spin restriction. Without spin restriction, organic life forms could not have developed in the oxygen atmosphere on earth because they would be spontaneously oxidized by O2.
O2 is capable of accepting a total of four electrons, which reduces it to water (Fig. 25.3). When O2 accepts one electron, superoxide is formed. Superoxide is still a radical because it has one unpaired electron remaining. This reaction is not thermodynamically favorable and requires a moderately strong reducing agent that can donate single electrons (e.g., the radical form of coenzyme Q [CoQH•] in the electron transport chain). When superoxide accepts an electron, it is reduced to hydrogen peroxide (H2O2), which is not a radical. The hydroxyl radical is formed in the next one-electron reduction step in the reduction sequence. Finally, acceptance of the last electron reduces the hydroxyl radical to H2O.
FIGURE 25.3 Reduction of oxygen by four one-electron steps. The four one-electron reduction steps for O2 progressively generate superoxide, hydrogen peroxide, and the hydroxyl radical plus water. Superoxide is sometimes written O2− to better illustrate its single unpaired electron. H2O2, the half-reduced form of O2, has accepted two electrons and is, therefore, not an oxygen radical.
B. Characteristics of Reactive Oxygen Species
ROS are oxygen-containing compounds that are highly reactive free radicals, or compounds that are readily converted to these oxygen-free radicals in the cell. The major oxygen metabolites produced by one-electron reduction of oxygen (superoxide, hydrogen peroxide, and the hydroxyl radical) are classified as ROS (Table 25.2).
TABLE 25.2 Reactive Oxygen Species (ROS) and Reactive Nitrogen–Oxygen Species (RNOS)
REACTIVE SPECIES | PROPERTIES |
Superoxide anion (O2−) | Produced by the electron transport chain and at other sites. Cannot diffuse far from the site of origin. Generates other reactive oxygen species (ROS). |
Hydrogen peroxide (H2O2) | Not a free radical, but can generate free radicals by reaction with a transition metal (e.g., Fe2+). Can diffuse into and through cell membranes. |
Hydroxyl radical (OH•) | The most reactive species in attacking biological molecules. Produced from H2O2 in the Fenton reaction in the presence of Fe2+ or Cu+. |
Organic radicals (RO•, R•, R–S) | Organic free radicals (R denotes the remainder of the compound). Produced from ROH, RH (e.g., at the carbon of a double bond in a fatty acid), or RSH OH• attack. |
Peroxyl radical (RCOO•) | An organic peroxyl radical, such as occurs during lipid degradation (also denoted LOO•). |
Hypochlorous acid (HOCl) | Produced in neutrophils during the respiratory burst to destroy invading organisms. Toxicity is through halogenation and oxidation reactions. Attacking species is OCl−. |
Singlet oxygen (O2↓↑) | Oxygen with antiparallel spins. Produced at high oxygen tensions from absorption of UV light. Decays so fast that it is probably not a significant in vivo source of toxicity. |
Nitric oxide (NO) | Reactive nitrogen–oxygen species (RNOS). A free radical is produced endogenously by nitric oxide synthase. Binds to metal ions. Combines with O2 or other oxygen-containing radicals to produce additional RNOS. |
Peroxynitrite (ONOO−) | RNOS. A strong oxidizing agent that is not a free radical. It can generate nitrogen dioxide (NO2), which is a radical. |
Reactive free radicals extract electrons (usually as hydrogen atoms) from other compounds to complete their own orbitals, thereby initiating free-radical chain reactions. The hydroxyl radical is probably the most potent of the ROS. It initiates chain reactions that form lipid peroxides and organic radicals and adds directly to compounds. The superoxide anion is also highly reactive, but it has limited lipid solubility and cannot diffuse far. However, it can generate the more reactive hydroxyl and hydroperoxy radicals by reacting nonenzymatically with hydrogen peroxide in the Haber–Weiss reaction (Fig. 25.4).
FIGURE 25.4 Generation of the hydroxyl radical by the nonenzymatic Haber–Weiss and Fenton reactions. In the simplified versions of these reactions shown here, the transfer of single electrons generates the hydroxyl radical. ROS are shown in the boxes. In addition to Fe2+, Cu+, and many other metals can also serve as single-electron donors in the Fenton reaction. The Haber–Weiss reaction is the sum of the Fenton reaction and the regeneration of Fe2+ by superoxide, forming oxygen from the superoxide.
Hydrogen peroxide, although not actually a radical, is a weak oxidizing agent that is classified as a ROS because it can generate the hydroxyl radical (OH•). Transition metals, such as Fe2+ or Cu+, catalyze the formation of the hydroxyl radical from hydrogen peroxide in the nonenzymatic Fenton reaction (see Fig. 25.4). Because hydrogen peroxide is lipid-soluble, it can diffuse through membranes and generate OH• at localized Fe2+- or Cu+-containing sites, such as the electron transport chain within the mitochondria. Hydrogen peroxide is also the precursor of hypochlorous acid (HOCl), a powerful oxidizing agent that is produced endogenously and enzymatically by phagocytic cells. To decrease the occurrence of the Fenton reaction, accessibility to transition metals, such as Fe2+ and Cu+, are highly restricted in cells, or in the body as a whole. Events that release iron from cellular storage sites, such as a crushing injury, are associated with increased free-radical injury.
Organic radicals are generated when superoxide or the hydroxyl radical indiscriminately extract electrons from other molecules. Organic peroxy radicals are intermediates of chain reactions, such as lipid peroxidation. Other organic radicals, such as the ethoxy radical, are intermediates of enzymatic reactions that escape into solution (see Table 25.2).
An additional group of oxygen-containing radicals, termed RNOS, contains nitrogen as well as oxygen. These radicals are derived principally from the free-radical nitric oxide (NO), which is produced endogenously by the enzyme nitric oxide synthase. Nitric oxide combines with O2 or superoxide to produce additional RNOS.
C. Major Sources of Primary Reactive Oxygen Species in the Cell
ROS are constantly being formed in the cell; approximately 3% to 5% of the oxygen we consume is converted to oxygen-free radicals. Some are produced as accidental by-products of normal enzymatic reactions that escape from the active site of metal-containing enzymes during oxidation reactions. Others, such as hydrogen peroxide, are physiological products of oxidases in peroxisomes. Deliberate production of toxic free radicals occurs during the inflammatory response. Drugs, natural radiation, air pollutants, and other chemicals also can increase the formation of free radicals in cells.
1. Generation of Superoxide
One of the major sites of superoxide generation is coenzyme Q (CoQ) in the mitochondrial electron transport chain (Fig. 25.5). The one-electron reduced form of CoQ (CoQH•) is free within the membrane and can accidentally transfer an electron to dissolved O2, thereby forming superoxide. In contrast, when O2 binds to cytochrome oxidase and accepts electrons, none of the O2 radical intermediates are released from the enzyme, and no ROS are generated.
FIGURE 25.5 Generation of superoxide by coenzyme Q (CoQ) in the electron transport chain. In the process of transporting electrons to O2, some of the electrons escape when CoQH• accidentally interacts with O2 to form superoxide. Fe-H represents the Fe-heme center of the cytochromes. FMN, flavin mononucleotide; NAD, nicotinamide adenine dinucleotide.
2. Oxidases, Oxygenases, and Peroxidases
Most of the oxidases, peroxidases, and oxygenases in the cell bind O2 and transfer single electrons to it via a metal. Free-radical intermediates of these reactions may be accidentally released before the reduction is complete.
Cytochrome P450 enzymes are a major source of free radicals “leaked” from reactions. Because these enzymes catalyze reactions in which single electrons are transferred to O2 and an organic substrate, the possibility of accidentally generating and releasing free-radical intermediates is high (see Chapters 20 and 24). Induction of P450 enzymes by alcohol, drugs, or chemical toxicants leads to increased cellular injury. As an example, carbon tetrachloride (CCl4), which was used as a solvent in the dry-cleaning industry, is converted by cytochrome P450 to a highly reactive free radical that led to hepatocellular necrosis in workers in that industry, resulting in the disuse of CCl4. When the enzyme-bound CCl4 accepts an electron, it dissociates into CCl3• and Cl. The CCl3• radical, which cannot continue through the P450 reaction sequence, “leaks” from the enzyme active site and initiates chain reactions in the surrounding polyunsaturated lipids of the endoplasmic reticulum. These reactions spread into the plasma membrane and to proteins, eventually resulting in cell swelling, accumulation of lipids, and cell death. When substrates for cytochrome P450 enzymes are not present, its potential for destructive damage is diminished by repression of gene transcription.
Hydrogen peroxide and lipid peroxides are generated enzymatically as major reaction products by a number of oxidases present in peroxisomes, mitochondria, and the endoplasmic reticulum. For example, monoamine oxidase, which oxidatively degrades the neurotransmitter dopamine, generates H2O2 at the mitochondrial membrane of certain neurons. Peroxisomal fatty acid oxidase generates H2O2 rather than FAD(2H) during the oxidation of very-long-chain fatty acids (see Chapter 30). Xanthine oxidase, an enzyme of purine degradation that can reduce O2 to O2− or H2O2 in the cytosol, is thought to be a major contributor to ischemia-reperfusion injury, especially in intestinal mucosal and endothelial cells. Lipid peroxides are also formed enzymatically as intermediates in the pathways for synthesis of many eicosanoids, including leukotrienes and prostaglandins.
3. Ionizing Radiation
Cosmic rays that continuously bombard the earth, radioactive chemicals, and x-rays are forms of ionizing radiation. Ionizing radiation has a high enough energy level that it can split water into hydroxyl and hydrogen radicals, thus leading to radiation damage to the skin, mutations, cancer, and cell death. It also may generate organic radicals through direct collision with organic cellular components.
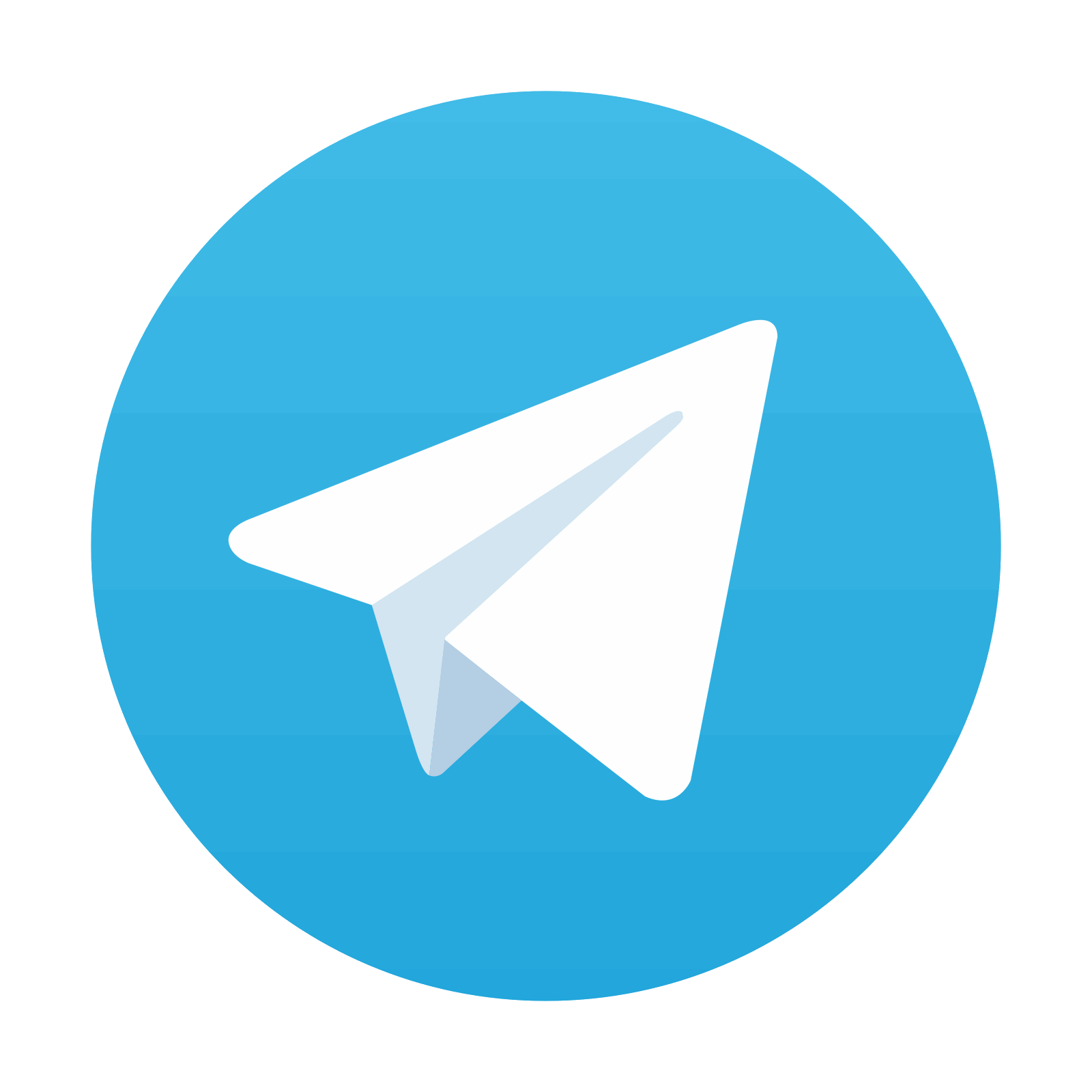
Stay updated, free articles. Join our Telegram channel

Full access? Get Clinical Tree
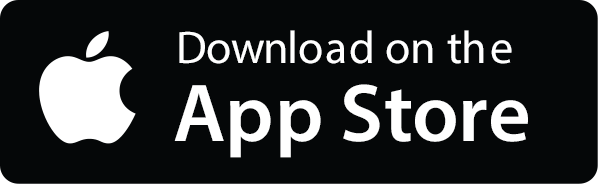
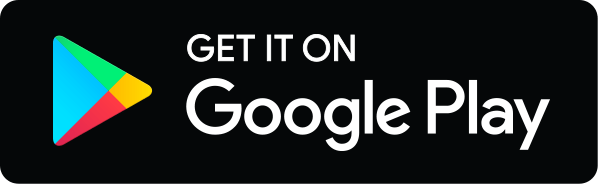