CHAPTER 12
Neuromuscular Blocking Agents
LESLY JURADO, PharmD, BCPS
TERESA A. ALLISON, PharmD, BCPS
BRIAN GULBIS, PharmD, BCPS
ELIZABETH FARRINGTON, PharmD, FCCP, FCCM, FPPAG, BCPS
DRUG OVERVIEW
Approximately 80 percent of critically ill intensive care patients require mechanical ventilation, thus administration of a one-time-only dose of a neuromuscular blocking agent (NMBA) is common. They are used to facilitate endotracheal intubation as they prevent laryngospasm and keep the patient from resisting the procedure. These agents should not be used, however, if the normalcy of the airway and the ability to successfully accomplish bag-mask ventilation and endotracheal intubation are questionable. Once intubated, only 1 percent to 15 percent of ICU patients are treated with continuous infusion or scheduled NMBAs (1%, surgical ICU; <10% medical ICU; ~15% trauma and pediatric ICU).1 Aggressive use of analgesia and sedation is essential initially, and NMBAs are reserved for patients who fail to meet desired goals despite maximum sedative therapy. The clinical practice guideline for sustained neuromuscular blockade published by the Society of Critical Care Medicine states that “NMBAs should be used in an adult patient in an ICU to manage ventilation, manage increased ICP, treat muscle spasms, and decrease oxygen consumption ONLY when all other means have been tried without success.”2
When used to manage ventilation, NMBAs allow improvement in pulmonary compliance. Neuromuscular blocking agents can assist ventilation therapy in at least three ways: (1) by reducing or eliminating spontaneous breathing; (2) preventing motor activity that might dislodge catheters, surgical dressings, or chest tubes; and (3) reducing oxygen consumption by patients with severely diminished cardiopulmonary function. However, the effect of neuromuscular blockade on ventilatory mechanics and chest wall compliance may be minimal in a patient who is maximally sedated. Although NMBAs are used in the mechanically ventilated patient, well-designed controlled trials do not exist that document improved patient outcomes when they are used to facilitate mechanical ventilation.1,2 Most reports are limited to case studies, small prospective open-label trials, and small randomized open-label and double-blind trials. In addition, none of these reports compared NMBAs to placebos.
NMBAs may be used postoperatively for several reasons. First, neuromuscular blockade can prevent unacceptably high oxygen consumption due to the profound shivering that frequently accompanies rewarming from hypothermia. This condition is particularly deleterious for hypoxic patients or those with a history of cardiovascular disease. Further, postoperative neuromuscular blockade may be a useful adjunct to promote healing of specific surgical wounds (e.g., vascular anastomosis, supraglottoplasty) by immobilizing the patient for a defined period. Immobilization may prove of particular benefit after tracheal resection and anastomosis or when closure of the wound has been difficult or disruptive and its loss of integrity would place the patient at great risk. Improved patient outcomes have been documented in the surgical population when NMBAs are utilized postoperatively for complicated ENT procedures (cricoid split and supraglottoplasty) when compared to historical controls.1
Apart from mechanical ventilation and postoperative indications, situations in the ICU that may warrant administration of NMBAs are diverse. Therapeutic paralysis has been used appropriately in treating tetanus, status epilepticus, and uncontrolled intracranial hypertension or intracranial pressure (ICP). The use of NMBAs for prevention of rhabdomyolysis, myoglobinuria, and acute renal failure following status epilepticus and tetanus leads to improved patient outcomes. These benefits are intuitive and not extensively documented in the literature (mostly case reports). However, as paralytics do nothing to terminate seizures or protect the brain of seizing patients, concomitant antiepileptic therapy is mandatory and continuous or intermittent EEG monitoring is recommended. The routine use of NMBAs for patients post head injury has been discouraged, due to increased risk of pneumonia that may result in prolongation of ICU stay. Therapeutic paralysis may help control elevated intracranial pressure, so NMBAs may have a role after more conventional therapies post head injury.
The main use of NMBAs outside of the ED and ICU settings is to produce skeletal muscle relaxation during surgery after general anesthesia has been induced. When used in this setting, NMBAs allow a lighter level of anesthesia to be used.
PHYSIOLOGY
Although a detailed review of the physiology of the neuromuscular junction cannot be presented here, an understanding of the basic physiology is necessary for discussion of the pharmacodynamics of the neuromuscular blocking agent (NMBA). The neuromuscular junction consists of the prejunctional motor nerve ending, synaptic cleft, and postjunctional membrane, which contain nicotinic cholinergic receptors. The neurotransmitter acetylcholine (ACh) is synthesized in the motor nerve terminal and stored in vesicles. Normal neuromuscular transmission results from the release of ACh from the nerve terminal, its movement across the synaptic cleft, and subsequent binding to the postsynaptic nicotinic receptor on the sarcolemma of the skeletal muscle. The ACh molecules diffuse across the synaptic cleft and bind to the acetylcholine receptors, initiating a conformational change in the receptor that “opens” a potential channel formed by the receptor subunits. The opening of this channel allows the influx of sodium and calcium ions and the efflux of potassium ions, thereby facilitating the depolarization of the motor endplate and propagation of an action potential that spreads across the skeletal muscle fibers, leading to contraction. The enzyme acetylcholinesterase is responsible for rapid hydrolysis of ACh, which terminates the depolarization of the motor endplate.3
Neuromuscular blocking agents are designed to structurally resemble acetylcholine and all currently available NMBAs induce paralysis of skeletal muscle by occupying the ACh receptors on the muscle fiber, thereby preventing the binding of ACh to the receptors. They are classified as either depolarizing or nondepolarizing relaxants according to their effect on the motor end plate. The bulky nature of nondepolarizing NMBA, compared with that of ACh, causes drugs to interact with the receptors as antagonists, rather than agonists. Succinylcholine, the only depolarizing NMBA in clinical use, has a high affinity for ACh receptor sites. It binds with ACh receptors and depolarizes the motor end plate, but produces a more sustained depolarization than ACh, inactivating sodium channels and preventing impulse transmission. Transient twitching of skeletal muscle (fasciculation) is briefly produced, followed by paralysis. By contrast, nondepolarizing agents compete with ACh for access to receptors on the motor end plate, but once bound have no agonist activity. They have no effect on the resting electric potential of the motor end plate and do not cause muscle contraction. Evidence suggests that they also act to prevent ACh mobilization to some degree.3
SEQUENCE OF ONSET OF NEUROMUSCULAR BLOCKADE
Small, rapidly moving muscles such as those of the eyes and digits are affected by NMBA before those of the trunk and abdomen. Ultimately, intercostal muscles and finally the diaphragm are paralyzed. Recovery of skeletal muscles usually occurs in the reverse order to that of paralysis such that the diaphragm is the first to regain function.
Intravenous injection of an NMBA to a person who is awake initially produces difficulty in focusing and weakness in the mandibular muscles followed by ptosis, diplopia, and dysphagia. Relaxation of the small muscles of the ears improves acuity of hearing. Consciousness and sensorium remain undisturbed even in the presence of complete neuromuscular blockade. If time and patient condition permit, counseling the patient of what he or she will experience would most likely decrease any need for anxiolytics and sedation as the experience would be less terrifying.
BIOAVAILABILITY
All NMBA are poorly absorbed from the gastrointestinal tract. The onset of action varies between individual agents (Table 12-1), the route of administration, dosage, and concomitant drug therapy. In general, the first signs of neuromuscular blockade occur within 2 minutes following the IV administration of the nondepolarizing NMBA, and maximal effects occur in approximately 3–6 minutes. The maximal effects of succinylcholine occur within 1 minute. The onset of action following IM administration is slower and less predictable than following IV administration; therefore, the IM route is reserved for patients with no IV access.
TABLE 12-1 | Summary of Available Neuromuscular Blocking Drugs |
MD, maintenance dose; CI, continuous infurion; ano longer manufactured in the United States.
Several blockers have been studied after intramuscular administration. The bioavailability of rapacuronium is 56 percent, and peak plasma concentrations occur 4–5 minutes after administration after 2.8 or 4.8 mg/kg during halothane anesthesia.4 Rocuronium has better qualities than other nondepolarizers administered intramuscularly in that its bioavailability is greater than 80 percent, and less than 5 percent of the drug remains in muscle 30 minutes after administration.5 Optimal intubating conditions exist 3 minutes after 1–1.8 mg/kg.5 Succinylcholine may also be administered IM with intubating conditions in 2–3 minutes.6
VOLUME OF DISTRIBUTION (VD)/DISTRIBUTION
A summary of individual Vd can be found in Table 12-2. Following IV administration, the drugs are distributed into the extracellular fluid and rapidly reach their site of action at the motor end plate. Conditions associated with an increase in extracellular fluid volume may require a higher dose of NMBA. These patients include those who have congestive heart failure, patients with peritonitis, and patients immediately postpartum.7 In addition, newborn infants are known to have larger extracellular fluid volumes per unit of body weight. For all neuromuscular blockers that have been studied, the volume of distribution is greater in infants.8 Most NMBA cross the blood-brain barrier to a small extent, if at all. Increased protein binding (possibly to alpha-1 acid glycoprotein) of nondepolarizing NMBA with a resulting decrease in free fraction of circulating drug has been reported in patients with burns.6 Other conditions associated with an increase in alpha-1 acid glycoprotein are acute myocardial infarction, cancer, inflammatory diseases (Crohn’s and inflammatory arthritis), surgery, trauma injury, and administration of phenytoin and carbamazepine.9-11
TABLE 12-2 | Pharmacokinetics of Neuromuscular Blocking Agents |
t1/2, half life; ano longer available in the United States
ELIMINATION
A summary of the method of elimination and clearance of individual NMBAs can be found in Table 12-2. Succinylcholine is metabolized rapidly by pseudocholinesterase and is excreted in the urine as active and inactive metabolites and small amounts of unchanged drug.5 Pancuronium and pipecuronium are excreted primarily unchanged in the urine. Following IV administration, atracurium besylate and cisatracurium undergo rapid metabolism via Hoffman elimination and via nonspecific enzymatic ester hydrolysis. Atracurium besylate and cisatracurium and their metabolites, including metabolic products of Hoffman elimination and ester hydrolysis are excreted primarily in the urine and also in feces via biliary elimination. Only a small fraction of the dose is excreted unchanged in the urine and bile. Vecuronium and rocuronium have both renal and biliary elimination; therefore, caution should be used when administering a continuous infusion to patients with either renal or hepatic dysfunction. Careful train-of-four monitoring is essential.
For NMBAs that are eliminated by renal elimination or hepatic metabolism, drug clearance is not proportional to the volume of distribution. A longer half-life, therefore, is observed in infants and children or any patient with renal or hepatic dysfunction. If the drug is metabolized in body fluids, however, as is the case for succinylcholine, mivacurium, atracurium, and cisatracurium; then, increasing the Vd results in increased clearance.8
PATIENT POPULATIONS WITH ALTERED PHARMACOKINETICS AND/OR PHARMACODYNAMICS
BURN PATIENTS
Patients with burn injury are resistant to the action of nondepolarizing NMBA. The magnitude of resistance depends on the extent of thermal injury and elapsed time since the burn, with patients having burns that extend over 25–30 percent or more of body surface area being most likely to exhibit resistance (increasing with increased injury) and the resistance only becoming apparent one week or longer after the burn.12 NMBA resistance has been reported to peak two or more weeks after the burn, persists for several months or longer, and then decreases gradually with healing.12 The mechanism of this resistance appears to be multifactorial and may involve pharmacokinetic, pharmacodynamic, and pathophysiologic factors. Increased production of alpha-1 acid glycoprotein will reduce the free (unbound) fraction of circulating NMBAs and may contribute to this resistance, however, the magnitude of the resistance cannot be solely explained by this mechanism. It also has been suggested that changes in the number of acetylcholine receptors and/or in anticholinesterase activity may contribute to this NMBA resistance. Other mechanisms (e.g., circulating substances in plasma that bind to or inactivate the drugs) also have been suggested. Higher and/or more frequent doses are required in patients with burn injury, especially when the injury is ≥30 percent.
OBESITY
For the majority of NMBAs, total body weight (TBW) dosing will result in a prolonged duration of effect in morbidly obese patients when compared with nonobese patients.
Succinylcholine
In morbidly obese patients, the concentration of pseudocholinesterase, the enzyme that metabolizes succinylcholine, is increased.13 Because the level of plasma pseudocholinesterase activity and the volume of extracellular fluid determine the duration of action of succinylcholine, and both of these factors are increased in obesity, morbidly obese patients have larger absolute succinylcholine dose requirements than average-weight patients. When succinylcholine administration is based upon TBW, rather than upon lean body weight (LBW) or ideal body weight (IBW), a more profound neuromuscular block and better intubating conditions are achieved.14
Rocuronium
Nondepolarizing muscle relaxants such as rocuronium are only weakly or moderately lipophilic because the quaternary ammonium group they contain makes these molecules, as a whole, highly ionized at physiologic pH. The poor lipophilicity limits distribution outside the extracellular fluid space. However, the effect of the increased extracellular fluid volume is poorly understood. In one study,15 after administration of 0.6 mg/kg of rocuronium, the pharmacokinetic parameters and spontaneous recovery to 75 percent of twitch height were similar in obese and lean patients. When administered to morbidly obese patients on the basis of both TBW and IBW, the duration of action was more than double when rocuronium was dosed on TBW.16 Although higher doses of rocuronium result in a prolonged duration of action, no difference in onset time is observed when 0.6 mg/kg rocuronium is administered based on IBW, IBW and 20 percent of excess weight, or IBW and 40 percent of excess weight.17 Therefore, the recommendation is to base rocuronium administration in morbidly obese patients on IBW. Similar results are reported for pancuronium.17
Vecuronium
Seven obese patients receiving TBW-based 0.1 mg/kg vecuronium took 60 percent longer to recover from neuromuscular blockade than did seven normal-weight controls.18 However, pharmacokinetic parameters uncorrected for weight were similar between the two groups; therefore, basing administration on IBW is recommended.
Cisatracurium
Because cisatracurium is eliminated via Hoffman elimination, investigators have suggested it as the NMBA of choice for obese patients. However, when administered to both morbidly obese and normal-weight patients on the basis of both TBW and IBW, the duration of action was prolonged in morbidly obese patients.19 When cisatracurium was administered to both obese patients and normal-weight patients according to IBW, its duration of action was shorter in the morbidly obese patient.20
In conclusion, succinylcholine should be dosed based on TBW. TBW dosing of nondepolarizing neuromuscular blockers will result in overdosing, therefore, IBW is recommended for these agents. If a nondepolarizing agent is needed, shorter-acting agents such as rocuronium or cisatracurium are recommended.
HYPOTHERMIA
During hypothermia, redistribution of blood away from the extremities, gastrointestinal tract, kidneys, and liver toward the coronary and cerebral circulation takes place. Vasodilation of skeletal muscles contributes to this redistribution. The intravascular distribution volume is reported to be decreased by 10–35 percent in animal models.21 The Vd of pancuronium was reported to decrease by 40 percent in patients with moderate-to-severe hypothermia. The reduction in extracellular volume in addition to the reduced renal and hepatic blood flow and biliary clearance indicated that smaller doses may be required, along with less frequent dosing. Intermittent dosing as needed would be a more practical approach than continuous infusion with train-of-four monitoring.
Choice of Agents
NMBA may also be classified according to the duration of blockade they produce: short, intermediate, or long (Table 12-1). The selection of an NMBA must be based on the needs of the patient. Four variables that must be considered are time of onset, duration of action, side effects, and route of elimination for the agent chosen. Other equally important factors, often overlooked by physicians caring for the patient, are intravenous access, drug-drug compatibility, and volume of intravenous fluid required to administer a continuous infusion. One may choose a longer-acting agent dosed as needed over a continuous infusion in a severely fluid restricted patient. Lastly, one should not overlook cost when choosing an NMBA.
The medical condition of the patient also influences the NMBA decision. Patients with cardiovascular impairment are of special concern, because some NMBA produce cardiovascular effects such as hypotension and arrhythmias. Cardiovascular-stable NMBAs include vecuronium, pipecuronium, cisatracurium, and rocuronium. Pancuronium should be avoided in patients with preexisting tachycardia who cannot tolerate a further increase in the heart rate (angina, tachyarrhythmia). However, many young patients without preexisting cardiovascular disease can tolerate the increase in heart rate. Presence of hepatic and/or renal failure must be taken into consideration when choosing an NMBA, but is not a contraindication to agents metabolized and eliminated by these routes as long as appropriate monitoring is performed. In fact, use of these agents in concert with train-of-four (TOF) monitoring or dosing with movement may be used in an effort to reduce the overall costs of NMBA therapy. Atracurium and cisatracurium are often used in multisystem organ failure because their metabolism is via Hoffman elimination and ester hydrolysis, which is independent of the hepatic metabolism and renal elimination. Histamine release by some NMBAs (d-tubocurarine, atracurium) can place an asthmatic patient at increased risk. Pancuronium, vecuronium, pipecuronium, rocuronium, or cisatracurium is not associated with significant histamine release and may be preferred for asthmatics. Patients with extensive burns may also require dosage adjustments, because they may have increased synthesis of extrajunctional cholinergic receptors and thus react unpredictably to NMBAs. The accumulation of extrajunctional ACh receptors may be responsible for the risk of severe hyperkalemia that can occur following the use of succinylcholine in patients with burns, stroke, polio, spinal cord injury, severe muscle trauma, enforced immobilization, Guillain-Barré syndrome, or other conditions producing loss of nerve function. Succinylcholine should never be used in any of these situations.
The consensus statement of the Society for Critical Care Medicine (SCCM) states that the majority of patients in the ICU who are prescribed an NMBA can be managed effectively with pancuronium.2 For patients for whom vagolysis is contraindicated (e.g., those with cardiovascular disease), NMBAs other than pancuronium may be used.2 Many practitioners prefer vecuronium for those patients with cardiac disease or hemodynamic instability in whom tachycardia may be deleterious, based on the drug’s cardiovascular stability, the low cost of the drug, and many years of experience in clinical practice. Lastly, because of their unique metabolism, cisatracurium or atracurium is recommended for patients with significant hepatic or renal disease.2
INTUBATION
Choice of appropriate NMBA for intubation is not always straightforward, especially when complicated by the need for tracheal intubation without bag and mask ventilation as in the case of a patient with a full stomach. Succinylcholine historically has been the gold standard for a rapid-sequence intubation because of the onset of 90 percent neuromuscular blockade within 60 seconds. However, large doses of some nondepolarizing agents such as mivacurium and rocuronium approach this onset: 2–2.5 minutes and 1–1.5 minutes, respectively. However, mivacurium is no longer commercially available in the United States. If practicing in an area where mivacurium is available, one should be aware that rapid bolus doses of mivacurium may cause some histamine release and hypotension in patients with preexisting cardiovascular instability.
The remainder of the nondepolarizing NMBAs generally take 3–4 minutes to reach intubating conditions. However, if succinylcholine is contraindicated and mivacurium or rocuronium are unavailable, two techniques can hasten the onset of neuromuscular blockade with nondepolarizing agents. One technique is priming. It involves the administration of one-tenth of an intubating dose of a nondepolarizing NMBA, followed 4 minutes later by an intubating dose. Then, after waiting an additional 90 seconds, intubation of the trachea may be performed.3 The inherent risks of this method are related to the degree of weakness or respiratory distress in the patient before priming and to the fear and anxiety produced by the diplopia and dyspnea that often follow the priming dose. Informing the patient of what to expect can be extremely helpful in decreasing the anxiety and fear.
The second technique involves giving a relative overdose of the NMBA to flood the receptors, thereby shortening the time of onset. The usual practice is to administer two times the intubating dose of an NMBA as a rapid bolus. The complications of this technique are related to the cardiovascular effects of the relaxant, which can be avoided by the use of a drug with stable cardiovascular profile such as vecuronium, doxacurium (not available in the U.S.), cisatracurium, rapacuronium, or rocuronium.
MONITORING WITH CONTINUOUS INFUSION
It is now widely recommended that continuous NMBA be monitored using either a train-of-four (TOF) or a double-burst muscle twitch response to peripheral nerve stimulation (PNS). Monitoring by this method may prevent prolonged effect of the NMBA due to (1) changing organ function, (2) addition of medications that potentiate NMBA, or (3) accumulation of the drug or metabolite. In addition, investigators have documented that adjusting the dose of NMBA by PNS versus standard clinical dosing in critically ill patients reduces the drug requirements and results in cost savings.1
Nerve stimulators deliver an electrical current that is intended to activate a motor nerve, while the mechanical response of a muscle enervated by that nerve is measured. As the NMBA occupies an increasing number of the postsynaptic ACh receptors, the block becomes more profound and the muscle response to nerve stimulation diminishes. One must be careful to avoid direct muscle stimulation with the nerve stimulator as muscle will contract if stimulated electrically, regardless of the degree of block of the neuromuscular junction. This false positive result would cause the clinician to increase the dose of NMBA that may lead to accumulation and prolonged paralysis after discontinuation of the NMBA.
Monitoring of neuromuscular function is uncomfortable and can be painful if tetanic stimulation is used. Therefore, monitoring should begin after sedation and analgesia, and optimally before any NMBA is given. The latter is not always possible. This sequence will assure that the nerve stimulator is functioning properly and the electrodes are placed correctly to assess the patient’s baseline strength of response. The electrical current is delivered via surface electrodes (ECG electrodes are most commonly used), which should be placed over skin that is clean, dry, and hairless. Electrodes should be replaced every 24 hours as the conductive gel dries out. Substantial edema or obesity may result in insufficient current being delivered to the nerve by surface electrodes. Needle electrodes (23G) are available if ECG electrodes are ineffective.
Theoretically, any accessible nerve may be stimulated to assess neuromuscular blockade. However, stimulating the ulnar nerve while measuring the effect at the adductor pollicis has become the standard. If the ulnar nerve is not available or easily accessible, the facial nerve can be used, and the response at the orbicularis occuli can be observed. Lastly, the posterior tibial nerve can be stimulated behind the medial malleus and plantar flexion of the great toe can be observed, or the peroneal nerve can be stimulated around the fibular head, and dorsiflexion of the foot can be recorded. The evoked responses can be uncomfortable and, therefore, are not always practical in patients who are conscious, but most patients will be receiving analgesics and sedatives.
Train-of-Four
Train-of-four (TOF) is used most commonly in the ICU to monitor NMBA. This approach uses a train or series of four stimuli at a frequency of 2 Hz for 2 seconds. In the absence of NMBA, four twitches of equal amplitude should be observed. In the presence of a nondepolarizing NMBA, a progressive decrease in amplitude of each successive twitch is seen. Formally, the measured response is reported as the ratio of the amplitude of the fourth twitch to the first twitch, as measured with a force transducer, yielding the TOF ratio. However, it is not practical to measure the amplitude of twitches with a transducer in the clinical setting, therefore, the number of stimuli-induced palpable twitches is recorded. Because of the wide margin of safety of neuromuscular transmission, a single twitch is not abolished until 75 percent blockade is achieved (ratio 3/4; three twitches present out of four). Two palpable twitches correlate with approximately 80 percent suppression, one palpable twitch with approximately 90 percent suppression, and no twitches correlates with 100 percent or greater twitch suppression.
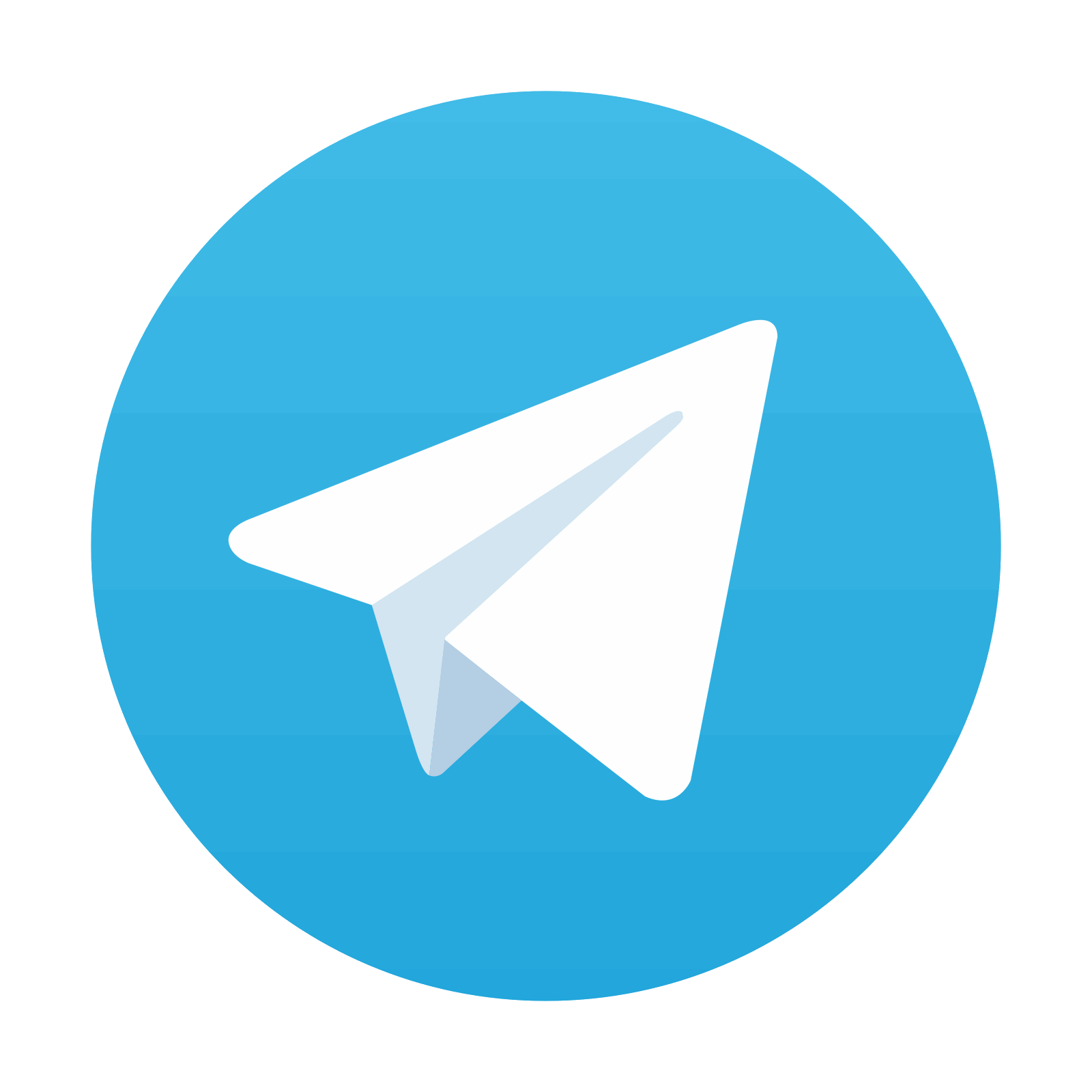
Stay updated, free articles. Join our Telegram channel

Full access? Get Clinical Tree
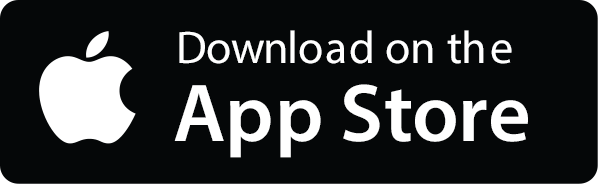
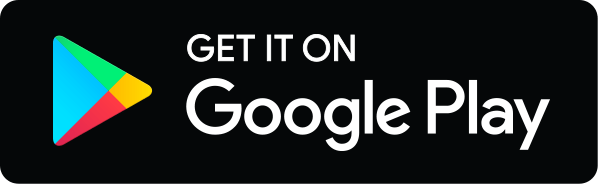